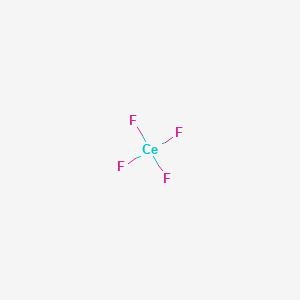
Ceriumfluorid (CeF4)
Übersicht
Beschreibung
Cerium fluoride (CeF4) is an inorganic compound comprised of the rare earth element cerium and the halogen fluoride. It is a white solid with a melting point of 1220°C and a density of 5.8 g/cm3. CeF4 has a variety of applications in scientific research, including as a fluorinating agent, an oxidizing agent, a catalyst, and a reagent in organic synthesis. In
Wissenschaftliche Forschungsanwendungen
Metallproduktion
CeF4 wird in der Metallproduktion eingesetzt, insbesondere in sauerstoffempfindlichen Anwendungen. Seine Rolle ist entscheidend aufgrund seiner wasserunlöslichen Natur, die es für Prozesse geeignet macht, bei denen Feuchtigkeit streng kontrolliert werden muss .
Ölraffination und Ätzen
Im Bereich der Ölraffination spielt CeF4 eine Rolle beim Ätzprozess. Das Ätzen ist für die Raffination von Metallen und die Vorbereitung von Oberflächen für die weitere Verarbeitung oder Veredelung unerlässlich .
Synthetische organische Chemie
CeF4 wird in der synthetischen organischen Chemie verwendet. Seine Eigenschaften sind vorteilhaft für verschiedene chemische Reaktionen und Prozesse, die das Rückgrat der organischen Synthese bilden .
Pharmazeutische Herstellung
Die Verbindung findet Anwendung bei der Herstellung von Arzneimitteln. Ihre chemischen Eigenschaften können bei der Produktion komplexer Arzneimittelverbindungen genutzt werden .
Glas- und Keramikindustrie
Ceriumfluorid wird in der Glas- und Keramikindustrie eingesetzt, insbesondere zum Polieren von Glas und in Keramiken als Katalysator und in Leuchtstoffen .
Katalyse
Es dient als Katalysator in verschiedenen chemischen Reaktionen, einschließlich der Hantzsch-Reaktion, die für die Synthese bestimmter organischer Verbindungen wichtig ist .
Mikroporöse Siliziumformation
CeF4 wird beim Ätzen von Flecken verwendet, um mikroporöses Silizium zu bilden, das Anwendungen in der Elektronik und Materialwissenschaft hat .
Fluorierungsreaktionen
Die Verbindung wird für Fluorierungsreaktionen verwendet, wie z. B. die Fluorierung von Kohlenwasserstoffen, ein kritischer Prozess in der organischen Chemie .
Wirkmechanismus
Target of Action
Cerium Fluoride (CeF4), also known as Tetrafluorocerium, is an inorganic compound . It primarily targets the fluorination process in various chemical reactions . It is also used as a catalyst in certain reactions .
Mode of Action
CeF4 is a strong oxidant . It can dissolve in DMSO and react to form the coordination complex [CeF4(DMSO)2] . This interaction with its targets leads to changes in the chemical structure of the compounds involved, enabling the progression of the reactions.
Biochemical Pathways
CeF4 is involved in the fluorination of various compounds . It is also used in the synthesis of sodium-rare earth silicate oxide-fluoride apatites . The exact biochemical pathways affected by CeF4 depend on the specific reactions it is involved in.
Pharmacokinetics
It’s important to note that cef4 is insoluble in water , which can impact its bioavailability in certain applications.
Result of Action
The primary result of CeF4’s action is the successful progression of the chemical reactions it is involved in. For example, it can facilitate the fluorination of fluorocyclobutene with high-valency metal fluoride . It can also be used for stain etching to form microporous silicon .
Action Environment
The action of CeF4 can be influenced by environmental factors. For instance, it is a strong oxidant and should be kept away from flammable materials to prevent fire accidents . It is also sensitive to moisture and should be stored in a dry environment . Its reactivity can be influenced by the temperature, as it can be produced by fluorinating cerium (III) fluoride or cerium dioxide with fluorine gas at 500 °C .
Safety and Hazards
Zukünftige Richtungen
Cerium (IV) fluoride has potential applications in various fields. For instance, it has been used to synthesize new cerium (IV) fluoride and fluoride–arsenate framework structures . It also has potential applications in the development of solid-state lasers, fiber optics , and could be of interest for pharmaceutical chemistry .
