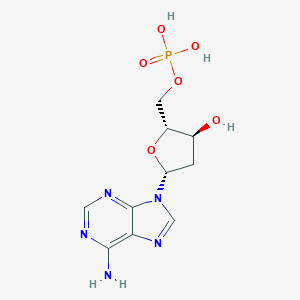
2'-Deoxyadenosine-5'-monophosphate
Übersicht
Beschreibung
2’-Deoxyadenosine-5’-monophosphate is a derivative of the nucleic acid, AMP, in which the hydroxyl group on the 2’’ carbon of the pentose has been reduced to a hydrogen atom . It is a nucleotide that serves as a building block for DNA synthesis .
Synthesis Analysis
2’-Deoxyadenosine-5’-monophosphate is a deoxyribonucleotide found in DNA. It can be used to study adenosine-based interactions during DNA synthesis and DNA damage . It may be used for the synthesis of deoxyadenylate oligonucleotides with enzymes such as polynucleotide phosphorylase from Escherichia coli .
Molecular Structure Analysis
The molecular formula of 2’-Deoxyadenosine-5’-monophosphate is C10H14N5O6P. The average molecular weight is 331.222 Da .
Chemical Reactions Analysis
The oxidation of 2’-deoxyadenosine-5’-monophosphate can be photosensitized by specific compounds in aqueous solutions under UV irradiation . The oxidation takes place through a type I mechanism, in which the excitation of the photosensitizer is followed by an electron transfer from the 2’-deoxyadenosine-5’-monophosphate molecule to the photosensitizer’s triplet excited state .
Wissenschaftliche Forschungsanwendungen
Here is a comprehensive analysis of the scientific research applications of 2’-Deoxyadenosine-5’-monophosphate, focusing on unique applications:
DNA Synthesis and Repair
2’-Deoxyadenosine-5’-monophosphate serves as a building block for DNA synthesis. It is crucial in the study of DNA replication and repair mechanisms, providing insights into cellular processes and genetic disorders .
Protein Studies via UV Resonance Raman Spectroscopy
This compound has been utilized in studying proteins through UV resonance Raman spectroscopy, a technique that offers detailed information about protein structure and dynamics .
Photoaffinity Labeling
Researchers have used 2’-Deoxyadenosine-5’-monophosphate in the synthesis of novel photoaffinity labels for incorporation into DNA. This application is significant for studying adenosine-based interactions during DNA synthesis and DNA damage .
Electrochemical Studies
The electrochemical oxidation of 2’-Deoxyadenosine-5’-monophosphate has been explored at pyrolytic graphite electrodes. These studies are important for understanding the biological redox chemistry of nucleotides .
Metabolomic Research
As a metabolite, 2’-Deoxyadenosine-5’-monophosphate is studied within the field of metabolomics, which can lead to discoveries in disease mechanisms and therapeutic targets .
Wirkmechanismus
Target of Action
2’-Deoxyadenosine-5’-monophosphate (D-AMP) is a nucleoside comprised of adenine attached to a ribose (ribofuranose) moiety via a -N9-glycosidic bond . It is a derivative of the common nucleic acid ATP, or adenosine triphosphate . The primary targets of D-AMP are the enzymes involved in DNA synthesis and DNA damage processes .
Mode of Action
D-AMP interacts with its targets by serving as a building block for DNA synthesis . It is incorporated into the DNA strand during replication, replacing the regular adenosine monophosphate (AMP). This interaction results in changes to the DNA structure and function .
Biochemical Pathways
D-AMP affects the DNA synthesis pathway, specifically the process of replication . It is incorporated into the growing DNA strand, effectively altering the sequence and structure of the DNA. This can have downstream effects on gene expression and protein synthesis, potentially influencing various cellular processes .
Result of Action
The incorporation of D-AMP into DNA can result in changes to the DNA sequence and structure . This can affect gene expression and protein synthesis, potentially leading to alterations in cellular function. The specific molecular and cellular effects would depend on the context, including the specific genes and pathways involved .
Action Environment
The action, efficacy, and stability of D-AMP can be influenced by various environmental factors. For example, the pH of the environment can affect its stability, with D-AMP being photosensitive and degrading under acidic conditions . Additionally, the presence of specific enzymes and cofactors, the rate of cellular metabolism, and other factors can influence the action and efficacy of D-AMP .
