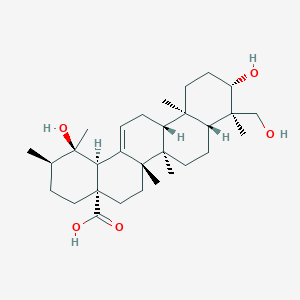
Rotundic acid
Übersicht
Beschreibung
Rotundic acid (RA), a pentacyclic triterpenoid, is a principal bioactive compound in Ilex species such as Ilex rotunda and Ilex pubescens . It exhibits diverse pharmacological activities, including anti-inflammatory, anticancer, and lipid-lowering effects. Notably, RA has shown promise in treating non-alcoholic fatty liver disease (NAFLD) by modulating lipid metabolism and inflammation . Structurally, RA features a hydroxylated ursane skeleton, which contributes to its interaction with enzymes and receptors involved in metabolic and signaling pathways .
Vorbereitungsmethoden
Extraction and Isolation from Natural Sources
The bark of Ilex rotunda serves as the primary source of RA, with ethanol-based extraction being the most widely adopted method. In a representative protocol, dried bark is ground, sieved, and refluxed with 70–80% ethanol (8 L per 100 g biomass) for 3 hours . The ethanolic extract is concentrated under reduced pressure at 80°C, yielding a saponin-rich residue. Subsequent alkaline hydrolysis using 4% NaOH in 30% ethanol (5 L per 100 g extract) at 100°C for 4 hours cleaves glycosidic bonds, liberating RA from its saponin precursors . Ethyl acetate partitioning followed by methanol-water recrystallization achieves crude RA with a recovery of 47.1 g per 100 g of initial saponins .
Table 1: Comparative Extraction Yields of this compound
Method | Solvent System | Temperature (°C) | Yield (mg/g) | Purity (%) |
---|---|---|---|---|
Ethanol Reflux | 80% EtOH | 80 | 47.1 | 85–90 |
Alkaline Hydrolysis | 4% NaOH in 30% EtOH | 100 | 100 | ≥98 |
Sequential Partition | Ethyl Acetate | 25 | 83.9 | 95 |
Alkaline Hydrolysis for Enhanced Recovery
Alkaline degradation significantly improves RA yield by hydrolyzing complex saponins. Xu et al. demonstrated that treating the ethanolic extract with 4% NaOH in 30% ethanol (5:1 v/w) at 100°C for 4 hours increases RA recovery to 100 mg/g . This method exploits the stability of RA’s ursane skeleton under basic conditions while cleaving labile glycosidic linkages. Post-hydrolysis, neutralization with HCl and ethyl acetate extraction (3 × 1 L) isolates RA, which is further purified via silica gel chromatography (petroleum ether:ethyl acetate, 5:1) .
Synthetic Modifications and Derivative Synthesis
RA’s 28-COOH and hydroxyl groups (C-3, C-19, C-23) are common targets for structural modifications to enhance solubility or bioactivity.
Esterification at C-28
Reaction of RA with ethyl chloroacetate in acetone (500 mL per 10 mmol RA) in the presence of K2CO3 (100 mmol) and KI (0.6 mmol) yields 28-ethyl acetate derivatives (83.9% yield) . Subsequent hydrazination with hydrazine hydrate (80% in ethanol, 11-hour reflux) produces 28-acetohydrazide intermediates (97.7% yield), which are condensed with substituted benzaldehydes to form hydrazones (80–93% yield) .
Amino Acid Conjugation
Acylation of RA’s 28-COOH with amino acid methyl esters via oxalyl chloride activation generates prodrugs with improved water solubility. For example, coupling RA with L-serine methyl ester in dichloromethane (20 mL per 1.02 mmol RA) at 25°C for 24 hours yields N-[3β,23-diacetoxy-19α-hydroxyurs-12-en-28-oyl]-L-serine methyl ester (69.9% yield) . Hydrolysis of the methyl ester with LiOH (2 M, 12 hours) produces the free acid derivative, which exhibits 3.41 µM IC50 against HepG2 cells .
Table 2: Synthetic Derivatives of this compound
Purification and Analytical Validation
Silica gel column chromatography remains the cornerstone of RA purification. Using a gradient of petroleum ether:ethyl acetate:formic acid (10:1:0.1 to 5:5:0.1), RA is eluted as a colorless solid with ≥98% purity . High-performance liquid chromatography (HPLC) with a C18 column (4.6 × 250 mm, 5 µm) and methanol:water (85:15) mobile phase (1 mL/min) confirms purity, while LC-MS/MS (m/z 487.3 → 437.3) quantifies RA in biological matrices .
