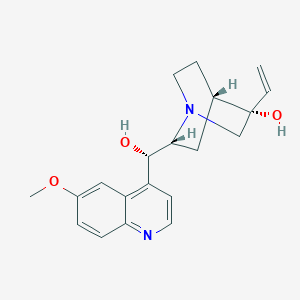
3-Hydroxyquinidine
Übersicht
Beschreibung
3-Hydroxyquinidine (C₂₀H₂₄N₂O₃), a major active metabolite of the antiarrhythmic drug quinidine, is formed via hydroxylation at the C3 position of the parent compound. Its stereochemistry has been confirmed as (3S)-3-hydroxyquinidine through X-ray crystallography and NMR analysis . Synthesized via microbial oxidation or chemical modification of quinidine, it shares structural similarities with quinidine but exhibits distinct pharmacokinetic and pharmacodynamic properties .
In vitro and clinical studies demonstrate that this compound retains antiarrhythmic activity, though with reduced potency compared to quinidine. It accumulates in plasma during chronic quinidine therapy, contributing to therapeutic and toxic effects . Approximately 60–70% of quinidine metabolism proceeds via the 3-hydroxylation pathway, primarily mediated by cytochrome P450 3A4 (CYP3A4) .
Vorbereitungsmethoden
Microbial Oxidation of Quinidine to 3-Hydroxyquinidine
Microbial oxidation represents a cornerstone in the biotechnological production of this compound, leveraging the metabolic capabilities of specific fungi and bacteria to hydroxylate quinidine at the C3 position. This method mimics mammalian metabolic pathways while offering advantages in selectivity and environmental sustainability .
Microorganisms and Metabolic Pathways
The biotransformation of quinidine to this compound has been documented in several fungal species:
-
Cunninghamella echinulata : This strain hydroxylates quinidine at the C3 position, producing this compound with a yield of 6–9% under optimized conditions .
-
Rhizopus arrhizus ATCC 10260 and 11145 : These strains selectively hydroxylate cinchona alkaloids, achieving comparable yields for structurally analogous compounds .
-
Xylaria digitata and Mycobacterium smegmatis : While primarily involved in N-oxidation, these species demonstrate secondary hydroxylation activity under substrate-limited conditions .
The reaction typically proceeds in nutrient-rich media at 25–30°C, with quinidine concentrations ranging from 0.5–2.0 g/L. Incubation periods span 72–120 hours, depending on microbial growth kinetics .
Table 1: Microbial Strains and Yields for this compound Production
Microorganism | Substrate Concentration (g/L) | Incubation Time (h) | Yield (%) |
---|---|---|---|
Cunninghamella echinulata | 1.5 | 96 | 8.5 |
Rhizopus arrhizus ATCC 10260 | 1.0 | 120 | 7.2 |
Mycobacterium smegmatis | 2.0 | 72 | 4.8 |
Optimization Strategies
Key parameters influencing yield include:
-
pH Control : Maintaining pH 6.5–7.0 enhances enzyme stability and substrate solubility .
-
Oxygenation : Increased dissolved oxygen (≥80% saturation) improves cytochrome P450 activity, critical for hydroxylation .
-
Co-Substrate Addition : Glucose (10–20 g/L) serves as a carbon source, while cyclodextrins enhance quinidine bioavailability via complexation .
Chemical Synthesis of this compound
Chemical synthesis provides an alternative route to this compound, particularly for laboratories lacking microbial fermentation infrastructure. This method involves regioselective hydroxylation of quinidine followed by purification and structural validation .
Regioselective Hydroxylation Protocols
A representative synthetic pathway involves:
-
Protection of the Quinidine Tertiary Amine : Treating quinidine with di-tert-butyl dicarbonate (Boc₂O) in dichloromethane to form the Boc-protected intermediate .
-
Hydroxylation at C3 : Using osmium tetroxide (OsO₄) as a catalyst and N-methylmorpholine N-oxide (NMO) as a co-oxidant in a tetrahydrofuran (THF)/water mixture .
-
Deprotection : Removal of the Boc group via trifluoroacetic acid (TFA) in dichloromethane, yielding this compound .
The reaction achieves a 65–70% yield after chromatographic purification (silica gel, ethyl acetate/methanol 9:1) .
