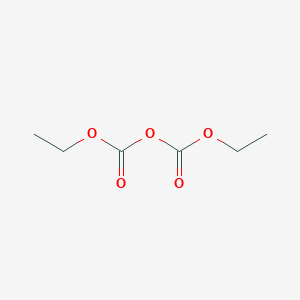
Diethyl pyrocarbonate
Übersicht
Beschreibung
Diethyl pyrocarbonate (DEPC) is the diethyl ester of dicarbonic acid . It is functionally related to a dicarbonic acid and is used as a preservative for wines, soft drinks, and fruit juices . It is also a gentle esterifying agent . DEPC is sensitive to moisture and pH and decomposes at 155°C, to ethanol and carbon dioxide in aqueous solution . It is also sensitive to ammonia, which causes decomposition to urethane, a possible carcinogen .
Synthesis Analysis
DEPC is used in the laboratory to inactivate RNase enzymes in water and on laboratory utensils . It does so by the covalent modification of histidine (most strongly), lysine, cysteine, and tyrosine residues . All the solutions used in the RNA extraction procedure were prepared with autoclaved 0.1% DEPC (diethyl pyrocarbonate) treated MilliQ water .Molecular Structure Analysis
The molecular formula of DEPC is C6H10O5 . The molecular weight is 162.14 g/mol . The structure is also available as a 2d Mol file or as a computed 3d SD file .Chemical Reactions Analysis
DEPC reacts with the catalytic amino acid in the reactive center of the RNAse molecule, inhibiting the enzyme . It reacts most strongly with histidine residues, but can also react with lysine, cysteine, and tyrosine residues . DEPC is unstable in water and susceptible to hydrolysis to carbon dioxide and ethanol, especially in the presence of a nucleophile . For this reason, DEPC cannot be used with Tris or HEPES buffers .Physical And Chemical Properties Analysis
DEPC is a clear, colorless liquid . Its density is 1.101 g/mL at 25 °C . It is soluble in alcohols, esters, ketones, and other hydrocarbons, but has limited solubility in water .Wissenschaftliche Forschungsanwendungen
Protein Modification
Diethyl pyrocarbonate (DEPC) is commonly used for the modification of histidyl residues in proteins. This application is crucial in studying protein structure and function, as it allows researchers to investigate the role of specific amino acids in enzymatic activities or protein interactions .
Nuclease Inhibition
DEPC serves as a nuclease inhibitor, which is essential for extracting undegraded nucleic acids from plant tissues. This application is particularly important in molecular biology research where intact RNA is required for experiments such as gene expression analysis .
DNA Modification
Researchers utilize DEPC for the modification of linear and supercoiled plasmid DNAs. This application aids in understanding DNA-protein interactions and can be used in various genetic engineering studies .
PCR Water Treatment
In PCR reactions, DEPC-treated deionized water is used to reduce the risk of RNA degradation by RNases. This ensures that the RNA remains intact throughout the amplification process, which is critical for accurate results .
Dot Blot Hybridization
DEPC is also employed in dot blot hybridization techniques to dilute total RNA isolated from different microorganisms. This application is vital for detecting specific RNA sequences and studying microbial gene expression .
RNase Inactivation
In laboratory settings, DEPC is used to inactivate RNase enzymes in water and on lab utensils. By covalently modifying histidine, lysine, cysteine, and tyrosine residues, DEPC prevents RNase activity, which is crucial for working with RNA without degradation .
Wirkmechanismus
Target of Action
Diethyl pyrocarbonate (DEPC) primarily targets RNase enzymes . These enzymes are responsible for the degradation of RNA, a crucial molecule involved in various biological functions such as coding, decoding, regulation, and expression of genes .
Mode of Action
DEPC interacts with its targets through covalent modification of certain amino acid residues. It most strongly modifies histidine residues , but can also react with lysine, cysteine, and tyrosine residues . This modification inhibits the activity of RNase enzymes, preventing them from degrading RNA .
Biochemical Pathways
The primary biochemical pathway affected by DEPC is the RNA degradation pathway . By inhibiting RNase enzymes, DEPC prevents the breakdown of RNA, thereby preserving its integrity for further biological processes . This is particularly important in laboratory settings where RNA degradation can compromise experimental results .
Pharmacokinetics
DEPC is unstable in water and susceptible to hydrolysis, decomposing into carbon dioxide and ethanol . This property impacts its bioavailability, as it must be carefully handled and stored to prevent premature decomposition .
Result of Action
The primary result of DEPC’s action is the preservation of RNA integrity. By inhibiting RNase enzymes, DEPC prevents RNA degradation, allowing for the successful extraction and analysis of RNA in laboratory settings . This is crucial for various molecular biology techniques that rely on intact RNA, such as reverse transcription and RNA sequencing .