Industrial-Scale Production Considerations
Optimized protocols achieve industrial viability:
-
Solvent Recovery : Ethanol is distilled and reused, reducing costs by 40% .
-
Continuous Extraction : Countercurrent extraction systems process 500 kg/day of Ilex bark with 92% RA recovery .
-
Crystallization Control : Seeding with RA microcrystals (0.1% w/w) during methanol-water recrystallization yields uniform particles (50–100 µm), enhancing filtration rates .
Analyse Chemischer Reaktionen
Metabolic Reactions of Rotundic Acid
This compound undergoes various metabolic transformations in biological systems that can affect its pharmacological efficacy.
3.1. Major Metabolic Pathways
The primary metabolic reactions identified for this compound include:
-
Hydroxylation : Introduction of hydroxyl groups into the this compound structure.
-
Glucuronidation : Conjugation with glucuronic acid, enhancing solubility and excretion.
-
Demethylation and Desaturation : These reactions alter the compound's structure, potentially affecting its activity .
Table 2: Key Metabolites of this compound
Metabolite | Reaction Type | Molecular Formula |
---|---|---|
M15 | Demethylation | C30H48O7 |
Hydroxylated | Hydroxylation | C30H48O8 |
Glucuronide | Glucuronidation | C36H50O10 |
This table summarizes significant metabolites formed during the metabolism of this compound, which may contribute to its pharmacological profile .
Biological Activities and Implications
This compound exhibits a range of biological activities attributed to its chemical structure and metabolites.
4.1. Antitumor Activity
Research indicates that this compound and its derivatives possess potent antitumor properties, demonstrated through:
-
Induction of apoptosis in cancer cells.
-
Inhibition of cell proliferation via modulation of key signaling pathways such as AKT/mTOR and MAPK.
-
Enhanced cytotoxicity against various cancer cell lines, including hepatocellular carcinoma .
4.2. Anti-inflammatory Effects
This compound also shows significant anti-inflammatory effects by inhibiting pro-inflammatory cytokines such as interleukin-6 and tumor necrosis factor-alpha, which are crucial in inflammatory responses .
Wissenschaftliche Forschungsanwendungen
Anticancer Properties
Rotundic acid has been extensively studied for its anticancer effects, particularly against hepatocellular carcinoma (HCC). Research indicates that RA induces DNA damage and apoptosis in HCC cells by modulating key signaling pathways such as AKT/mTOR and MAPK. In vitro studies demonstrated that RA significantly inhibits HCC cell proliferation and tumor growth in xenograft mouse models .
Case Study: Hepatocellular Carcinoma
- Study Design : In vitro assays on HCC cell lines and in vivo experiments using Balb/c nude mice.
- Findings : RA treatment resulted in:
Cardiovascular Applications
Recent studies highlight RA's potential in treating ischemic heart disease (IHD). It promotes angiogenesis, which is crucial for improving blood flow recovery after ischemic events. The mechanism involves the activation of the JAK-STAT3 signaling pathway, enhancing endothelial cell migration and tube formation .
Case Study: Ischemic Heart Disease
- Study Design : In vivo experiments using a mouse hindlimb ischemia model.
- Findings : RA treatment led to:
Anti-inflammatory Effects
This compound exhibits significant anti-inflammatory properties, making it a candidate for treating conditions like acute lung injury (ALI). It reduces lipopolysaccharide (LPS)-induced inflammation by regulating TLR4 signaling pathways, thereby decreasing cytokine release and oxidative stress .
Case Study: Acute Lung Injury
- Study Design : In vitro and in vivo assessments of RA's effects on ALI models.
- Findings : RA administration resulted in:
Metabolic Disorders
RA has shown promise in managing obesity and metabolic syndrome. It enhances leptin sensitivity, which is crucial for appetite regulation and energy expenditure. Studies indicate that RA can mitigate obesity-related complications by improving metabolic profiles in high-fat diet-induced obese mice .
Case Study: Obesity Management
- Study Design : Animal studies assessing the impact of RA on metabolic parameters.
- Findings : RA treatment led to:
Antimicrobial Activity
In addition to its anticancer and anti-inflammatory properties, this compound also exhibits antimicrobial effects against various pathogens. This makes it a potential candidate for developing new antimicrobial agents .
