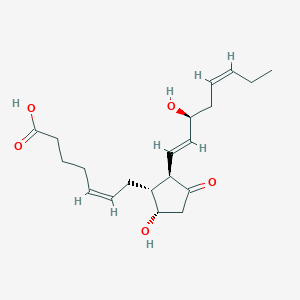
Prostaglandin D3
Übersicht
Beschreibung
Prostaglandin D3 ist ein Mitglied der Prostaglandin-Familie, die Lipide aus Fettsäuren sind. This compound ist bekannt für seine Rolle als Hemmstoff der Thrombozytenaggregation und als Modulator der autonomen Neurotransmission beim Menschen . Es hat die Summenformel C20H30O5 und ein Molekulargewicht von 350,45 g/mol .
Vorbereitungsmethoden
Synthesewege und Reaktionsbedingungen
Prostaglandin D3 kann durch verschiedene chemische Verfahren synthetisiert werden. Ein gängiges Verfahren beinhaltet die Oxidation von Eicosapentaensäure (EPA) unter Verwendung spezifischer Oxidationsmittel unter kontrollierten Bedingungen. Die Reaktion erfordert typischerweise einen Katalysator und verläuft über mehrere Zwischenschritte, einschließlich der Bildung von Hydroperoxiden und der anschließenden Reduktion, um die endgültige Prostaglandinstruktur zu erhalten .
Industrielle Produktionsmethoden
Die industrielle Produktion von this compound erfolgt häufig in großem Maßstab unter Verwendung ähnlicher chemischer Verfahren wie im Labor, die jedoch für höhere Ausbeuten und Reinheit optimiert sind. Dazu kann die Verwendung fortschrittlicher katalytischer Systeme und kontinuierlicher Durchflussreaktoren gehören, um eine konstante Produktionsqualität und Effizienz zu gewährleisten .
Chemische Reaktionsanalyse
Arten von Reaktionen
This compound durchläuft verschiedene chemische Reaktionen, darunter:
Oxidation: this compound kann oxidiert werden, um verschiedene Derivate zu bilden, die unterschiedliche biologische Aktivitäten haben können.
Reduktion: Reduktionsreaktionen können die funktionellen Gruppen am Prostaglandinmolekül modifizieren und so seine Eigenschaften verändern.
Häufige Reagenzien und Bedingungen
Häufig verwendete Reagenzien in diesen Reaktionen umfassen Oxidationsmittel wie Wasserstoffperoxid, Reduktionsmittel wie Natriumborhydrid und verschiedene Katalysatoren, um die Reaktionen zu ermöglichen. Reaktionsbedingungen umfassen typischerweise kontrollierte Temperaturen und pH-Werte, um die gewünschten Transformationen zu gewährleisten .
Hauptprodukte, die gebildet werden
Die Hauptprodukte, die aus diesen Reaktionen gebildet werden, umfassen verschiedene Prostaglandinderivate mit modifizierten funktionellen Gruppen, die unterschiedliche biologische Aktivitäten und therapeutische Potentiale aufweisen können .
Analyse Chemischer Reaktionen
Types of Reactions
Prostaglandin D3 undergoes various chemical reactions, including:
Oxidation: this compound can be oxidized to form different derivatives, which may have distinct biological activities.
Reduction: Reduction reactions can modify the functional groups on the prostaglandin molecule, altering its properties.
Common Reagents and Conditions
Common reagents used in these reactions include oxidizing agents like hydrogen peroxide, reducing agents such as sodium borohydride, and various catalysts to facilitate the reactions. Reaction conditions typically involve controlled temperatures and pH levels to ensure the desired transformations .
Major Products Formed
The major products formed from these reactions include various prostaglandin derivatives with modified functional groups, which can exhibit different biological activities and therapeutic potentials .
Wissenschaftliche Forschungsanwendungen
Antithrombotic Properties
PGD3 has been identified as a potential antithrombotic agent. Research indicates that PGD3 can inhibit platelet aggregation, which is a crucial factor in thrombus formation. In studies involving human platelet-rich plasma (PRP), pretreatment with PGD3 resulted in increased cyclic AMP concentrations, leading to inhibited platelet aggregation. This suggests that PGD3 could be utilized in preventing thrombotic events, particularly in patients at risk for cardiovascular diseases .
Table 1: Effects of PGD3 on Platelet Aggregation
Study | Method | Findings |
---|---|---|
Human PRP | PGD3 inhibits platelet aggregation by increasing cAMP levels. | |
In vitro | PGD3 shows equipotent effects to PGD2 in reducing systemic blood pressure. |
Modulation of Inflammatory Responses
PGD3 plays a role in modulating inflammatory responses, particularly through its interaction with immune cells. It has been shown to influence the production of other prostaglandins and cytokines, which are pivotal in inflammation and immune regulation. For instance, vitamin D3 metabolites have been found to stimulate the production of prostaglandins, including PGD3, within human fetal membranes and placenta, suggesting a paracrine role in modulating inflammatory processes during pregnancy .
