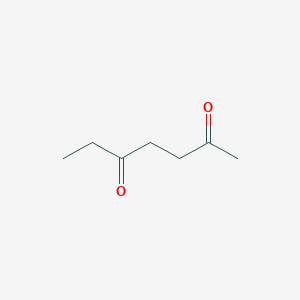
2,5-Heptandion
Übersicht
Beschreibung
Heptan-2,5-dione is a ketone.
2,5-Heptanedione is a natural product found in Streptomyces with data available.
Wissenschaftliche Forschungsanwendungen
Metallchelatisierung für MOCVD
2,5-Heptandion dient als Metallchelatisierungsmittel bei der Herstellung von Metalldiketonaten für die Metallorganische chemische Gasphasenabscheidung (MOCVD), ein Verfahren zur Abscheidung dünner Schichten aus der Gasphase .
Elektronische Transporteigenschaften
Die Forschung hat die elektronischen Transporteigenschaften von β-Diketonen wie this compound untersucht, wenn sie als molekulare Drähte in elektronischen Geräten verwendet werden. Diese Studien konzentrieren sich auf die Umwandlung zwischen Enol- und Ketoformen, die durch Wasserstoffübertragung induziert wird .
Synthese und Charakterisierung von Komplexen
Komplexe mit this compound-Derivaten wurden synthetisiert und hinsichtlich ihrer thermischen Eigenschaften und potenziellen Anwendungen in der Materialwissenschaft charakterisiert .
Optoelektronische Eigenschaften
Europiumkomplexe mit this compound-Derivaten wurden hergestellt und hinsichtlich verschiedener optoelektronischer Eigenschaften charakterisiert, die für Anwendungen in Leuchtdioden (LEDs) und anderen Anzeigetechnologien entscheidend sind .
Wirkmechanismus
Target of Action
The primary target of 2,5-Heptanedione is the lysine residues in axonal proteins . These proteins play a crucial role in the structure and function of neurons, particularly in the peripheral nervous system .
Mode of Action
2,5-Heptanedione interacts with its targets through a two-step process. First, it reacts with lysine residues in axonal proteins by Schiff base formation . This reaction is followed by cyclization to give pyrroles . The formation of these pyrroles is a key step in the compound’s mode of action .
Biochemical Pathways
The biochemical pathway affected by 2,5-Heptanedione involves the oxidation of the pyrrole residues . This oxidation causes cross-linking and denaturation of proteins, which perturbs axonal transport and function . The disruption of these pathways leads to damage to nerve cells .
Pharmacokinetics
The pharmacokinetics of 2,5-Heptanedione involves its absorption, distribution, metabolism, and excretion (ADME). In both rats and humans, the inhalation kinetics of n-hexane and n-heptane were compared with the urinary excretion of 2,5-hexanedione . The reactivities of 2,5-hexanedione and 2,5-heptanedione with Nα-acetyl-L-lysine towards the formation of pyrrolyc adducts were also studied .
Result of Action
The result of 2,5-Heptanedione’s action is the damage to nerve cells . This damage is associated with a degeneration of the peripheral nervous system, and eventually the central nervous system . Symptoms of this damage can include tingling and cramps in the arms and legs, followed by general muscular weakness . In severe cases, atrophy of the skeletal muscles is observed, along with a loss of coordination and vision problems .
Safety and Hazards
2,5-Heptanedione is considered hazardous. It is classified as a flammable liquid, skin corrosive/irritant, serious eye damage/eye irritant, and specific target organ toxicant (single exposure). The target organs are the respiratory system . Personal precautions, protective equipment, and emergency procedures are recommended when handling 2,5-Heptanedione .
Zukünftige Richtungen
Future directions for the study of 2,5-Heptanedione could include further exploration of its synthesis, chemical reactions, and mechanism of action. There is also a need for more detailed analysis of its physical and chemical properties, as well as its safety and hazards .
Relevant papers on 2,5-Heptanedione have been analyzed to provide this comprehensive overview .
Biochemische Analyse
Biochemical Properties
It is known that its structural analog, 2,5-Hexanedione, reacts with lysine residues in axonal proteins by Schiff base formation followed by cyclization to give pyrroles . This suggests that 2,5-Heptanedione may have similar interactions with enzymes, proteins, and other biomolecules.
Cellular Effects
Studies on 2,5-Hexanedione have shown that it can cause neurotoxicity . It is possible that 2,5-Heptanedione may have similar effects on various types of cells and cellular processes.
Molecular Mechanism
It is known that 2,5-Hexanedione, a structurally similar compound, reacts with lysine residues in axonal proteins by Schiff base formation followed by cyclization to give pyrroles . Oxidation of the pyrrole residues then causes cross-linking and denaturation of proteins, which perturbs axonal transport and function and causes damage to nerve cells .
Temporal Effects in Laboratory Settings
It is known that its structural analog, 2,5-Hexanedione, can cause neurotoxicity , suggesting that 2,5-Heptanedione may have similar long-term effects on cellular function.
Dosage Effects in Animal Models
It is known that its structural analog, 2,5-Hexanedione, can cause neurotoxicity , suggesting that 2,5-Heptanedione may have similar dosage-dependent effects.
Metabolic Pathways
It is known that its structural analog, 2,5-Hexanedione, is a major metabolite of the neurotoxic industrial solvent methyl n-butyl ketone .
Transport and Distribution
It is known that its structural analog, 2,5-Hexanedione, can cause neurotoxicity , suggesting that 2,5-Heptanedione may have similar effects on its localization or accumulation.
Subcellular Localization
It is known that its structural analog, 2,5-Hexanedione, can cause neurotoxicity , suggesting that 2,5-Heptanedione may have similar effects on its activity or function.