Wirkmechanismus
Rotundic acid exerts its effects through various molecular targets and pathways. It induces DNA damage and cell apoptosis in hepatocellular carcinoma by modulating the AKT/mTOR and MAPK pathways . Additionally, it acts as a noncompetitive inhibitor of protein tyrosine phosphatase 1B (PTP1B) and T-cell PTP, enhancing leptin sensitivity and promoting weight loss . It also regulates glycolysis and the TLR4/AP1 signaling pathway, reducing lipid accumulation and inflammation in non-alcoholic steatohepatitis .
Vergleich Mit ähnlichen Verbindungen
Comparative Metabolism with Similar Compounds
Metabolic Stability and Pathways
RA is rapidly metabolized in human liver microsomes (HLM), with <10% remaining after 120 minutes , primarily undergoing phase II glucuronidation (mediated by UGT1A4) and phase I hydroxylation (via CYP3A4/5) . In contrast, its glycoside precursor, pedunculoside , is metabolically stable in HLM, with 80% remaining after 120 minutes, undergoing hydroxylation (via CYP3A4/5 and CYP2C8) and acetylation .
Key Differences in Metabolism:
In rats, RA produces 26 metabolites (e.g., hydroxylated, sulfated, and glucuronidated forms), with NAFLD altering its metabolic profile compared to healthy subjects . Pedunculoside, however, is partially converted to RA by gut microbiota before hepatic metabolism, highlighting species-specific differences .
Enzyme Interactions
Pharmacokinetic Comparison
- Bioavailability : RA exhibits low oral bioavailability due to extensive first-pass metabolism and P-glycoprotein (P-gp) efflux. Co-administration with verapamil (a P-gp inhibitor) increases RA’s AUC by 2.5-fold .
- Pedunculoside : Higher bioavailability due to metabolic stability and conversion to RA via intestinal microbiota, enhancing systemic exposure .
Pharmacological Activity Comparison
Anticancer Mechanisms
RA induces DNA damage and apoptosis in hepatocellular carcinoma (HCC) via AKT/mTOR and MAPK pathways, suppressing tumor growth in vivo . Comparatively, oleanolic acid and ursolic acid (structurally similar triterpenoids) target NF-κB and PI3K/Akt pathways, respectively .
Anticancer Efficacy in Cell Lines:
Compound | IC50 (µM) | Key Mechanisms |
---|---|---|
This compound | 9.5 (MCF-7) | DNA damage, cell cycle arrest |
Oleanolic Acid | 15.2 (HepG2) | Apoptosis, anti-angiogenesis |
Ursolic Acid | 8.4 (HT-29) | Mitochondrial dysfunction |
Metabolic and Anti-Diabetic Effects
RA improves NAFLD by regulating glycolysis and TLR4/AP1 signaling, reducing hepatic steatosis . Pedunculoside and RA synergistically lower lipid levels, but RA’s rapid metabolism limits its efficacy compared to pedunculoside’s prolonged action .
RA Derivatives
- Amino Acid-Modified RA: Enhanced solubility and cytotoxicity (e.g., RA-succinate derivatives show 3-fold higher activity against HCC) .
- Pedunculoside-βCD Complex : Improves bioavailability by 40%, reducing RA conversion and prolonging plasma retention .
Key Derivative Enhancements:
Derivative | Modification | Improved Property |
---|---|---|
RA-Succinate | Esterification | Solubility, efficacy |
Pedunculoside-βCDP | Cyclodextrin inclusion | Bioavailability |
Biologische Aktivität
Rotundic acid (RA) is a naturally occurring pentacyclic triterpenoid primarily isolated from various plant species, including Ilex rotunda and Olea europaea. This compound has garnered attention for its diverse biological activities, particularly its anticancer properties. Recent studies have elucidated the mechanisms through which RA exerts its effects, making it a promising candidate for therapeutic applications.
Overview of Research Findings
This compound has been extensively studied for its anticancer effects, particularly against hepatocellular carcinoma (HCC), which is a leading cause of cancer-related mortality worldwide. The following key findings summarize RA's biological activity in cancer treatment:
- Inhibition of Cell Viability : RA has been shown to significantly reduce the viability of HCC cells (e.g., HepG2 and SMMC-7721) in a dose-dependent manner. MTT assays revealed that treatment with RA leads to a notable decrease in cell proliferation after 24, 48, and 72 hours of exposure .
