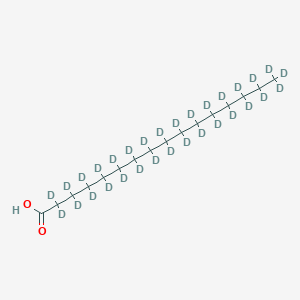
Hexadecanoic-d31 acid
Übersicht
Beschreibung
Hexadecanoic-d31 acid is a deuterated isotopologue of hexadecanoic acid (palmitic acid), where 31 hydrogen atoms are replaced with deuterium. This compound is primarily utilized in research as a stable isotopic tracer in metabolic, environmental, and biochemical studies. Its non-deuterated counterpart, hexadecanoic acid (C₁₆H₃₂O₂), is a saturated long-chain fatty acid ubiquitously found in biological systems and plant-derived materials .
Vorbereitungsmethoden
The most widely cited method involves catalytic deuteration of palmitic acid precursors under high-pressure deuterium gas. Yamada et al. (2016) achieved a 99% yield by reacting 1-hexadecylcarboxylic acid with d8-isopropanol in the presence of 10% Pt/activated carbon and deuterium oxide (D2O) at 120°C for 24 hours . A subsequent hydrolysis step with sodium hydroxide at 70°C for 24 hours removed protecting groups and finalized the deuteration.
Reaction Mechanism
The platinum catalyst facilitates H/D exchange at all aliphatic positions via a radical-mediated pathway. Isotopic scrambling is minimized by maintaining a large excess of D2O, ensuring near-complete deuteration. Kinetic studies reveal that the reaction follows pseudo-first-order kinetics, with an activation energy of .
Optimization Parameters
-
Temperature : Reactions below 100°C result in incomplete deuteration (<90%), while temperatures above 130°C promote decomposition.
-
Solvent : d8-Isopropanol enhances solubility of the fatty acid precursor without introducing protiated contaminants.
-
Catalyst Loading : 10 wt% Pt/C achieves optimal activity; higher loadings cause side reactions (e.g., over-reduction of carboxylic acid groups) .
Dithiane-Based Cryptoregiochemical Labeling
For studies requiring site-specific deuteration, the dithiane intermediate approach enables precise labeling at C13 and C14 positions. A 2007 Journal of Organic Chemistry study synthesized hexadeuterated palmitic acid by incorporating a dithiane ring at C13–C14, followed by deuterium exchange and ring-opening .
Synthetic Steps
-
Dithiane Formation : 11-Hexadecynoic acid is treated with 1,2-ethanedithiol under BF3·Et2O catalysis to form a cyclic dithiane.
-
Deuteration : The dithiane undergoes H/D exchange using D2O and DC1, introducing six deuteriums at C13 and C14.
-
Reductive Ring-Opening : NiCl2/NaBD4 reduces the dithiane to yield a deuterated alkane chain.
This method achieves >98% isotopic purity at targeted positions but requires multi-step purification, limiting its scalability .
1,1’-Carbonyldiimidazole (CDI) Activation for Deuterated Triglycerides
A 2023 European Journal of Organic Chemistry study adapted CDI-mediated activation to synthesize deuterated triglycerides, which can be hydrolyzed to isolate this compound . Fatty acids are first activated with CDI, forming imidazolides that react with deuterated glycerol under mild conditions.
Key Advantages
-
Mild Conditions : Reactions proceed at 25°C, avoiding thermal degradation.
-
Functional Group Tolerance : CDI activation preserves unsaturated bonds, enabling synthesis of deuterated unsaturated analogs.
-
Yield : 85–92% for triglyceride intermediates, with subsequent hydrolysis yielding 97% pure this compound .
Enzymatic Synthesis Using Lipases
Enzyme-assisted methods employ lipases to catalyze deuteration in aqueous media. A 2020 ACS Omega study demonstrated that Candida antarctica lipase B (CAL-B) efficiently incorporates deuterium into fatty acid chains during transesterification .