Action Environment
The action of DEPC is influenced by environmental factors such as pH and the presence of nucleophiles . DEPC is sensitive to moisture and pH, and it cannot be used with certain buffers like Tris or HEPES . It can be used with phosphate-buffered saline or mops . These factors must be carefully controlled to ensure the effective action of DEPC .
Safety and Hazards
DEPC is harmful if swallowed or inhaled . It causes skin irritation and serious eye irritation . It may cause respiratory irritation . It is recommended to avoid breathing dust/fume/gas/mist/vapors/spray and to use only outdoors or in a well-ventilated area . Protective gloves, protective clothing, eye protection, and face protection should be worn when handling DEPC .
Zukünftige Richtungen
Eigenschaften
IUPAC Name |
ethoxycarbonyl ethyl carbonate | |
---|---|---|
Source | PubChem | |
URL | https://pubchem.ncbi.nlm.nih.gov | |
Description | Data deposited in or computed by PubChem | |
InChI |
InChI=1S/C6H10O5/c1-3-9-5(7)11-6(8)10-4-2/h3-4H2,1-2H3 | |
Source | PubChem | |
URL | https://pubchem.ncbi.nlm.nih.gov | |
Description | Data deposited in or computed by PubChem | |
InChI Key |
FFYPMLJYZAEMQB-UHFFFAOYSA-N | |
Source | PubChem | |
URL | https://pubchem.ncbi.nlm.nih.gov | |
Description | Data deposited in or computed by PubChem | |
Canonical SMILES |
CCOC(=O)OC(=O)OCC | |
Source | PubChem | |
URL | https://pubchem.ncbi.nlm.nih.gov | |
Description | Data deposited in or computed by PubChem | |
Molecular Formula |
C6H10O5 | |
Source | PubChem | |
URL | https://pubchem.ncbi.nlm.nih.gov | |
Description | Data deposited in or computed by PubChem | |
DSSTOX Substance ID |
DTXSID2051764 | |
Record name | Diethyl pyrocarbonate | |
Source | EPA DSSTox | |
URL | https://comptox.epa.gov/dashboard/DTXSID2051764 | |
Description | DSSTox provides a high quality public chemistry resource for supporting improved predictive toxicology. | |
Molecular Weight |
162.14 g/mol | |
Source | PubChem | |
URL | https://pubchem.ncbi.nlm.nih.gov | |
Description | Data deposited in or computed by PubChem | |
Physical Description |
Viscous liquid with a fruity odor. [Merck Index] Clear colorless liquid; [Sigma-Aldrich MSDS], Solid | |
Record name | Diethyl pyrocarbonate | |
Source | Haz-Map, Information on Hazardous Chemicals and Occupational Diseases | |
URL | https://haz-map.com/Agents/13805 | |
Description | Haz-Map® is an occupational health database designed for health and safety professionals and for consumers seeking information about the adverse effects of workplace exposures to chemical and biological agents. | |
Explanation | Copyright (c) 2022 Haz-Map(R). All rights reserved. Unless otherwise indicated, all materials from Haz-Map are copyrighted by Haz-Map(R). No part of these materials, either text or image may be used for any purpose other than for personal use. Therefore, reproduction, modification, storage in a retrieval system or retransmission, in any form or by any means, electronic, mechanical or otherwise, for reasons other than personal use, is strictly prohibited without prior written permission. | |
Record name | Diethyl dicarbonate | |
Source | Human Metabolome Database (HMDB) | |
URL | http://www.hmdb.ca/metabolites/HMDB0032873 | |
Description | The Human Metabolome Database (HMDB) is a freely available electronic database containing detailed information about small molecule metabolites found in the human body. | |
Explanation | HMDB is offered to the public as a freely available resource. Use and re-distribution of the data, in whole or in part, for commercial purposes requires explicit permission of the authors and explicit acknowledgment of the source material (HMDB) and the original publication (see the HMDB citing page). We ask that users who download significant portions of the database cite the HMDB paper in any resulting publications. | |
Product Name |
Diethyl pyrocarbonate | |
CAS RN |
1609-47-8 | |
Record name | Diethyl dicarbonate | |
Source | CAS Common Chemistry | |
URL | https://commonchemistry.cas.org/detail?cas_rn=1609-47-8 | |
Description | CAS Common Chemistry is an open community resource for accessing chemical information. Nearly 500,000 chemical substances from CAS REGISTRY cover areas of community interest, including common and frequently regulated chemicals, and those relevant to high school and undergraduate chemistry classes. This chemical information, curated by our expert scientists, is provided in alignment with our mission as a division of the American Chemical Society. | |
Explanation | The data from CAS Common Chemistry is provided under a CC-BY-NC 4.0 license, unless otherwise stated. | |
Record name | Diethyl pyrocarbonate | |
Source | ChemIDplus | |
URL | https://pubchem.ncbi.nlm.nih.gov/substance/?source=chemidplus&sourceid=0001609478 | |
Description | ChemIDplus is a free, web search system that provides access to the structure and nomenclature authority files used for the identification of chemical substances cited in National Library of Medicine (NLM) databases, including the TOXNET system. | |