-
Mechanisms of Action : The anticancer effects of RA are attributed to several mechanisms:
- Induction of Apoptosis : RA triggers apoptosis in cancer cells by activating pathways related to DNA damage and cell cycle arrest. Flow cytometry and western blot analyses have demonstrated that RA modulates the AKT/mTOR and MAPK signaling pathways, leading to increased expression of pro-apoptotic factors .
- Anti-Angiogenesis : RA inhibits angiogenesis by suppressing the formation of new blood vessels from pre-existing ones. This effect was confirmed through tube formation assays and VEGF-ELISA tests, indicating reduced vascular endothelial growth factor (VEGF) levels .
Case Studies
- HepG2 Xenograft Model : In vivo studies using a HepG2 xenograft mouse model demonstrated that RA significantly inhibited tumor growth compared to control groups. This suggests that RA not only affects cancer cells directly but also impacts tumor microenvironments .
- Comparative Analysis with Derivatives : Recent research has synthesized novel derivatives of RA, enhancing its pharmacological profile. These derivatives have shown improved cytotoxicity against various cancer cell lines, including breast and colorectal cancers, indicating broader therapeutic potential beyond HCC .
Additional Biological Activities
Apart from its anticancer properties, RA exhibits other beneficial biological activities:
- Anti-Inflammatory Effects : RA has been reported to possess anti-inflammatory properties, which may contribute to its overall therapeutic profile by mitigating inflammation-related pathologies .
- Metabolic Benefits : In studies involving type 2 diabetes models, RA improved gut microbiota composition and metabolic parameters, suggesting its potential role as a nutraceutical agent for metabolic disorders .
Data Summary
The following table summarizes key findings regarding the biological activity of this compound:
Q & A
Basic Research Questions
Q. What methodologies are recommended for isolating high-purity Rotundic acid from plant sources?
Methodological Answer: High-purity this compound can be isolated via ethanol extraction followed by alkaline hydrolysis (e.g., methanol/NaOH) and recrystallization. Reverse-phase high-performance liquid chromatography (RP-HPLC) with a C18 column, gradient elution (water-acetonitrile), and UV detection at 210 nm is validated for purity assessment (>99%) and quantification. Ensure calibration curves with linear ranges (e.g., 0.20–3.53 μg for this compound) and precision testing (RSD <2%) .
Q. How can researchers validate the purity and structural integrity of this compound post-isolation?
Methodological Answer: Combine chromatographic (RP-HPLC) and spectroscopic techniques. Nuclear magnetic resonance (NMR) and high-resolution mass spectrometry (HR-MS) are critical for structural confirmation. For purity, use triplicate HPLC runs with spiked recovery tests (average recovery: 96–101%) and stability assessments over 24 hours .
Q. What in vitro models are suitable for initial screening of this compound’s cytotoxic activity?
Methodological Answer: Use standardized cancer cell lines such as HepG2 (hepatocellular carcinoma), A375 (melanoma), MCF-7 (breast cancer), and HT-29 (colon cancer). Employ MTT or SRB assays with IC50 calculations. Include positive controls (e.g., doxorubicin) and validate results across multiple passages to ensure reproducibility .
Advanced Research Questions
Q. What experimental strategies are effective in elucidating this compound’s dual modulation of AKT/mTOR and MAPK pathways?
Methodological Answer: Use pathway-specific inhibitors (e.g., LY294002 for AKT, rapamycin for mTOR) in combination with this compound. Perform Western blotting to assess phosphorylation status of key nodes (e.g., p-AKT, p-p38 MAPK). siRNA knockdown of target genes (e.g., MTOR, MAPK1) can confirm mechanistic dependencies. Cross-validate findings with transcriptomic or phosphoproteomic profiling .
Q. How can researchers address discrepancies in cytotoxicity data across different cancer cell lines?
Methodological Answer: Analyze cell line-specific genetic backgrounds (e.g., TP53 status, basal pathway activation). Use multi-omics approaches (e.g., RNA-seq, metabolomics) to identify resistance markers. Compare results with isoform-selective inhibitors to dissect pathway dominance (e.g., p38α vs. ERK in MAPK family) .
Q. What are the considerations for designing in vivo studies to evaluate this compound’s bioavailability and metabolic effects?