Case Study: Vitamin D and Prostaglandin Production
In a study examining the effects of vitamin D metabolites on prostaglandin production, researchers observed that treatment with 25-hydroxyvitamin D and 1,25-dihydroxyvitamin D led to increased levels of PGE2 and PGF2α in human placental cells. This indicates that vitamin D may enhance prostaglandin synthesis via pathways involving PGD3, potentially influencing pregnancy outcomes .
Pain Management
Recent studies have indicated that prostaglandins play significant roles in pain perception and management. PGD3's ability to modulate pain pathways makes it a candidate for therapeutic interventions in pain management. For instance, vitamin D supplementation has been linked to decreased levels of inflammatory prostaglandins, including PGD3, suggesting that it may help alleviate pain associated with inflammatory conditions .
Table 2: Role of Prostaglandins in Pain Management
Potential Role in Cancer Prevention
Emerging evidence suggests that PGD3 may have a protective role against certain cancers by modulating the inflammatory environment and reducing levels of pro-carcinogenic prostaglandins like PGE2. A study indicated that women with higher vitamin D levels had significantly lower odds of developing breast cancer, potentially due to the interplay between vitamin D metabolites and prostaglandin synthesis .
Case Study: Vitamin D Levels and Breast Cancer Risk
A clinical trial involving women showed that those receiving higher doses of vitamin D experienced reduced levels of PGE2, which is associated with cancer progression. This highlights the potential for using vitamin D to influence PGD3 levels as part of cancer prevention strategies .
Wirkmechanismus
Prostaglandin D3 exerts its effects by binding to specific prostaglandin receptors on the surface of target cells. This binding triggers a cascade of intracellular signaling pathways that modulate various physiological processes, including platelet aggregation and neurotransmission. The molecular targets involved include G-protein-coupled receptors and downstream signaling molecules such as cyclic AMP (cAMP) and protein kinases .
Vergleich Mit ähnlichen Verbindungen
Prostaglandin D3 kann mit anderen Prostaglandinen wie Prostaglandin D2 und Prostaglandin E2 verglichen werden. Während all diese Verbindungen eine ähnliche Kernstruktur aufweisen, unterscheiden sie sich in ihren funktionellen Gruppen und biologischen Aktivitäten. This compound ist einzigartig in seiner spezifischen Hemmung der Thrombozytenaggregation und Modulation der autonomen Neurotransmission, was es von anderen Prostaglandinen unterscheidet .
Liste ähnlicher Verbindungen
- Prostaglandin D2
- Prostaglandin E2
- Prostaglandin F2α
- Prostaglandin I2 (Prostacyclin)
- Thromboxan A2
Biologische Aktivität
Prostaglandin D3 (PGD3) is a member of the prostaglandin family, which are lipid compounds derived from fatty acids that play crucial roles in various physiological processes. PGD3 is particularly interesting due to its potential biological activities, including anti-inflammatory effects and modulation of platelet aggregation. This article explores the biological activity of PGD3, supported by research findings, case studies, and data tables.
Overview of this compound
PGD3 is synthesized from icosapentaenoic acid (EPA), an omega-3 fatty acid found in fish oils. Its formation occurs through enzymatic processes involving cyclooxygenase enzymes (COX). PGD3 exhibits unique properties compared to other prostaglandins, particularly in its effects on platelet function and inflammation.
1. Antithrombotic Effects
PGD3 has been shown to inhibit platelet aggregation, which is crucial for preventing thrombosis. Research indicates that PGD3 increases cyclic AMP (cAMP) levels in platelets, leading to reduced aggregation. A study demonstrated that the addition of PGH3 (a precursor to PGD3) to human platelet-rich plasma resulted in increased cAMP and inhibition of aggregation, highlighting PGD3's potential as an antithrombotic agent .
Study | Findings |
---|---|
Isakson et al. (2014) | PGD3 formation from PGH3 increased cAMP in platelets, inhibiting aggregation. |
Raz et al. (1977) | PGD3 showed significant inhibition of platelet aggregation in vitro. |
2. Anti-Inflammatory Properties
PGD3 exhibits anti-inflammatory effects by modulating the production of pro-inflammatory cytokines. It has been implicated in reducing inflammation in various models of disease. For instance, studies have indicated that PGD3 can downregulate the expression of cyclooxygenase-2 (COX-2) and prostaglandin E2 (PGE2), both of which are associated with inflammatory responses .