Structural Validation
Synthetic this compound is characterized using:
-
X-Ray Crystallography : Single-crystal analysis of the methanesulfonate salt confirms the (3S) stereochemistry .
-
NMR Spectroscopy : and NMR spectra match microbial-derived samples, with key signals at δ 5.87 (H-3) and δ 79.2 (C-3) .
Enzymatic Hydroxylation via CYP3A4
The cytochrome P450 enzyme CYP3A4 catalyzes the (3S)-hydroxylation of quinidine in human hepatic systems, offering a platform for in vitro enzymatic synthesis .
Kinetic Parameters and Reaction Conditions
Studies in human liver microsomes reveal Michaelis-Menten kinetics for this compound formation:
{\text{max}} = 74.4 \, \text{nmol/mg/h}, \quad Km = 74.2 \, \mu\text{M}
The reaction proceeds optimally at pH 7.4 and 37°C, with NADPH (1 mM) as a cofactor .
Table 2: Kinetic Parameters of CYP3A4-Mediated Hydroxylation
Parameter | Value |
---|---|
74.4 ± 3.2 nmol/mg/h | |
74.2 ± 5.1 µM | |
12.1 ± 0.8 min⁻¹ |
Recombinant CYP3A4 Systems
Recombinant CYP3A4 expressed in Saccharomyces cerevisiae achieves a 4.8-fold higher compared to human liver microsomes, enabling scalable production .
Comparative Analysis of Preparation Methods
Yield and Scalability
-
Microbial Oxidation : Yields (4.8–9%) suit small-scale production but require prolonged incubation .
-
Chemical Synthesis : Higher yields (65–70%) but involve toxic reagents (OsO₄) .
-
Enzymatic Hydroxylation : Theoretically scalable with recombinant enzymes, though costs remain prohibitive for industrial use .
Analyse Chemischer Reaktionen
3-Hydroxyquinidine undergoes various chemical reactions, including oxidation, reduction, and substitution reactions. Common reagents used in these reactions include oxidizing agents like potassium permanganate and reducing agents like sodium borohydride. The major products formed from these reactions depend on the specific conditions and reagents used. For example, oxidation of this compound can yield quinidine-N-oxide, while reduction can produce dihydroquinidine .
Wissenschaftliche Forschungsanwendungen
Pharmacokinetics and Pharmacodynamics
3-Hydroxyquinidine is recognized for its pharmacologically active properties, influencing the therapeutic effects of quinidine. Studies show that the pharmacokinetic profile of this compound differs significantly from that of its parent compound, quinidine.
- Serum Concentrations : Research indicates that at steady state, the area under the serum concentration vs. time curve (AUC) for this compound is higher than after a single dose (2.0 ± 0.7 vs. 3.0 ± 0.6 mg l⁻¹ h) . This suggests that repeated dosing enhances the metabolite's bioavailability.
- Clearance Rates : The renal clearance of this compound is notably less than that of quinidine (1.54 ± 0.38 ml min⁻¹ kg⁻¹ vs. 3.0 ± 1.1 ml min⁻¹ kg⁻¹), indicating a longer half-life and sustained activity in the body .
Drug Interactions
This compound plays a crucial role in drug-drug interactions (DDIs), particularly as an inhibitor of cytochrome P450 enzymes.
- CYP2D6 Inhibition : It has been established that both quinidine and its metabolite inhibit CYP2D6, which is essential for the metabolism of many drugs . This inhibition can lead to increased plasma concentrations of co-administered medications metabolized by this pathway.
- Clinical Implications : The metabolite's interaction with other drugs has been modeled using physiologically-based pharmacokinetic (PBPK) approaches, allowing researchers to predict complex DDIs effectively . For instance, the interaction between quinidine and metoprolol has been highlighted as a significant clinical concern due to altered drug levels.
Therapeutic Uses
The therapeutic applications of this compound are primarily linked to its effects on cardiac function and arrhythmia management.
- Antiarrhythmic Properties : As an active metabolite, this compound contributes to the antiarrhythmic effects observed with quinidine treatment . Its efficacy in managing atrial fibrillation and ventricular tachycardia has been documented.
- Case Studies : In clinical evaluations involving patients treated with quinidine, it was noted that concentrations of this compound often exceed those of quinidine itself in certain individuals, suggesting a significant contribution to overall therapeutic outcomes .