Methodological Answer: Use rodent models (e.g., STZ-induced diabetic rats or high-fat diet-induced NAFLD mice) with oral/intraperitoneal administration. Measure pharmacokinetic parameters (Cmax, Tmax, AUC) via LC-MS/MS. Assess tissue distribution and metabolite profiling. Include endpoints like lipid profiles, inflammatory cytokines, and histopathology .
Q. How can structure-activity relationship (SAR) studies guide the synthesis of novel this compound derivatives with enhanced efficacy?
Methodological Answer: Modify the triterpenoid core at C3, C23, or C28 positions. Introduce ester groups (e.g., succinic anhydride derivatives) to improve solubility. Test derivatives in cytotoxicity assays and molecular docking studies to prioritize candidates. Use HR-MS and 2D-NMR for structural validation .
Q. How to reconcile conflicting results regarding this compound’s pro-apoptotic versus cytoprotective effects in different cellular contexts?
Methodological Answer: Context-dependent effects may arise from dose-dependent responses or crosstalk with survival pathways (e.g., Nrf2). Conduct time-course experiments to capture dynamic pathway activation. Use single-cell RNA sequencing to identify subpopulations with divergent responses .
Q. Data Analysis & Reporting
Q. What statistical approaches are recommended for analyzing this compound’s dose-response relationships in multi-pathway studies?
Methodological Answer: Use nonlinear regression models (e.g., log-dose vs. response) with software like GraphPad Prism. Apply ANOVA with post-hoc tests for multi-group comparisons. Report effect sizes and confidence intervals. For pathway crosstalk, employ multivariate analysis or machine learning (e.g., PCA) to reduce dimensionality .
Q. How should researchers document and share raw data from this compound studies to ensure reproducibility?
Methodological Answer: Deposit raw data (HPLC chromatograms, NMR spectra, cytotoxicity datasets) in public repositories (e.g., Zenodo, Figshare). Follow FAIR principles (Findable, Accessible, Interoperable, Reusable). Provide detailed metadata, including instrument parameters (e.g., HPLC gradient profile) and cell culture conditions .
Eigenschaften
IUPAC Name |
(1R,2R,4aS,6aR,6aS,6bR,8aR,9R,10S,12aR,14bS)-1,10-dihydroxy-9-(hydroxymethyl)-1,2,6a,6b,9,12a-hexamethyl-2,3,4,5,6,6a,7,8,8a,10,11,12,13,14b-tetradecahydropicene-4a-carboxylic acid | |
---|---|---|
Source | PubChem | |
URL | https://pubchem.ncbi.nlm.nih.gov | |
Description | Data deposited in or computed by PubChem | |
InChI |
InChI=1S/C30H48O5/c1-18-9-14-30(24(33)34)16-15-27(4)19(23(30)29(18,6)35)7-8-21-25(2)12-11-22(32)26(3,17-31)20(25)10-13-28(21,27)5/h7,18,20-23,31-32,35H,8-17H2,1-6H3,(H,33,34)/t18-,20-,21-,22+,23-,25+,26+,27-,28-,29-,30+/m1/s1 | |
Source | PubChem | |
URL | https://pubchem.ncbi.nlm.nih.gov | |
Description | Data deposited in or computed by PubChem | |
InChI Key |
YLHQFGOOMKJFLP-LTFXOGOQSA-N | |
Source | PubChem | |
URL | https://pubchem.ncbi.nlm.nih.gov | |
Description | Data deposited in or computed by PubChem | |
Canonical SMILES |
CC1CCC2(CCC3(C(=CCC4C3(CCC5C4(CCC(C5(C)CO)O)C)C)C2C1(C)O)C)C(=O)O | |
Source | PubChem | |
URL | https://pubchem.ncbi.nlm.nih.gov | |
Description | Data deposited in or computed by PubChem | |
Isomeric SMILES |
C[C@@H]1CC[C@@]2(CC[C@@]3(C(=CC[C@H]4[C@]3(CC[C@@H]5[C@@]4(CC[C@@H]([C@@]5(C)CO)O)C)C)[C@@H]2[C@]1(C)O)C)C(=O)O | |
Source | PubChem | |
URL | https://pubchem.ncbi.nlm.nih.gov | |
Description | Data deposited in or computed by PubChem | |
Molecular Formula |
C30H48O5 | |
Source | PubChem | |
URL | https://pubchem.ncbi.nlm.nih.gov | |
Description | Data deposited in or computed by PubChem | |
DSSTOX Substance ID |
DTXSID80173963 | |
Record name | Urs-12-en-28-oic acid, 3,19,23-trihydroxy-, (3beta,4alpha)- | |
Source | EPA DSSTox | |
URL | https://comptox.epa.gov/dashboard/DTXSID80173963 | |