Protocol
-
Substrate Preparation : Methyl palmitate and D2O are mixed in a 1:5 molar ratio.
-
Enzymatic Reaction : CAL-B (10 mg/mL) catalyzes H/D exchange at 37°C for 48 hours.
-
Acidification : HCl hydrolysis converts the ester to free this compound.
This method achieves 80–85% deuteration but requires iterative cycles to reach >95% isotopic enrichment .
Comparative Analysis of Preparation Methods
Method | Yield (%) | Isotopic Purity (%) | Temperature (°C) | Scalability |
---|---|---|---|---|
Catalytic Deuteration | 99 | 99.5 | 120 | High |
Dithiane Labeling | 75 | 98.0 | 25–80 | Low |
CDI Activation | 92 | 97.0 | 25 | Moderate |
Enzymatic Synthesis | 85 | 95.0 | 37 | Moderate |
Analyse Chemischer Reaktionen
Arten von Reaktionen
Palmitinsäure-d31 durchläuft verschiedene chemische Reaktionen, darunter:
Oxidation: Palmitinsäure-d31 kann oxidiert werden, um entsprechende Ketone und Aldehyde zu produzieren.
Reduktion: Es kann reduziert werden, um Alkohole zu bilden.
Substitution: Die Carboxylgruppe kann durch andere funktionelle Gruppen substituiert werden.
Häufige Reagenzien und Bedingungen
Oxidation: Häufige Oxidationsmittel sind Kaliumpermanganat (KMnO4) und Chromtrioxid (CrO3).
Reduktion: Als Reduktionsmittel werden Lithiumaluminiumhydrid (LiAlH4) und Natriumborhydrid (NaBH4) verwendet.
Substitution: Reagenzien wie Thionylchlorid (SOCl2) und Phosphortribromid (PBr3) werden für Substitutionsreaktionen verwendet.
Hauptprodukte, die gebildet werden
Oxidation: Produziert Ketone und Aldehyde.
Reduktion: Bildet Alkohole.
Substitution: Führt zur Bildung von Estern und Amiden.
Wissenschaftliche Forschungsanwendungen
Metabolic Research
Hexadecanoic-d31 Acid in Metabolism Studies
This compound serves as a valuable tool in metabolic research, particularly in understanding fatty acid metabolism and its implications in health and disease. Its deuterated nature allows for precise tracking of metabolic pathways using techniques such as mass spectrometry and nuclear magnetic resonance (NMR).
- Case Study: Fatty Acid Uptake in Cancer Cells
A study investigated the uptake of this compound in prostate cancer cells (PC-3). The results indicated that the uptake of D31-PA was significantly higher compared to untreated cells, demonstrating its utility in assessing fatty acid metabolism under different conditions. The uptake rates were monitored over time, revealing a continuous increase within the first 90 minutes post-treatment, followed by a gradual decline .
Analytical Chemistry
Quantification of Fatty Acids
This compound is commonly used as an internal standard for the quantification of fatty acids in various samples. Its incorporation into analytical methods such as gas chromatography (GC) and liquid chromatography (LC) enhances the accuracy of fatty acid measurements.
- Application Example: GC-MS Analysis
In analytical settings, this compound is utilized to calibrate assays for quantifying total fatty acids from biological samples. This application is particularly important for studies involving lipid profiles in clinical samples or dietary assessments .
Nutritional Studies
Assessment of Dietary Fatty Acid Oxidation
This compound can be employed to assess dietary fatty acid oxidation during nutritional interventions. By tracking the metabolism of deuterated fatty acids, researchers can gain insights into how different diets affect fat metabolism.
- Research Findings
A study demonstrated that administering this compound alongside H2(18)O allowed for the assessment of dietary fatty acid oxidation rates in subjects undergoing various nutritional interventions. This method provided valuable data on how specific dietary components influence metabolic pathways .
Biological Tracers
Use in Animal Models
In animal studies, this compound is used as a tracer to evaluate metabolic changes associated with diseases such as non-alcoholic fatty liver disease (NAFLD). The ability to visualize and quantify the metabolism of this compound provides insights into disease mechanisms.