Research Findings and Insights
Recent studies have provided valuable insights into the behavior and effects of this compound:
- Concentration Ratios : A study found that the average ratio of this compound to quinidine in serum was approximately 0.34 ± 0.17, with some patients exhibiting ratios greater than 0.50, indicating variability in individual responses to treatment .
- Age-related Clearance : Research indicates that the clearance rates for both quinidine and its metabolite decrease with age, necessitating careful dosage adjustments in older populations .
Summary Table of Key Findings
Parameter | Quinidine | This compound |
---|---|---|
AUC (mg l⁻¹ h) | 2.0 ± 0.7 (single dose) | 3.0 ± 0.6 (steady state) |
Renal Clearance (ml min⁻¹ kg⁻¹) | 3.0 ± 1.1 | 1.54 ± 0.38 |
Average Serum Ratio | - | 0.34 ± 0.17 |
Inhibition of CYP2D6 | Yes | Yes |
Wirkmechanismus
3-Hydroxyquinidine exerts its effects by blocking sodium and potassium currents in cardiac cells, similar to quinidine. This action prolongs the cellular action potential and reduces myocardial excitability and conduction velocity. The compound also affects calcium transport across cell membranes, contributing to its antiarrhythmic properties . The molecular targets of this compound include voltage-gated sodium channels and potassium channels, which are critical for maintaining normal cardiac rhythm .
Vergleich Mit ähnlichen Verbindungen
Structural and Metabolic Comparisons
Pharmacodynamic Differences
- Causes early afterdepolarizations in canine Purkinje fibers at high concentrations . Additive antiarrhythmic effects when combined with quinidine .
- Quinidine-N-oxide: No significant effect on cardiac transmembrane action potentials or QT intervals .
Dihydroquinidine :
Pharmacokinetic Profiles
This compound :
- O-Desmethylquinidine: Exhibits nonlinear kinetics due to saturation of conjugation pathways .
Quinidine-N-oxide :
Enzyme Interactions
CYP3A4 :
CYP2D6 :
Clinical and Analytical Considerations
Toxicity Monitoring :
Drug-Drug Interactions :
- Quinidine’s inhibition of CYP2D6 increases dextromethorphan levels (e.g., in Nuedexta®), while CYP3A4 inducers (e.g., rifampin) reduce this compound exposure .
Biologische Aktivität
3-Hydroxyquinidine (3-OH-Q) is a significant metabolite of quinidine, a well-known antiarrhythmic agent. This compound has garnered attention due to its pharmacological activity and its role in the metabolic pathways of quinidine. This article explores the biological activity of this compound, highlighting its pharmacodynamics, metabolic pathways, and clinical implications.
Metabolism and Formation
This compound is primarily formed through the (3S)-3-hydroxylation of quinidine, which is catalyzed by the cytochrome P450 enzyme CYP3A4. In studies involving human liver microsomes, the formation of 3-OH-Q demonstrated Michaelis-Menten kinetics with a maximum velocity () of 74.4 nmol/mg/h and a Michaelis constant () of 74.2 µM . The specificity of CYP3A4 for this reaction makes it a reliable biomarker for assessing CYP3A4 activity in clinical settings.
Pharmacodynamics
The pharmacological effects of this compound have been studied in various contexts, particularly concerning its impact on cardiac function:
- QT Interval Prolongation : A randomized double-blind study assessed the relationship between serum concentrations of 3-OH-Q and QT interval prolongation in healthy subjects. The results indicated a statistically significant correlation between serum levels of both quinidine and 3-OH-Q with QTc prolongation . The mean slope for 3-OH-Q was found to be , indicating its potential role in cardiac electrophysiology.
- Combined Administration : When administered alongside quinidine, 3-OH-Q exhibited an additive effect on QTc prolongation, suggesting that it contributes to the overall pharmacodynamic profile of quinidine .
Clinical Case Studies
Several clinical studies have highlighted the importance of monitoring both quinidine and its metabolite, this compound, in therapeutic settings:
- Study on Concentrations : A study involving 25 patients receiving quinidine showed that at peak concentrations, unbound levels of 3-OH-Q frequently exceeded those of quinidine. This finding underscores the significance of considering both metabolites when evaluating therapeutic efficacy and safety .