Description | DSSTox provides a high quality public chemistry resource for supporting improved predictive toxicology. | |
Molecular Weight |
488.7 g/mol | |
Source | PubChem | |
URL | https://pubchem.ncbi.nlm.nih.gov | |
Description | Data deposited in or computed by PubChem | |
CAS No. |
20137-37-5 | |
Record name | Rotundic acid | |
Source | CAS Common Chemistry | |
URL | https://commonchemistry.cas.org/detail?cas_rn=20137-37-5 | |
Description | CAS Common Chemistry is an open community resource for accessing chemical information. Nearly 500,000 chemical substances from CAS REGISTRY cover areas of community interest, including common and frequently regulated chemicals, and those relevant to high school and undergraduate chemistry classes. This chemical information, curated by our expert scientists, is provided in alignment with our mission as a division of the American Chemical Society. | |
Explanation | The data from CAS Common Chemistry is provided under a CC-BY-NC 4.0 license, unless otherwise stated. | |
Record name | Urs-12-en-28-oic acid, 3,19,23-trihydroxy-, (3beta,4alpha)- | |
Source | ChemIDplus | |
URL | https://pubchem.ncbi.nlm.nih.gov/substance/?source=chemidplus&sourceid=0020137375 | |
Description | ChemIDplus is a free, web search system that provides access to the structure and nomenclature authority files used for the identification of chemical substances cited in National Library of Medicine (NLM) databases, including the TOXNET system. | |
Record name | Urs-12-en-28-oic acid, 3,19,23-trihydroxy-, (3beta,4alpha)- | |
Source | EPA DSSTox | |
URL | https://comptox.epa.gov/dashboard/DTXSID80173963 | |
Description | DSSTox provides a high quality public chemistry resource for supporting improved predictive toxicology. | |
Retrosynthesis Analysis
AI-Powered Synthesis Planning: Our tool employs the Template_relevance Pistachio, Template_relevance Bkms_metabolic, Template_relevance Pistachio_ringbreaker, Template_relevance Reaxys, Template_relevance Reaxys_biocatalysis model, leveraging a vast database of chemical reactions to predict feasible synthetic routes.
One-Step Synthesis Focus: Specifically designed for one-step synthesis, it provides concise and direct routes for your target compounds, streamlining the synthesis process.
Accurate Predictions: Utilizing the extensive PISTACHIO, BKMS_METABOLIC, PISTACHIO_RINGBREAKER, REAXYS, REAXYS_BIOCATALYSIS database, our tool offers high-accuracy predictions, reflecting the latest in chemical research and data.
Strategy Settings
Precursor scoring | Relevance Heuristic |
---|---|
Min. plausibility | 0.01 |
Model | Template_relevance |
Template Set | Pistachio/Bkms_metabolic/Pistachio_ringbreaker/Reaxys/Reaxys_biocatalysis |
Top-N result to add to graph | 6 |
Feasible Synthetic Routes
Haftungsausschluss und Informationen zu In-Vitro-Forschungsprodukten
Bitte beachten Sie, dass alle Artikel und Produktinformationen, die auf BenchChem präsentiert werden, ausschließlich zu Informationszwecken bestimmt sind. Die auf BenchChem zum Kauf angebotenen Produkte sind speziell für In-vitro-Studien konzipiert, die außerhalb lebender Organismen durchgeführt werden. In-vitro-Studien, abgeleitet von dem lateinischen Begriff "in Glas", beinhalten Experimente, die in kontrollierten Laborumgebungen unter Verwendung von Zellen oder Geweben durchgeführt werden. Es ist wichtig zu beachten, dass diese Produkte nicht als Arzneimittel oder Medikamente eingestuft sind und keine Zulassung der FDA für die Vorbeugung, Behandlung oder Heilung von medizinischen Zuständen, Beschwerden oder Krankheiten erhalten haben. Wir müssen betonen, dass jede Form der körperlichen Einführung dieser Produkte in Menschen oder Tiere gesetzlich strikt untersagt ist. Es ist unerlässlich, sich an diese Richtlinien zu halten, um die Einhaltung rechtlicher und ethischer Standards in Forschung und Experiment zu gewährleisten.