- Experimental Setup
In vivo experiments have shown that administering this compound can help elucidate differences in fatty acid metabolism between healthy and diseased states. For instance, higher concentrations of palmitic acid were observed in animal models with metabolic disorders compared to controls .
Summary Table of Applications
Application Area | Description | Key Findings/Notes |
---|---|---|
Metabolic Research | Tracking fatty acid metabolism using NMR and mass spectrometry | Significant uptake in cancer cells |
Analytical Chemistry | Internal standard for quantifying fatty acids | Enhances accuracy in GC/LC analyses |
Nutritional Studies | Assessing dietary fatty acid oxidation | Provides insights into dietary impacts |
Biological Tracers | Evaluating metabolic changes in animal models | Useful for studying NAFLD and other diseases |
Wirkmechanismus
Palmitic Acid-d31 exerts its effects by mimicking the behavior of palmitic acid in biological systems. It is incorporated into cellular membranes and metabolic pathways, allowing researchers to study the metabolism and function of fatty acids. The deuterium atoms in Palmitic Acid-d31 provide a distinct mass shift, making it easily detectable in mass spectrometry .
Vergleich Mit ähnlichen Verbindungen
Chemical Structure and Physical Properties
Hexadecanoic acid (palmitic acid) is a straight-chain 16-carbon fatty acid with the formula CH₃(CH₂)₁₄COOH. Key comparisons with structurally similar compounds are outlined below:
Hexadecanoic-d31 acid retains the carboxylic acid group but exhibits distinct physicochemical properties (e.g., higher molecular weight, altered vibrational modes) due to deuterium substitution, enhancing its utility in mass spectrometry and nuclear magnetic resonance (NMR) studies.
Comparison with Esters and Derivatives
Hexadecanoic acid derivatives exhibit distinct bioactivities and applications:
Table 2: Functional Comparisons of Hexadecanoic Acid Derivatives
Comparison with Other Fatty Acids
Research Implications
This compound’s primary research value lies in its isotopic labeling capability, enabling precise tracking in:
- Environmental Science: Studying aerosol hygroscopicity (κ values for hexadecanoic acid-AS systems show an "S" shape, similar to marine aerosols) .
- Metabolic Engineering : Tracing fatty acid oxidation and lipid accumulation in hepatic studies .
- Phytochemistry : Isolating bioactive compounds in traditional medicines (e.g., Lilium-Belamcanda decoction ).
Biologische Aktivität
Hexadecanoic-d31 acid, also known as palmitic acid-d31, is a deuterated form of palmitic acid, a common saturated fatty acid with various biological activities. This article explores its antibacterial and antioxidant properties, mechanisms of action, and potential applications in pharmaceuticals based on diverse research findings.
- Molecular Formula : CHDO
- Molecular Weight : 287.62 g/mol
- CAS Number : 39756-30-4
Antibacterial Activity
This compound has been studied for its antibacterial properties against various pathogens. Research indicates that it exhibits moderate antibacterial activity against a range of bacteria, including:
- Escherichia coli
- Klebsiella pneumoniae
- Bacillus subtilis
- Aeromonas hydrophila
- Vibrio harveyi
Case Studies
-
Study on Excoecaria Agallocha L. :
- Objective : Evaluate the antibacterial and antioxidant properties of n-hexadecanoic acid isolated from the leaves.
- Method : The compound was extracted using Soxhlet extraction with methanol and fractionated.
- Results : The study reported inhibition zones of 11.02 mm to 13.2 mm against various bacteria at a concentration of 150 µg/ml, highlighting its potential as a natural antibacterial agent .
-
Antibacterial Activities Against Multidrug-Resistant Bacteria :
- Research Focus : The effectiveness of hexadecanoic acid methyl ester against multidrug-resistant (MDR) bacteria.
- Findings : The study demonstrated significant antimicrobial effects, suggesting that hexadecanoic acid derivatives could be utilized in developing treatments for bacterial infections resistant to conventional antibiotics .