Safety and Hazards
Eigenschaften
IUPAC Name |
[(2R,3S,5R)-5-(6-aminopurin-9-yl)-3-hydroxyoxolan-2-yl]methyl dihydrogen phosphate | |
---|---|---|
Source | PubChem | |
URL | https://pubchem.ncbi.nlm.nih.gov | |
Description | Data deposited in or computed by PubChem | |
InChI |
InChI=1S/C10H14N5O6P/c11-9-8-10(13-3-12-9)15(4-14-8)7-1-5(16)6(21-7)2-20-22(17,18)19/h3-7,16H,1-2H2,(H2,11,12,13)(H2,17,18,19)/t5-,6+,7+/m0/s1 | |
Source | PubChem | |
URL | https://pubchem.ncbi.nlm.nih.gov | |
Description | Data deposited in or computed by PubChem | |
InChI Key |
KHWCHTKSEGGWEX-RRKCRQDMSA-N | |
Source | PubChem | |
URL | https://pubchem.ncbi.nlm.nih.gov | |
Description | Data deposited in or computed by PubChem | |
Canonical SMILES |
C1C(C(OC1N2C=NC3=C(N=CN=C32)N)COP(=O)(O)O)O | |
Source | PubChem | |
URL | https://pubchem.ncbi.nlm.nih.gov | |
Description | Data deposited in or computed by PubChem | |
Isomeric SMILES |
C1[C@@H]([C@H](O[C@H]1N2C=NC3=C(N=CN=C32)N)COP(=O)(O)O)O | |
Source | PubChem | |
URL | https://pubchem.ncbi.nlm.nih.gov | |
Description | Data deposited in or computed by PubChem | |
Molecular Formula |
C10H14N5O6P | |
Source | PubChem | |
URL | https://pubchem.ncbi.nlm.nih.gov | |
Description | Data deposited in or computed by PubChem | |
Related CAS |
25191-20-2, 2922-74-9 (di-hydrochloride salt) | |
Record name | Poly(dA) | |
Source | CAS Common Chemistry | |
URL | https://commonchemistry.cas.org/detail?cas_rn=25191-20-2 | |
Description | CAS Common Chemistry is an open community resource for accessing chemical information. Nearly 500,000 chemical substances from CAS REGISTRY cover areas of community interest, including common and frequently regulated chemicals, and those relevant to high school and undergraduate chemistry classes. This chemical information, curated by our expert scientists, is provided in alignment with our mission as a division of the American Chemical Society. | |
Explanation | The data from CAS Common Chemistry is provided under a CC-BY-NC 4.0 license, unless otherwise stated. | |
Record name | 2'-Deoxy-5'-adenosine monophosphate | |
Source | ChemIDplus | |
URL | https://pubchem.ncbi.nlm.nih.gov/substance/?source=chemidplus&sourceid=0000653634 | |
Description | ChemIDplus is a free, web search system that provides access to the structure and nomenclature authority files used for the identification of chemical substances cited in National Library of Medicine (NLM) databases, including the TOXNET system. | |
DSSTOX Substance ID |
DTXSID901034859 | |
Record name | 2'-Deoxy-5'-adenosine monophosphate | |
Source | EPA DSSTox | |
URL | https://comptox.epa.gov/dashboard/DTXSID901034859 | |
Description | DSSTox provides a high quality public chemistry resource for supporting improved predictive toxicology. | |
Molecular Weight |
331.22 g/mol | |
Source | PubChem | |
URL | https://pubchem.ncbi.nlm.nih.gov | |
Description | Data deposited in or computed by PubChem | |
Physical Description |
Solid | |
Record name | Deoxyadenosine monophosphate | |
Source | Human Metabolome Database (HMDB) | |
URL | http://www.hmdb.ca/metabolites/HMDB0000905 | |
Description | The Human Metabolome Database (HMDB) is a freely available electronic database containing detailed information about small molecule metabolites found in the human body. | |
Explanation | HMDB is offered to the public as a freely available resource. Use and re-distribution of the data, in whole or in part, for commercial purposes requires explicit permission of the authors and explicit acknowledgment of the source material (HMDB) and the original publication (see the HMDB citing page). We ask that users who download significant portions of the database cite the HMDB paper in any resulting publications. | |
Product Name |
2'-Deoxyadenosine-5'-monophosphate | |
CAS RN |
653-63-4 | |
Record name | dAMP | |
Source | CAS Common Chemistry | |
URL | https://commonchemistry.cas.org/detail?cas_rn=653-63-4 | |
Description | CAS Common Chemistry is an open community resource for accessing chemical information. Nearly 500,000 chemical substances from CAS REGISTRY cover areas of community interest, including common and frequently regulated chemicals, and those relevant to high school and undergraduate chemistry classes. This chemical information, curated by our expert scientists, is provided in alignment with our mission as a division of the American Chemical Society. | |
Explanation | The data from CAS Common Chemistry is provided under a CC-BY-NC 4.0 license, unless otherwise stated. | |
Record name | 2'-Deoxy-5'-adenosine monophosphate | |
Source | ChemIDplus | |
URL | https://pubchem.ncbi.nlm.nih.gov/substance/?source=chemidplus&sourceid=0000653634 | |