- Brugada Syndrome Treatment : In patients with Brugada syndrome, hydroquinidine (HQ), which can be metabolized to 3-OH-Q, was effective in reducing ventricular arrhythmias during electrophysiological studies. The treatment led to a significant reduction in arrhythmia inducibility and symptoms associated with the condition .
Table: Summary of Key Pharmacokinetic Parameters
Parameter | Value |
---|---|
(3-OH-Q formation) | 74.4 nmol/mg/h |
(3-OH-Q formation) | 74.2 µM |
Peak concentration (3-OH-Q) | 1362 - 3480 ng/ml |
Free fraction (3-OH-Q) | 49% ± 4.8 |
Free fraction (quinidine) | 20% ± 4.3 |
Q & A
Q. Basic: What are the primary metabolic pathways of 3-Hydroxyquinidine in hepatic systems, and how are they quantitatively assessed?
Methodological Answer:
this compound is a major metabolite of quinidine, primarily formed via CYP3A4-mediated oxidation . To assess its metabolic pathways, researchers employ in vitro liver models (e.g., liver-on-a-chip devices) combined with kinetic modeling. Key steps include:
- Experimental Design : Measure parent drug (quinidine) and metabolite (this compound) concentration-time profiles under controlled conditions. Use hepatocyte systems with intact metabolic enzymes for physiological relevance .
- Quantitative Analysis : Estimate intrinsic clearance (CLint) and fraction metabolized (ƒm) using compartmental models. For example, Model 3 (which includes this compound formation and additional pathways like quinidine N-oxide production) showed ƒm values of 0.64–0.71 for this compound, validated via Bayesian Information Criterion (BIC) comparisons .
Q. Advanced: How can researchers resolve discrepancies between in silico models predicting this compound metabolism kinetics?
Methodological Answer:
Discrepancies often arise from incomplete pathway characterization. For example:
- Model Comparison : Four models were tested in quinidine metabolism studies. Model 1 (single pathway) underperformed (BIC = 120), while Models 2–4 (multiple pathways) showed better fit (BIC = 85–90) .
- Resolution Strategies :
- Extended Incubation : Prolong experiments beyond 24 hours to capture delayed metabolite formation (e.g., quinidine N-oxide) .
- Supplementary Data : Quantify secondary metabolites (e.g., via LC-MS/MS) to validate competing pathways .
- Uncertainty Analysis : Use structural identifiability studies to assess parameter confidence intervals (e.g., ~50% uncertainty in CLint for this compound in Model 4) .
Q. Basic: What analytical techniques are recommended for quantifying this compound in in vitro systems?
Methodological Answer:
- Chromatography : High-performance liquid chromatography (HPLC) with UV detection or liquid chromatography-tandem mass spectrometry (LC-MS/MS) for high sensitivity .
- Validation : Include calibration curves (linear range: 0.1–100 µM), inter-day/intra-day precision (<15% RSD), and recovery studies (>80%) to ensure reproducibility .
- Data Reporting : Follow Beilstein Journal guidelines: report raw data in appendices, processed data in main text, and cite instrument parameters (e.g., column type, mobile phase) .
Q. Advanced: What experimental designs optimize the identification of secondary metabolites in this compound metabolism studies?
Methodological Answer:
- Time-Course Experiments : Collect samples at multiple time points (e.g., 0, 6, 12, 24, 48 hours) to capture slow-forming metabolites .
- Enzyme Inhibition : Use selective CYP inhibitors (e.g., ketoconazole for CYP3A4) to isolate pathways and confirm metabolite origins .
- Multi-Omics Integration : Combine metabolomics (untargeted LC-MS) with proteomics to correlate metabolite profiles with enzyme expression levels .
Q. Basic: How does this compound interact with cytochrome P450 enzymes, and what implications does this have for drug interaction studies?
Methodological Answer:
- Enzyme Inhibition : this compound inhibits CYP2D6, as shown by mutant studies (e.g., F120A CYP2D6 variant catalyzes this compound formation) .
- Study Design :
Q. Advanced: What statistical approaches are most effective for analyzing variability in this compound metabolic data across different hepatic models?