Antioxidant Activity
This compound also exhibits notable antioxidant properties. A study utilizing the DPPH assay indicated that the compound showed antioxidant activity ranging from 65.52% to 87.40% at concentrations between 100 µg/ml and 500 µg/ml. This suggests its potential use in formulations aimed at combating oxidative stress .
The mechanisms by which this compound exerts its biological effects include:
- Membrane Disruption : The fatty acid can integrate into bacterial membranes, altering their permeability and leading to cell lysis.
- Inhibition of Biofilm Formation : It may affect the ability of bacteria to form biofilms, which are critical for their survival and resistance.
- Antioxidant Mechanism : By scavenging free radicals, this compound helps mitigate oxidative damage within cells.
Applications in Pharmaceuticals
Given its antibacterial and antioxidant properties, this compound presents several potential applications:
- Natural Preservatives : Its antimicrobial activity can be harnessed in food preservation and cosmetic formulations.
- Pharmaceuticals : As a component in drug formulations targeting bacterial infections or oxidative stress-related conditions.
- Research Tools : Used as an internal standard in mass spectrometry for quantifying fatty acids in biological samples .
Q & A
Basic Research Questions
Q. How can Hexadecanoic-d31 acid be reliably identified and quantified in biological matrices using analytical techniques?
- Methodology : Gas chromatography-mass spectrometry (GC-MS) is a primary method for identifying deuterated fatty acids like Hexadecanoic-d31. The isotopic substitution (d31) provides a distinct mass spectral signature, enabling differentiation from non-deuterated analogs. For quantification, use internal standards with similar isotopic labeling and compare retention times and fragmentation patterns against reference libraries (e.g., NIST Chemistry WebBook) . Calibration curves should be constructed using spiked matrices to account for matrix effects.
Q. What biological roles or pathways involve Hexadecanoic acid, and how does its deuterated form (d31) enhance mechanistic studies?
- Methodology : Hexadecanoic acid (palmitic acid) is a key saturated fatty acid in lipid metabolism, membrane synthesis, and energy storage. The deuterated form (d31) serves as a stable isotope tracer in metabolic flux analysis, enabling precise tracking via techniques like LC-MS/MS. For example, in lipidomics, d31-labeled analogs minimize interference from endogenous compounds, improving signal specificity in studies of β-oxidation or lipid turnover .
Q. What safety protocols are critical when handling this compound in laboratory settings?
- Methodology : While specific data for the deuterated form may be limited, standard protocols for fatty acids apply. Use chemical-resistant gloves (e.g., nitrile) and flame-retardant lab coats to prevent skin contact. Ensure proper ventilation to avoid inhalation of aerosols. Refer to safety data sheets (SDS) for non-deuterated analogs (e.g., Hexadecanoic acid) for hazard classifications (e.g., skin/eye irritation) and disposal guidelines .
Advanced Research Questions
Q. How can researchers resolve contradictions in reported biological activities of Hexadecanoic acid across studies (e.g., pro-inflammatory vs. anti-inflammatory effects)?
- Methodology : Discrepancies often arise from concentration-dependent effects or matrix interactions. For example, at high concentrations (>100 µM), Hexadecanoic acid may induce pro-inflammatory responses in cell models, while lower doses (<50 µM) show anti-inflammatory activity in Euphorbia milii extracts . Use dose-response experiments with standardized cell lines (e.g., RAW 264.7 macrophages) and include controls for lipid solubility and carrier solvents (e.g., DMSO vs. albumin-bound forms).
Q. What experimental designs are optimal for studying the isotopic effects of this compound in polymer composites or material science applications?
- Methodology : In polymer research, deuterated fatty acids can alter crystallization kinetics or thermal stability. For instance, in polylactic acid (PLA) composites, use differential scanning calorimetry (DSC) and scanning electron microscopy (SEM) to compare domain formation between deuterated and non-deuterated analogs. Ensure consistent blending ratios (e.g., 4.4 g this compound per 75 g PLA) and monitor phase separation under controlled temperature gradients .