Record name | Dicarbonic acid, 1,3-diethyl ester | |
Source | EPA Chemicals under the TSCA | |
URL | https://www.epa.gov/chemicals-under-tsca | |
Description | EPA Chemicals under the Toxic Substances Control Act (TSCA) collection contains information on chemicals and their regulations under TSCA, including non-confidential content from the TSCA Chemical Substance Inventory and Chemical Data Reporting. | |
Record name | Diethyl pyrocarbonate | |
Source | EPA DSSTox | |
URL | https://comptox.epa.gov/dashboard/DTXSID2051764 | |
Description | DSSTox provides a high quality public chemistry resource for supporting improved predictive toxicology. | |
Record name | Diethyl pyrocarbonate | |
Source | European Chemicals Agency (ECHA) | |
URL | https://echa.europa.eu/substance-information/-/substanceinfo/100.015.039 | |
Description | The European Chemicals Agency (ECHA) is an agency of the European Union which is the driving force among regulatory authorities in implementing the EU's groundbreaking chemicals legislation for the benefit of human health and the environment as well as for innovation and competitiveness. | |
Explanation | Use of the information, documents and data from the ECHA website is subject to the terms and conditions of this Legal Notice, and subject to other binding limitations provided for under applicable law, the information, documents and data made available on the ECHA website may be reproduced, distributed and/or used, totally or in part, for non-commercial purposes provided that ECHA is acknowledged as the source: "Source: European Chemicals Agency, http://echa.europa.eu/". Such acknowledgement must be included in each copy of the material. ECHA permits and encourages organisations and individuals to create links to the ECHA website under the following cumulative conditions: Links can only be made to webpages that provide a link to the Legal Notice page. | |
Record name | DIETHYL PYROCARBONATE | |
Source | FDA Global Substance Registration System (GSRS) | |
URL | https://gsrs.ncats.nih.gov/ginas/app/beta/substances/LMR3LZG146 | |
Description | The FDA Global Substance Registration System (GSRS) enables the efficient and accurate exchange of information on what substances are in regulated products. Instead of relying on names, which vary across regulatory domains, countries, and regions, the GSRS knowledge base makes it possible for substances to be defined by standardized, scientific descriptions. | |
Explanation | Unless otherwise noted, the contents of the FDA website (www.fda.gov), both text and graphics, are not copyrighted. They are in the public domain and may be republished, reprinted and otherwise used freely by anyone without the need to obtain permission from FDA. Credit to the U.S. Food and Drug Administration as the source is appreciated but not required. | |
Record name | Diethyl dicarbonate | |
Source | Human Metabolome Database (HMDB) | |
URL | http://www.hmdb.ca/metabolites/HMDB0032873 | |
Description | The Human Metabolome Database (HMDB) is a freely available electronic database containing detailed information about small molecule metabolites found in the human body. | |
Explanation | HMDB is offered to the public as a freely available resource. Use and re-distribution of the data, in whole or in part, for commercial purposes requires explicit permission of the authors and explicit acknowledgment of the source material (HMDB) and the original publication (see the HMDB citing page). We ask that users who download significant portions of the database cite the HMDB paper in any resulting publications. | |
Melting Point |
< 25 °C | |
Record name | Diethyl dicarbonate | |
Source | Human Metabolome Database (HMDB) | |
URL | http://www.hmdb.ca/metabolites/HMDB0032873 | |
Description | The Human Metabolome Database (HMDB) is a freely available electronic database containing detailed information about small molecule metabolites found in the human body. | |
Explanation | HMDB is offered to the public as a freely available resource. Use and re-distribution of the data, in whole or in part, for commercial purposes requires explicit permission of the authors and explicit acknowledgment of the source material (HMDB) and the original publication (see the HMDB citing page). We ask that users who download significant portions of the database cite the HMDB paper in any resulting publications. | |
Synthesis routes and methods I
Procedure details
Synthesis routes and methods II
Procedure details
Retrosynthesis Analysis
AI-Powered Synthesis Planning: Our tool employs the Template_relevance Pistachio, Template_relevance Bkms_metabolic, Template_relevance Pistachio_ringbreaker, Template_relevance Reaxys, Template_relevance Reaxys_biocatalysis model, leveraging a vast database of chemical reactions to predict feasible synthetic routes.
One-Step Synthesis Focus: Specifically designed for one-step synthesis, it provides concise and direct routes for your target compounds, streamlining the synthesis process.