Description | ChemIDplus is a free, web search system that provides access to the structure and nomenclature authority files used for the identification of chemical substances cited in National Library of Medicine (NLM) databases, including the TOXNET system. | |
Record name | 2'-Deoxy-5'-adenosine monophosphate | |
Source | EPA DSSTox | |
URL | https://comptox.epa.gov/dashboard/DTXSID901034859 | |
Description | DSSTox provides a high quality public chemistry resource for supporting improved predictive toxicology. | |
Record name | 2'-deoxyadenosine 5'-(dihydrogen phosphate) | |
Source | European Chemicals Agency (ECHA) | |
URL | https://echa.europa.eu/substance-information/-/substanceinfo/100.010.459 | |
Description | The European Chemicals Agency (ECHA) is an agency of the European Union which is the driving force among regulatory authorities in implementing the EU's groundbreaking chemicals legislation for the benefit of human health and the environment as well as for innovation and competitiveness. | |
Explanation | Use of the information, documents and data from the ECHA website is subject to the terms and conditions of this Legal Notice, and subject to other binding limitations provided for under applicable law, the information, documents and data made available on the ECHA website may be reproduced, distributed and/or used, totally or in part, for non-commercial purposes provided that ECHA is acknowledged as the source: "Source: European Chemicals Agency, http://echa.europa.eu/". Such acknowledgement must be included in each copy of the material. ECHA permits and encourages organisations and individuals to create links to the ECHA website under the following cumulative conditions: Links can only be made to webpages that provide a link to the Legal Notice page. | |
Record name | DEOXYADENOSINE 5'-MONOPHOSPHATE | |
Source | FDA Global Substance Registration System (GSRS) | |
URL | https://gsrs.ncats.nih.gov/ginas/app/beta/substances/VFR8I97ORM | |
Description | The FDA Global Substance Registration System (GSRS) enables the efficient and accurate exchange of information on what substances are in regulated products. Instead of relying on names, which vary across regulatory domains, countries, and regions, the GSRS knowledge base makes it possible for substances to be defined by standardized, scientific descriptions. | |
Explanation | Unless otherwise noted, the contents of the FDA website (www.fda.gov), both text and graphics, are not copyrighted. They are in the public domain and may be republished, reprinted and otherwise used freely by anyone without the need to obtain permission from FDA. Credit to the U.S. Food and Drug Administration as the source is appreciated but not required. | |
Record name | Deoxyadenosine monophosphate | |
Source | Human Metabolome Database (HMDB) | |
URL | http://www.hmdb.ca/metabolites/HMDB0000905 | |
Description | The Human Metabolome Database (HMDB) is a freely available electronic database containing detailed information about small molecule metabolites found in the human body. | |
Explanation | HMDB is offered to the public as a freely available resource. Use and re-distribution of the data, in whole or in part, for commercial purposes requires explicit permission of the authors and explicit acknowledgment of the source material (HMDB) and the original publication (see the HMDB citing page). We ask that users who download significant portions of the database cite the HMDB paper in any resulting publications. | |
Melting Point |
148 °C | |
Record name | Deoxyadenosine monophosphate | |
Source | Human Metabolome Database (HMDB) | |
URL | http://www.hmdb.ca/metabolites/HMDB0000905 | |
Description | The Human Metabolome Database (HMDB) is a freely available electronic database containing detailed information about small molecule metabolites found in the human body. | |
Explanation | HMDB is offered to the public as a freely available resource. Use and re-distribution of the data, in whole or in part, for commercial purposes requires explicit permission of the authors and explicit acknowledgment of the source material (HMDB) and the original publication (see the HMDB citing page). We ask that users who download significant portions of the database cite the HMDB paper in any resulting publications. | |
Retrosynthesis Analysis
AI-Powered Synthesis Planning: Our tool employs the Template_relevance Pistachio, Template_relevance Bkms_metabolic, Template_relevance Pistachio_ringbreaker, Template_relevance Reaxys, Template_relevance Reaxys_biocatalysis model, leveraging a vast database of chemical reactions to predict feasible synthetic routes.