Methodological Answer:
- Hierarchical Bayesian Modeling : Account for inter-individual variability by pooling data across models (e.g., liver-on-a-chip vs. microsomes) to estimate population-level CLint .
- Sensitivity Analysis : Identify parameters (e.g., enzyme Vmax) contributing most to variability using Monte Carlo simulations .
- Ethical Reporting : Disclose raw data and computational scripts in supplementary materials to enable reproducibility .
Q. Basic: How should researchers validate the identity and purity of newly synthesized this compound in pharmacological studies?
Methodological Answer:
- Spectroscopic Characterization : Use NMR (<sup>1</sup>H, <sup>13</sup>C) and high-resolution MS to confirm molecular structure .
- Purity Assessment : Report HPLC chromatograms (purity >95%) and elemental analysis (C, H, N within ±0.4% of theoretical) .
- Literature Cross-Referencing : Compare spectral data with published values for quinidine metabolites .
Q. Advanced: How can physiologically based pharmacokinetic (PBPK) modeling improve predictions of this compound disposition in humans?
Methodological Answer:
- Model Parameterization : Integrate in vitro CLint and ƒm values (from liver-on-a-chip data) with human physiological parameters (e.g., hepatic blood flow) .
- Validation : Compare simulated plasma concentrations with clinical data from quinidine trials, adjusting for renal excretion of this compound .
- Regulatory Compliance : Follow EMA/FDA guidelines for PBPK reporting, including sensitivity analysis and uncertainty quantification .
Eigenschaften
IUPAC Name |
(3S,4S,6R)-3-ethenyl-6-[(S)-hydroxy-(6-methoxyquinolin-4-yl)methyl]-1-azabicyclo[2.2.2]octan-3-ol | |
---|---|---|
Source | PubChem | |
URL | https://pubchem.ncbi.nlm.nih.gov | |
Description | Data deposited in or computed by PubChem | |
InChI |
InChI=1S/C20H24N2O3/c1-3-20(24)12-22-9-7-13(20)10-18(22)19(23)15-6-8-21-17-5-4-14(25-2)11-16(15)17/h3-6,8,11,13,18-19,23-24H,1,7,9-10,12H2,2H3/t13-,18+,19-,20+/m0/s1 | |
Source | PubChem | |
URL | https://pubchem.ncbi.nlm.nih.gov | |
Description | Data deposited in or computed by PubChem | |
InChI Key |
BSRUJCFCZKMFMB-LGWHJFRWSA-N | |
Source | PubChem | |
URL | https://pubchem.ncbi.nlm.nih.gov | |
Description | Data deposited in or computed by PubChem | |
Canonical SMILES |
COC1=CC2=C(C=CN=C2C=C1)C(C3CC4CCN3CC4(C=C)O)O | |
Source | PubChem | |
URL | https://pubchem.ncbi.nlm.nih.gov | |
Description | Data deposited in or computed by PubChem | |
Isomeric SMILES |
COC1=CC2=C(C=CN=C2C=C1)[C@@H]([C@H]3C[C@@H]4CCN3C[C@@]4(C=C)O)O | |
Source | PubChem | |
URL | https://pubchem.ncbi.nlm.nih.gov | |
Description | Data deposited in or computed by PubChem | |
Molecular Formula |
C20H24N2O3 | |
Source | PubChem | |
URL | https://pubchem.ncbi.nlm.nih.gov | |
Description | Data deposited in or computed by PubChem | |
DSSTOX Substance ID |
DTXSID601024742 | |
Record name | (3S)-hydroxyquinidine | |
Source | EPA DSSTox | |
URL | https://comptox.epa.gov/dashboard/DTXSID601024742 | |
Description | DSSTox provides a high quality public chemistry resource for supporting improved predictive toxicology. | |
Molecular Weight |
340.4 g/mol | |
Source | PubChem | |
URL | https://pubchem.ncbi.nlm.nih.gov | |
Description | Data deposited in or computed by PubChem | |
CAS No. |
53467-23-5 | |
Record name | (9S)-6′-Methoxycinchonan-3,9-diol | |
Source | CAS Common Chemistry | |
URL | https://commonchemistry.cas.org/detail?cas_rn=53467-23-5 | |
Description | CAS Common Chemistry is an open community resource for accessing chemical information. Nearly 500,000 chemical substances from CAS REGISTRY cover areas of community interest, including common and frequently regulated chemicals, and those relevant to high school and undergraduate chemistry classes. This chemical information, curated by our expert scientists, is provided in alignment with our mission as a division of the American Chemical Society. | |