Accurate Predictions: Utilizing the extensive PISTACHIO, BKMS_METABOLIC, PISTACHIO_RINGBREAKER, REAXYS, REAXYS_BIOCATALYSIS database, our tool offers high-accuracy predictions, reflecting the latest in chemical research and data.
Strategy Settings
Precursor scoring | Relevance Heuristic |
---|---|
Min. plausibility | 0.01 |
Model | Template_relevance |
Template Set | Pistachio/Bkms_metabolic/Pistachio_ringbreaker/Reaxys/Reaxys_biocatalysis |
Top-N result to add to graph | 6 |
Feasible Synthetic Routes
Q & A
Q1: What is the primary target of DEPC in biological systems?
A1: DEPC primarily reacts with histidine residues in proteins. [, , , , , , ] This reaction forms N-carbethoxyhistidine, which can disrupt the protein's structure and function. [, , ]
Q2: Can DEPC modify other amino acids besides histidine?
A2: While histidine is the primary target, DEPC can also react with lysine, tyrosine, and cysteine residues under certain conditions. [, , , , , ]
Q3: How does DEPC modification affect enzyme activity?
A3: DEPC modification can lead to enzyme inactivation by disrupting the active site or altering the protein's conformation. [, , , , , , , , , , , , , ] The degree of inactivation depends on the specific enzyme and the number of essential residues modified.
Q4: Can the effects of DEPC modification be reversed?
A4: Yes, the modification of histidine residues by DEPC can often be reversed by treatment with hydroxylamine. [, , , , , , , , , , ] This reversibility makes DEPC a valuable tool for studying the role of histidine residues in protein function.
Q5: Does DEPC always inactivate enzymes?
A5: Not necessarily. In some cases, DEPC treatment can even enhance enzymatic activity. [, ] This suggests that DEPC can induce subtle conformational changes that may positively influence the active site in certain enzymes.
Q6: How does DEPC affect insulin binding to its receptor?
A6: DEPC inhibits insulin binding to its receptor in rat liver plasma membranes by decreasing the number of available receptors. [] This suggests that a histidine residue is crucial for the formation of the insulin-receptor complex.
Q7: Can DEPC distinguish between different types of binding sites?
A7: Yes, research shows that DEPC can differentially affect binding sites. For example, DEPC treatment of benzodiazepine receptors modifies a portion of the sites while leaving others unaffected, suggesting heterogeneity within this receptor population. []
Q8: What is the molecular formula and weight of DEPC?
A8: The molecular formula of DEPC is C6H10O5, and its molecular weight is 162.14 g/mol.
Q9: What spectroscopic data are available for DEPC?
A9: The reaction of DEPC with histidine residues results in a characteristic increase in absorbance at 240 nm, which can be monitored spectrophotometrically. [, , , , , , , , , ]
Q10: How stable is DEPC in solution?
A10: DEPC is unstable in aqueous solutions and hydrolyzes relatively quickly. [, ] This hydrolysis needs to be accounted for when designing experiments and interpreting kinetic data.
Q11: How does DEPC act as a nuclease inhibitor?
A11: DEPC inactivates nucleases by modifying histidine residues essential for their catalytic activity. [] This property makes it valuable for RNA isolation, ensuring RNA integrity.
Q12: What are other applications of DEPC beyond nuclease inhibition?
A12: DEPC is also used to probe the structure and function of proteins by selectively modifying histidine residues. [, , , , , , , , , , , , , , , , , , , ] This information helps to identify essential residues involved in catalysis, substrate binding, or protein-protein interactions.
Haftungsausschluss und Informationen zu In-Vitro-Forschungsprodukten
Bitte beachten Sie, dass alle Artikel und Produktinformationen, die auf BenchChem präsentiert werden, ausschließlich zu Informationszwecken bestimmt sind. Die auf BenchChem zum Kauf angebotenen Produkte sind speziell für In-vitro-Studien konzipiert, die außerhalb lebender Organismen durchgeführt werden. In-vitro-Studien, abgeleitet von dem lateinischen Begriff "in Glas", beinhalten Experimente, die in kontrollierten Laborumgebungen unter Verwendung von Zellen oder Geweben durchgeführt werden. Es ist wichtig zu beachten, dass diese Produkte nicht als Arzneimittel oder Medikamente eingestuft sind und keine Zulassung der FDA für die Vorbeugung, Behandlung oder Heilung von medizinischen Zuständen, Beschwerden oder Krankheiten erhalten haben. Wir müssen betonen, dass jede Form der körperlichen Einführung dieser Produkte in Menschen oder Tiere gesetzlich strikt untersagt ist. Es ist unerlässlich, sich an diese Richtlinien zu halten, um die Einhaltung rechtlicher und ethischer Standards in Forschung und Experiment zu gewährleisten.