One-Step Synthesis Focus: Specifically designed for one-step synthesis, it provides concise and direct routes for your target compounds, streamlining the synthesis process.
Accurate Predictions: Utilizing the extensive PISTACHIO, BKMS_METABOLIC, PISTACHIO_RINGBREAKER, REAXYS, REAXYS_BIOCATALYSIS database, our tool offers high-accuracy predictions, reflecting the latest in chemical research and data.
Strategy Settings
Precursor scoring | Relevance Heuristic |
---|---|
Min. plausibility | 0.01 |
Model | Template_relevance |
Template Set | Pistachio/Bkms_metabolic/Pistachio_ringbreaker/Reaxys/Reaxys_biocatalysis |
Top-N result to add to graph | 6 |
Feasible Synthetic Routes
Q & A
Q1: What is the molecular formula and weight of dAMP?
A1: The molecular formula of dAMP is C10H14N5O6P, and its molecular weight is 331.22 g/mol.
Q2: How can spectroscopic techniques differentiate between adenine and dAMP when complexed with silver?
A2: Surface-enhanced Raman scattering (SERS) reveals distinct spectral differences between silver-adenine and silver-dAMP complexes. Silver-adenine complexes exhibit broad, unresolved bands between 1200 and 1500 cm-1, while silver-dAMP complexes show clearly resolved bands in the same region. [] This distinction arises from the variety of complexes adenine can form with silver, which are not possible for dAMP.
Q3: How does pterin interact with dAMP under UV-A radiation?
A3: Pterin (PT) can photosensitize dAMP under UV-A radiation. Kinetic studies suggest that singlet oxygen generated by excited PT is not the primary reactive species. Instead, the photosensitized oxidation likely occurs via a type I mechanism involving electron transfer, as the formation of 8-oxo-7,8-dihydro-2′-deoxyadenosine-5′-monophosphate (8-oxo-dAMP) is observed. []
Q4: Can phenylpropanoid glycosides protect dAMP from oxidative damage?
A4: Yes, phenylpropanoid glycosides like angoroside C and acteoside, isolated from Scrophularia ningpoensis, can effectively repair oxidized hydroxyl radical adducts of dAMP. These compounds achieve this by rapidly transferring electrons to the damaged dAMP molecules. []
Q5: How does cisplatin interact with dAMP?
A5: Mass spectrometry and IRMPD spectroscopy studies reveal that cisplatin can coordinate with dAMP at the N3 or N1 positions of the adenine ring. Surprisingly, the N3 isomer, though slightly less stable in the gas phase, is more abundant than the N1 isomer. This suggests that the reaction kinetics in solution, rather than thermodynamic factors, govern the interaction between cisplatin and dAMP. []
Q6: Does the presence of dAMP affect the binding of TATA-binding protein (TBP) to DNA?
A6: Yes, incorporating the anti-leukemic nucleotide analog, 2-chloro-2′-deoxyadenosine-5′-monophosphate, into a promoter sequence alters the DNA binding affinity of TBP. [] This suggests potential implications for gene expression and anticancer activity.
Q7: How do zinc ions influence the fluorescence properties of dAMP?
A7: While dAMP exhibits weak intrinsic fluorescence, complexation with zinc ions significantly enhances its fluorescence intensity. Moreover, the fluorescence spectrum of the Zn(II)–dAMP complex shows a distinct shift compared to free dAMP. This phenomenon allows for the optical detection and differentiation of dAMP from other nucleotides. []
Q8: Can low-energy electrons directly damage dAMP?
A8: Yes, X-ray photoelectron spectroscopy studies demonstrate that low-energy electrons (3–25 eV) can directly induce damage to condensed dAMP. This damage includes decomposition of the base and sugar moieties, as well as cleavage of glycosidic and phosphoester bonds. []
Q9: How does lumazine affect dAMP under UV irradiation?
A9: Lumazine (Lum) can photosensitize the oxidation of dAMP in aqueous solutions under UV irradiation. The mechanism involves electron transfer from dAMP to the triplet excited state of Lum, generating superoxide radicals and ultimately hydrogen peroxide. This process is pH-dependent and primarily observed with the acidic form of Lum. []
Q10: What role does dAMP play in the adaptation of plateau pikas to high altitude?