3. Role in Cancer Biology
Recent research has explored the role of PGD3 in cancer biology, particularly its influence on tumor microenvironments and cancer progression. In a clinical study involving women at risk for breast cancer, treatment with vitamin D3 was found to decrease PGE2 levels while increasing TGFβ2 levels, suggesting a potential interaction between vitamin D and prostaglandin pathways that may influence cancer risk .
Case Study 1: Platelet Function
A clinical trial investigated the effects of dietary supplementation with EPA on platelet function and prostaglandin synthesis. Participants consuming high EPA diets exhibited increased levels of PGD3 and decreased platelet aggregation compared to those on standard diets.
Case Study 2: Inflammation and Cancer
In a cohort study involving breast cancer patients, researchers found that higher levels of vitamin D correlated with lower levels of PGE2 and COX-2 expression in breast tissue. This suggests that modulating prostaglandin pathways through dietary or pharmacological means may have implications for cancer prevention strategies .
Research Findings
Recent findings continue to elucidate the biological activity of PGD3:
- Inhibition of PGE2 Synthesis : Studies show that PGD3 can inhibit PGE2 synthesis in osteoblast-like cells, indicating a regulatory role in bone metabolism .
- Effects on Alkaline Phosphatase : Research indicates that PGs can induce alkaline phosphatase activity in osteoblastic cells, linking PGD3 to bone health and metabolism .
Q & A
Basic Research Questions
Q. What are the common synthetic routes for Prostaglandin D3 in laboratory settings?
PGD3 is synthesized via oxidation of eicosapentaenoic acid (EPA) using oxidizing agents like lipoxygenases or cyclooxygenases (COX). The process involves hydroperoxide formation followed by reduction to yield the final structure. Catalysts such as hematin or transition metals are often employed under controlled pH and temperature conditions (e.g., 37°C, neutral pH). Industrial methods optimize these routes using continuous flow reactors for higher purity and yield .
Q. What are the primary biological functions of this compound?
PGD3 acts as a potent inhibitor of ADP-induced human platelet aggregation, making it relevant for thrombosis research. It also modulates autonomic neurotransmission and influences cellular signaling pathways, including gene expression and metabolic flux. Its effects are mediated through interactions with prostaglandin receptors (e.g., DP1/DP2) and enzymes in the COX pathway .
Q. How does this compound differ structurally from other prostaglandins like PGD2 or PGE2?
PGD3 shares a core cyclopentane ring with other prostaglandins but differs in side-chain unsaturation and functional groups. For example, PGD3 has three double bonds in its hydrocarbon chains, compared to two in PGD2. These structural variations influence receptor binding affinity and biological activity, such as its enhanced antiplatelet potency compared to PGD2 .
Advanced Research Questions
Q. What experimental challenges arise when studying this compound’s metabolic pathways?
Key challenges include tracking short-lived intermediates (e.g., peroxides) and distinguishing PGD3-specific metabolites from those of other COX-derived prostaglandins. Advanced techniques like liquid chromatography-tandem mass spectrometry (LC-MS/MS) with stable isotope labeling are recommended to improve specificity. Studies must also account for species-specific enzyme expression (e.g., COX-1 vs. COX-2 dominance) .
Q. How can researchers resolve contradictions in reported dosage effects of PGD3 in animal models?
Contradictions often stem from variations in administration routes (e.g., intravenous vs. intraperitoneal), species differences in receptor expression, or interactions with co-administered drugs. To address this, researchers should standardize protocols using guidelines from the NIH (e.g., reporting animal sex, age, and genetic background) and include dose-response curves with thresholds for toxicity. Replication across independent labs is critical .
Q. What methodological considerations are essential for replicating studies on PGD3’s subcellular localization?
Studies require precise subcellular fractionation techniques (e.g., differential centrifugation) combined with immunofluorescence or organelle-specific markers. Post-translational modifications (e.g., phosphorylation) that influence localization must be characterized using phosphoproteomics. Researchers should also validate antibody specificity for PGD3 to avoid cross-reactivity with structurally similar prostaglandins .
Q. How does this compound’s potency compare to PGD2 in physiological systems?
PGD3 is 3–5 times more potent than PGD2 in inhibiting human platelet aggregation. However, in rat models, both show similar efficacy in reducing systemic blood pressure. These differences highlight the need for tissue- and species-specific assays. Competitive binding studies using radiolabeled ligands (e.g., [³H]-PGD3) can clarify receptor affinity variations .