Explanation | The data from CAS Common Chemistry is provided under a CC-BY-NC 4.0 license, unless otherwise stated. | |
Record name | 3-Hydroxyquinidine | |
Source | ChemIDplus | |
URL | https://pubchem.ncbi.nlm.nih.gov/substance/?source=chemidplus&sourceid=0053467235 | |
Description | ChemIDplus is a free, web search system that provides access to the structure and nomenclature authority files used for the identification of chemical substances cited in National Library of Medicine (NLM) databases, including the TOXNET system. | |
Record name | (3S)-hydroxyquinidine | |
Source | EPA DSSTox | |
URL | https://comptox.epa.gov/dashboard/DTXSID601024742 | |
Description | DSSTox provides a high quality public chemistry resource for supporting improved predictive toxicology. | |
Record name | 3-HYDROXYQUINIDINE | |
Source | FDA Global Substance Registration System (GSRS) | |
URL | https://gsrs.ncats.nih.gov/ginas/app/beta/substances/00G939C83O | |
Description | The FDA Global Substance Registration System (GSRS) enables the efficient and accurate exchange of information on what substances are in regulated products. Instead of relying on names, which vary across regulatory domains, countries, and regions, the GSRS knowledge base makes it possible for substances to be defined by standardized, scientific descriptions. | |
Explanation | Unless otherwise noted, the contents of the FDA website (www.fda.gov), both text and graphics, are not copyrighted. They are in the public domain and may be republished, reprinted and otherwise used freely by anyone without the need to obtain permission from FDA. Credit to the U.S. Food and Drug Administration as the source is appreciated but not required. | |
Retrosynthesis Analysis
AI-Powered Synthesis Planning: Our tool employs the Template_relevance Pistachio, Template_relevance Bkms_metabolic, Template_relevance Pistachio_ringbreaker, Template_relevance Reaxys, Template_relevance Reaxys_biocatalysis model, leveraging a vast database of chemical reactions to predict feasible synthetic routes.
One-Step Synthesis Focus: Specifically designed for one-step synthesis, it provides concise and direct routes for your target compounds, streamlining the synthesis process.
Accurate Predictions: Utilizing the extensive PISTACHIO, BKMS_METABOLIC, PISTACHIO_RINGBREAKER, REAXYS, REAXYS_BIOCATALYSIS database, our tool offers high-accuracy predictions, reflecting the latest in chemical research and data.
Strategy Settings
Precursor scoring | Relevance Heuristic |
---|---|
Min. plausibility | 0.01 |
Model | Template_relevance |
Template Set | Pistachio/Bkms_metabolic/Pistachio_ringbreaker/Reaxys/Reaxys_biocatalysis |
Top-N result to add to graph | 6 |
Feasible Synthetic Routes
Haftungsausschluss und Informationen zu In-Vitro-Forschungsprodukten
Bitte beachten Sie, dass alle Artikel und Produktinformationen, die auf BenchChem präsentiert werden, ausschließlich zu Informationszwecken bestimmt sind. Die auf BenchChem zum Kauf angebotenen Produkte sind speziell für In-vitro-Studien konzipiert, die außerhalb lebender Organismen durchgeführt werden. In-vitro-Studien, abgeleitet von dem lateinischen Begriff "in Glas", beinhalten Experimente, die in kontrollierten Laborumgebungen unter Verwendung von Zellen oder Geweben durchgeführt werden. Es ist wichtig zu beachten, dass diese Produkte nicht als Arzneimittel oder Medikamente eingestuft sind und keine Zulassung der FDA für die Vorbeugung, Behandlung oder Heilung von medizinischen Zuständen, Beschwerden oder Krankheiten erhalten haben. Wir müssen betonen, dass jede Form der körperlichen Einführung dieser Produkte in Menschen oder Tiere gesetzlich strikt untersagt ist. Es ist unerlässlich, sich an diese Richtlinien zu halten, um die Einhaltung rechtlicher und ethischer Standards in Forschung und Experiment zu gewährleisten.