A10: Metabolic profiling of high-altitude pikas reveals significant alterations in various metabolic pathways, including those involving dAMP. Specifically, pikas living at higher altitudes exhibit decreased levels of dAMP in their myocardium, suggesting a potential role for dAMP in their adaptation to hypoxia. []
Q11: Can dAMP be used to study the RNase H activity of hepatitis B virus polymerase?
A11: Yes, dAMP can inhibit the RNase H activity of the human hepatitis B virus polymerase. This inhibition can be utilized in in vitro assays to study the enzyme's activity and characterize its properties. []
Q12: What is the role of 2-azido analogs of dAMP in nucleotide research?
A12: 2-Azido analogs of dAMP, such as 2-azido-1,N6-etheno-2′-deoxyadenosine 5′-monophosphate, serve as valuable nucleotide photoaffinity probes. These analogs can be incorporated into DNA and, upon UV irradiation, form covalent bonds with nearby molecules, allowing researchers to study DNA-protein interactions and identify binding sites. []
Q13: Can aristolochic acid I form adducts with dAMP?
A13: Yes, aristolochic acid I (AAI) can bind covalently to the exocyclic amino group of dAMP to form AAI-DNA adducts. These adducts can be detected and characterized using techniques like liquid chromatography electrospray ionization/tandem mass spectrometry (LC-ESI-MS/MS). [, ]
Q14: Can dAMP be encapsulated in silica gels, and if so, how does its conformation affect the process?
A14: dAMP can be successfully encapsulated in silica gels using sol-gel methods. The encapsulation process does not disrupt the helical conformation of double-stranded DNA, and the interaction between dAMP and the silica network is primarily through physical association rather than chemical bonding. This interaction occurs through the phosphate groups of dAMP and the silanol groups of the silica, with molecular water acting as a barrier. []
Q15: How does the PM3 method perform in predicting hydrogen bonding interactions in dAMP and DNA base pairs?
A15: The PM3 semiempirical method, while an improvement over earlier models, shows limitations in accurately predicting hydrogen bonding in dAMP and DNA base pairs. It tends to underestimate hydrogen bond lengths and stacking interactions. Accurate modeling of these systems often requires higher-level computational methods. []
Q16: Can computational methods provide insights into the electron affinity and potential for proton transfer in dAMP anions?
A16: Yes, DFT calculations reveal that while isolated adenine doesn't easily form stable valence anions, neutral dAMP readily captures an electron, forming an adiabatically stable anion. This process is often accompanied by a barrier-free proton transfer and internal rotation. The predicted electron affinity and vertical detachment energy for the most stable dAMP anion align well with experimental photoelectron spectroscopy data. []
Q17: What is the significance of understanding dAMP's interaction with metal ions in biological systems?
A17: Metal ions play critical roles in numerous biological processes, and their interactions with nucleotides like dAMP are crucial for maintaining DNA stability and function. For instance, metal ions like Mg2+, Ni2+, Cu2+, and Zn2+ exhibit distinct binding affinities and preferences for dAMP compared to AMP, influencing their roles in enzymatic reactions and cellular signaling. []
Haftungsausschluss und Informationen zu In-Vitro-Forschungsprodukten
Bitte beachten Sie, dass alle Artikel und Produktinformationen, die auf BenchChem präsentiert werden, ausschließlich zu Informationszwecken bestimmt sind. Die auf BenchChem zum Kauf angebotenen Produkte sind speziell für In-vitro-Studien konzipiert, die außerhalb lebender Organismen durchgeführt werden. In-vitro-Studien, abgeleitet von dem lateinischen Begriff "in Glas", beinhalten Experimente, die in kontrollierten Laborumgebungen unter Verwendung von Zellen oder Geweben durchgeführt werden. Es ist wichtig zu beachten, dass diese Produkte nicht als Arzneimittel oder Medikamente eingestuft sind und keine Zulassung der FDA für die Vorbeugung, Behandlung oder Heilung von medizinischen Zuständen, Beschwerden oder Krankheiten erhalten haben. Wir müssen betonen, dass jede Form der körperlichen Einführung dieser Produkte in Menschen oder Tiere gesetzlich strikt untersagt ist. Es ist unerlässlich, sich an diese Richtlinien zu halten, um die Einhaltung rechtlicher und ethischer Standards in Forschung und Experiment zu gewährleisten.