Q. How do variations in Hexadecanoic acid content across biological samples (e.g., plant vs. animal sources) impact extraction and quantification workflows?
- Methodology : Extraction efficiency varies with source material. For plant tissues (e.g., Moringa oleifera), use Soxhlet extraction with hexane to isolate hexadecanoic acid, achieving yields up to 20.8% in methanol extracts . For animal-derived samples (e.g., goat milk), saponification followed by methylation improves recovery. Validate methods using spike-and-recovery experiments with this compound to correct for losses during lipid hydrolysis .
Q. What advanced statistical approaches are recommended for analyzing fatty acid composition datasets involving this compound?
- Methodology : Multivariate analysis (e.g., PCA or PLS-DA) can identify correlations between Hexadecanoic acid levels and biological outcomes. For example, in goat milk studies, breed-specific variations (15.48–40.02% Hexadecanoic acid) require ANOVA with post-hoc Tukey tests to assess significance. Normalize data using deuterated internal standards to reduce technical variability .
Q. Methodological Best Practices
- Data Validation : Always include deuterated analogs (e.g., Hexadecanoic-d31) as internal standards in GC-MS workflows to correct for ion suppression or matrix effects .
- Contradiction Mitigation : Replicate experiments across independent labs using harmonized protocols (e.g., ISO/IEC 17025 guidelines) to address variability in biological activity reports .
- Ethical Reporting : Disclose isotopic purity (>98% for d31) and synthesis methods (e.g., catalytic deuteration) to ensure reproducibility in tracer studies .
Eigenschaften
IUPAC Name |
2,2,3,3,4,4,5,5,6,6,7,7,8,8,9,9,10,10,11,11,12,12,13,13,14,14,15,15,16,16,16-hentriacontadeuteriohexadecanoic acid | |
---|---|---|
Source | PubChem | |
URL | https://pubchem.ncbi.nlm.nih.gov | |
Description | Data deposited in or computed by PubChem | |
InChI |
InChI=1S/C16H32O2/c1-2-3-4-5-6-7-8-9-10-11-12-13-14-15-16(17)18/h2-15H2,1H3,(H,17,18)/i1D3,2D2,3D2,4D2,5D2,6D2,7D2,8D2,9D2,10D2,11D2,12D2,13D2,14D2,15D2 | |
Source | PubChem | |
URL | https://pubchem.ncbi.nlm.nih.gov | |
Description | Data deposited in or computed by PubChem | |
InChI Key |
IPCSVZSSVZVIGE-SAQPIRCFSA-N | |
Source | PubChem | |
URL | https://pubchem.ncbi.nlm.nih.gov | |
Description | Data deposited in or computed by PubChem | |
Canonical SMILES |
CCCCCCCCCCCCCCCC(=O)O | |
Source | PubChem | |
URL | https://pubchem.ncbi.nlm.nih.gov | |
Description | Data deposited in or computed by PubChem | |
Isomeric SMILES |
[2H]C([2H])([2H])C([2H])([2H])C([2H])([2H])C([2H])([2H])C([2H])([2H])C([2H])([2H])C([2H])([2H])C([2H])([2H])C([2H])([2H])C([2H])([2H])C([2H])([2H])C([2H])([2H])C([2H])([2H])C([2H])([2H])C([2H])([2H])C(=O)O | |
Source | PubChem | |
URL | https://pubchem.ncbi.nlm.nih.gov | |
Description | Data deposited in or computed by PubChem | |
Molecular Formula |
C16H32O2 | |
Source | PubChem | |
URL | https://pubchem.ncbi.nlm.nih.gov | |
Description | Data deposited in or computed by PubChem | |
DSSTOX Substance ID |
DTXSID10583617 | |
Record name | (~2~H_31_)Hexadecanoic acid | |
Source | EPA DSSTox | |
URL | https://comptox.epa.gov/dashboard/DTXSID10583617 | |
Description | DSSTox provides a high quality public chemistry resource for supporting improved predictive toxicology. | |
Molecular Weight |
287.61 g/mol | |
Source | PubChem | |
URL | https://pubchem.ncbi.nlm.nih.gov | |
Description | Data deposited in or computed by PubChem | |
CAS No. |
39756-30-4 | |