Biochemische Analyse
Biochemical Properties
Cerium fluoride is known to interact with various biomolecules. For instance, it can dissolve in DMSO and react to form the coordination complex
Molecular Mechanism
It is known to form a coordination complex when dissolved in DMSO , suggesting it may interact with biomolecules through binding interactions
Eigenschaften
IUPAC Name |
cerium(4+);tetrafluoride | |
---|---|---|
Details | Computed by LexiChem 2.6.6 (PubChem release 2019.06.18) | |
Source | PubChem | |
URL | https://pubchem.ncbi.nlm.nih.gov | |
Description | Data deposited in or computed by PubChem | |
InChI |
InChI=1S/Ce.4FH/h;4*1H/q+4;;;;/p-4 | |
Details | Computed by InChI 1.0.5 (PubChem release 2019.06.18) | |
Source | PubChem | |
URL | https://pubchem.ncbi.nlm.nih.gov | |
Description | Data deposited in or computed by PubChem | |
InChI Key |
SWURHZJFFJEBEE-UHFFFAOYSA-J | |
Details | Computed by InChI 1.0.5 (PubChem release 2019.06.18) | |
Source | PubChem | |
URL | https://pubchem.ncbi.nlm.nih.gov | |
Description | Data deposited in or computed by PubChem | |
Canonical SMILES |
[F-].[F-].[F-].[F-].[Ce+4] | |
Details | Computed by OEChem 2.1.5 (PubChem release 2019.06.18) | |
Source | PubChem | |
URL | https://pubchem.ncbi.nlm.nih.gov | |
Description | Data deposited in or computed by PubChem | |
Molecular Formula |
CeF4 | |
Details | Computed by PubChem 2.1 (PubChem release 2019.06.18) | |
Source | PubChem | |
URL | https://pubchem.ncbi.nlm.nih.gov | |
Description | Data deposited in or computed by PubChem | |
Molecular Weight |
216.110 g/mol | |
Details | Computed by PubChem 2.1 (PubChem release 2021.08.13) | |
Source | PubChem | |
URL | https://pubchem.ncbi.nlm.nih.gov | |
Description | Data deposited in or computed by PubChem | |
CAS No. |
10060-10-3 | |
Record name | Cerium fluoride (CeF4) | |
Source | CAS Common Chemistry | |
URL | https://commonchemistry.cas.org/detail?cas_rn=10060-10-3 | |
Description | CAS Common Chemistry is an open community resource for accessing chemical information. Nearly 500,000 chemical substances from CAS REGISTRY cover areas of community interest, including common and frequently regulated chemicals, and those relevant to high school and undergraduate chemistry classes. This chemical information, curated by our expert scientists, is provided in alignment with our mission as a division of the American Chemical Society. | |
Explanation | The data from CAS Common Chemistry is provided under a CC-BY-NC 4.0 license, unless otherwise stated. | |
Record name | CERIC FLUORIDE | |
Source | FDA Global Substance Registration System (GSRS) | |
URL | https://gsrs.ncats.nih.gov/ginas/app/beta/substances/A381976S11 | |
Description | The FDA Global Substance Registration System (GSRS) enables the efficient and accurate exchange of information on what substances are in regulated products. Instead of relying on names, which vary across regulatory domains, countries, and regions, the GSRS knowledge base makes it possible for substances to be defined by standardized, scientific descriptions. | |
Explanation | Unless otherwise noted, the contents of the FDA website (www.fda.gov), both text and graphics, are not copyrighted. They are in the public domain and may be republished, reprinted and otherwise used freely by anyone without the need to obtain permission from FDA. Credit to the U.S. Food and Drug Administration as the source is appreciated but not required. | |
Q1: How does cerium fluoride (CeF4) interact with Acid Blue 62 dye and what are the downstream effects?
A1: Cerium fluoride (CeF4) acts as a strong oxidizing agent. The research indicates that CeF4 effectively breaks down the aromatic ring structure of Acid Blue 62 dye []. This degradation process leads to decolorization of the dye solution and a reduction in chemical oxygen demand (COD), indicating a decrease in the overall organic load of the wastewater [].
Haftungsausschluss und Informationen zu In-Vitro-Forschungsprodukten
Bitte beachten Sie, dass alle Artikel und Produktinformationen, die auf BenchChem präsentiert werden, ausschließlich zu Informationszwecken bestimmt sind. Die auf BenchChem zum Kauf angebotenen Produkte sind speziell für In-vitro-Studien konzipiert, die außerhalb lebender Organismen durchgeführt werden. In-vitro-Studien, abgeleitet von dem lateinischen Begriff "in Glas", beinhalten Experimente, die in kontrollierten Laborumgebungen unter Verwendung von Zellen oder Geweben durchgeführt werden. Es ist wichtig zu beachten, dass diese Produkte nicht als Arzneimittel oder Medikamente eingestuft sind und keine Zulassung der FDA für die Vorbeugung, Behandlung oder Heilung von medizinischen Zuständen, Beschwerden oder Krankheiten erhalten haben. Wir müssen betonen, dass jede Form der körperlichen Einführung dieser Produkte in Menschen oder Tiere gesetzlich strikt untersagt ist. Es ist unerlässlich, sich an diese Richtlinien zu halten, um die Einhaltung rechtlicher und ethischer Standards in Forschung und Experiment zu gewährleisten.