Eigenschaften
IUPAC Name |
heptane-2,5-dione | |
---|---|---|
Source | PubChem | |
URL | https://pubchem.ncbi.nlm.nih.gov | |
Description | Data deposited in or computed by PubChem | |
InChI |
InChI=1S/C7H12O2/c1-3-7(9)5-4-6(2)8/h3-5H2,1-2H3 | |
Source | PubChem | |
URL | https://pubchem.ncbi.nlm.nih.gov | |
Description | Data deposited in or computed by PubChem | |
InChI Key |
HGRGPAAXHOTBAM-UHFFFAOYSA-N | |
Source | PubChem | |
URL | https://pubchem.ncbi.nlm.nih.gov | |
Description | Data deposited in or computed by PubChem | |
Canonical SMILES |
CCC(=O)CCC(=O)C | |
Source | PubChem | |
URL | https://pubchem.ncbi.nlm.nih.gov | |
Description | Data deposited in or computed by PubChem | |
Molecular Formula |
C7H12O2 | |
Source | PubChem | |
URL | https://pubchem.ncbi.nlm.nih.gov | |
Description | Data deposited in or computed by PubChem | |
DSSTOX Substance ID |
DTXSID7073272 | |
Record name | 2,5-Heptanedione | |
Source | EPA DSSTox | |
URL | https://comptox.epa.gov/dashboard/DTXSID7073272 | |
Description | DSSTox provides a high quality public chemistry resource for supporting improved predictive toxicology. | |
Molecular Weight |
128.17 g/mol | |
Source | PubChem | |
URL | https://pubchem.ncbi.nlm.nih.gov | |
Description | Data deposited in or computed by PubChem | |
CAS No. |
1703-51-1 | |
Record name | 2,5-Heptanedione | |
Source | ChemIDplus | |
URL | https://pubchem.ncbi.nlm.nih.gov/substance/?source=chemidplus&sourceid=0001703511 | |
Description | ChemIDplus is a free, web search system that provides access to the structure and nomenclature authority files used for the identification of chemical substances cited in National Library of Medicine (NLM) databases, including the TOXNET system. | |
Record name | 2,5-Heptanedione | |
Source | EPA DSSTox | |
URL | https://comptox.epa.gov/dashboard/DTXSID7073272 | |
Description | DSSTox provides a high quality public chemistry resource for supporting improved predictive toxicology. | |
Record name | 2,5-HEPTANEDIONE | |
Source | FDA Global Substance Registration System (GSRS) | |
URL | https://gsrs.ncats.nih.gov/ginas/app/beta/substances/UP8A88O1MS | |
Description | The FDA Global Substance Registration System (GSRS) enables the efficient and accurate exchange of information on what substances are in regulated products. Instead of relying on names, which vary across regulatory domains, countries, and regions, the GSRS knowledge base makes it possible for substances to be defined by standardized, scientific descriptions. | |
Explanation | Unless otherwise noted, the contents of the FDA website (www.fda.gov), both text and graphics, are not copyrighted. They are in the public domain and may be republished, reprinted and otherwise used freely by anyone without the need to obtain permission from FDA. Credit to the U.S. Food and Drug Administration as the source is appreciated but not required. | |
Retrosynthesis Analysis
AI-Powered Synthesis Planning: Our tool employs the Template_relevance Pistachio, Template_relevance Bkms_metabolic, Template_relevance Pistachio_ringbreaker, Template_relevance Reaxys, Template_relevance Reaxys_biocatalysis model, leveraging a vast database of chemical reactions to predict feasible synthetic routes.
One-Step Synthesis Focus: Specifically designed for one-step synthesis, it provides concise and direct routes for your target compounds, streamlining the synthesis process.
Accurate Predictions: Utilizing the extensive PISTACHIO, BKMS_METABOLIC, PISTACHIO_RINGBREAKER, REAXYS, REAXYS_BIOCATALYSIS database, our tool offers high-accuracy predictions, reflecting the latest in chemical research and data.
Strategy Settings
Precursor scoring | Relevance Heuristic |
---|---|
Min. plausibility | 0.01 |
Model | Template_relevance |
Template Set | Pistachio/Bkms_metabolic/Pistachio_ringbreaker/Reaxys/Reaxys_biocatalysis |
Top-N result to add to graph | 6 |
Feasible Synthetic Routes
Haftungsausschluss und Informationen zu In-Vitro-Forschungsprodukten
Bitte beachten Sie, dass alle Artikel und Produktinformationen, die auf BenchChem präsentiert werden, ausschließlich zu Informationszwecken bestimmt sind. Die auf BenchChem zum Kauf angebotenen Produkte sind speziell für In-vitro-Studien konzipiert, die außerhalb lebender Organismen durchgeführt werden. In-vitro-Studien, abgeleitet von dem lateinischen Begriff "in Glas", beinhalten Experimente, die in kontrollierten Laborumgebungen unter Verwendung von Zellen oder Geweben durchgeführt werden. Es ist wichtig zu beachten, dass diese Produkte nicht als Arzneimittel oder Medikamente eingestuft sind und keine Zulassung der FDA für die Vorbeugung, Behandlung oder Heilung von medizinischen Zuständen, Beschwerden oder Krankheiten erhalten haben. Wir müssen betonen, dass jede Form der körperlichen Einführung dieser Produkte in Menschen oder Tiere gesetzlich strikt untersagt ist. Es ist unerlässlich, sich an diese Richtlinien zu halten, um die Einhaltung rechtlicher und ethischer Standards in Forschung und Experiment zu gewährleisten.