Record name | (~2~H_31_)Hexadecanoic acid | |
Source | EPA DSSTox | |
URL | https://comptox.epa.gov/dashboard/DTXSID10583617 | |
Description | DSSTox provides a high quality public chemistry resource for supporting improved predictive toxicology. | |
Record name | Palmitic d31 acid | |
Source | European Chemicals Agency (ECHA) | |
URL | https://echa.europa.eu/information-on-chemicals | |
Description | The European Chemicals Agency (ECHA) is an agency of the European Union which is the driving force among regulatory authorities in implementing the EU's groundbreaking chemicals legislation for the benefit of human health and the environment as well as for innovation and competitiveness. | |
Explanation | Use of the information, documents and data from the ECHA website is subject to the terms and conditions of this Legal Notice, and subject to other binding limitations provided for under applicable law, the information, documents and data made available on the ECHA website may be reproduced, distributed and/or used, totally or in part, for non-commercial purposes provided that ECHA is acknowledged as the source: "Source: European Chemicals Agency, http://echa.europa.eu/". Such acknowledgement must be included in each copy of the material. ECHA permits and encourages organisations and individuals to create links to the ECHA website under the following cumulative conditions: Links can only be made to webpages that provide a link to the Legal Notice page. | |
Retrosynthesis Analysis
AI-Powered Synthesis Planning: Our tool employs the Template_relevance Pistachio, Template_relevance Bkms_metabolic, Template_relevance Pistachio_ringbreaker, Template_relevance Reaxys, Template_relevance Reaxys_biocatalysis model, leveraging a vast database of chemical reactions to predict feasible synthetic routes.
One-Step Synthesis Focus: Specifically designed for one-step synthesis, it provides concise and direct routes for your target compounds, streamlining the synthesis process.
Accurate Predictions: Utilizing the extensive PISTACHIO, BKMS_METABOLIC, PISTACHIO_RINGBREAKER, REAXYS, REAXYS_BIOCATALYSIS database, our tool offers high-accuracy predictions, reflecting the latest in chemical research and data.
Strategy Settings
Precursor scoring | Relevance Heuristic |
---|---|
Min. plausibility | 0.01 |
Model | Template_relevance |
Template Set | Pistachio/Bkms_metabolic/Pistachio_ringbreaker/Reaxys/Reaxys_biocatalysis |
Top-N result to add to graph | 6 |
Feasible Synthetic Routes
Haftungsausschluss und Informationen zu In-Vitro-Forschungsprodukten
Bitte beachten Sie, dass alle Artikel und Produktinformationen, die auf BenchChem präsentiert werden, ausschließlich zu Informationszwecken bestimmt sind. Die auf BenchChem zum Kauf angebotenen Produkte sind speziell für In-vitro-Studien konzipiert, die außerhalb lebender Organismen durchgeführt werden. In-vitro-Studien, abgeleitet von dem lateinischen Begriff "in Glas", beinhalten Experimente, die in kontrollierten Laborumgebungen unter Verwendung von Zellen oder Geweben durchgeführt werden. Es ist wichtig zu beachten, dass diese Produkte nicht als Arzneimittel oder Medikamente eingestuft sind und keine Zulassung der FDA für die Vorbeugung, Behandlung oder Heilung von medizinischen Zuständen, Beschwerden oder Krankheiten erhalten haben. Wir müssen betonen, dass jede Form der körperlichen Einführung dieser Produkte in Menschen oder Tiere gesetzlich strikt untersagt ist. Es ist unerlässlich, sich an diese Richtlinien zu halten, um die Einhaltung rechtlicher und ethischer Standards in Forschung und Experiment zu gewährleisten.