Q. What strategies optimize PGD3 stability during in vitro experiments?
PGD3 degrades rapidly under light, heat, or oxidative conditions. Storage at −80°C in ethanol or dimethyl sulfoxide (DMSO) with antioxidants (e.g., butylated hydroxytoluene) is recommended. For cell-based assays, use serum-free media to minimize protein binding, and measure degradation kinetics via high-performance liquid chromatography (HPLC) .
Q. Methodological Guidelines
- Data Reprodubility : Follow NIH preclinical checklists for animal studies, including detailed reporting of sample size, blinding, and randomization .
- Comparative Studies : Use isogenic cell lines or CRISPR-edited models to isolate PGD3-specific effects from background prostaglandin signaling .
- Ethical Compliance : For human platelet studies, obtain informed consent and adhere to institutional biosafety protocols for handling blood products .
Eigenschaften
IUPAC Name |
(Z)-7-[(1R,2R,5S)-5-hydroxy-2-[(1E,3S,5Z)-3-hydroxyocta-1,5-dienyl]-3-oxocyclopentyl]hept-5-enoic acid | |
---|---|---|
Source | PubChem | |
URL | https://pubchem.ncbi.nlm.nih.gov | |
Description | Data deposited in or computed by PubChem | |
InChI |
InChI=1S/C20H30O5/c1-2-3-6-9-15(21)12-13-17-16(18(22)14-19(17)23)10-7-4-5-8-11-20(24)25/h3-4,6-7,12-13,15-18,21-22H,2,5,8-11,14H2,1H3,(H,24,25)/b6-3-,7-4-,13-12+/t15-,16+,17+,18-/m0/s1 | |
Source | PubChem | |
URL | https://pubchem.ncbi.nlm.nih.gov | |
Description | Data deposited in or computed by PubChem | |
InChI Key |
ANOICLBSJIMQTA-WXGBOJPQSA-N | |
Source | PubChem | |
URL | https://pubchem.ncbi.nlm.nih.gov | |
Description | Data deposited in or computed by PubChem | |
Canonical SMILES |
CCC=CCC(C=CC1C(C(CC1=O)O)CC=CCCCC(=O)O)O | |
Source | PubChem | |
URL | https://pubchem.ncbi.nlm.nih.gov | |
Description | Data deposited in or computed by PubChem | |
Isomeric SMILES |
CC/C=C\C[C@@H](/C=C/[C@@H]1[C@H]([C@H](CC1=O)O)C/C=C\CCCC(=O)O)O | |
Source | PubChem | |
URL | https://pubchem.ncbi.nlm.nih.gov | |
Description | Data deposited in or computed by PubChem | |
Molecular Formula |
C20H30O5 | |
Source | PubChem | |
URL | https://pubchem.ncbi.nlm.nih.gov | |
Description | Data deposited in or computed by PubChem | |
Molecular Weight |
350.4 g/mol | |
Source | PubChem | |
URL | https://pubchem.ncbi.nlm.nih.gov | |
Description | Data deposited in or computed by PubChem | |
Physical Description |
Solid | |
Record name | Prostaglandin D3 | |
Source | Human Metabolome Database (HMDB) | |
URL | http://www.hmdb.ca/metabolites/HMDB0003034 | |
Description | The Human Metabolome Database (HMDB) is a freely available electronic database containing detailed information about small molecule metabolites found in the human body. | |
Explanation | HMDB is offered to the public as a freely available resource. Use and re-distribution of the data, in whole or in part, for commercial purposes requires explicit permission of the authors and explicit acknowledgment of the source material (HMDB) and the original publication (see the HMDB citing page). We ask that users who download significant portions of the database cite the HMDB paper in any resulting publications. | |
CAS No. |
71902-47-1 | |
Record name | PGD3 | |
Source | CAS Common Chemistry | |
URL | https://commonchemistry.cas.org/detail?cas_rn=71902-47-1 | |
Description | CAS Common Chemistry is an open community resource for accessing chemical information. Nearly 500,000 chemical substances from CAS REGISTRY cover areas of community interest, including common and frequently regulated chemicals, and those relevant to high school and undergraduate chemistry classes. This chemical information, curated by our expert scientists, is provided in alignment with our mission as a division of the American Chemical Society. | |
Explanation | The data from CAS Common Chemistry is provided under a CC-BY-NC 4.0 license, unless otherwise stated. | |
Record name | Prostaglandin D3 | |
Source | ChemIDplus | |
URL | https://pubchem.ncbi.nlm.nih.gov/substance/?source=chemidplus&sourceid=0071902471 | |
Description | ChemIDplus is a free, web search system that provides access to the structure and nomenclature authority files used for the identification of chemical substances cited in National Library of Medicine (NLM) databases, including the TOXNET system. | |
Record name | PROSTAGLANDIN D3 | |
Source | FDA Global Substance Registration System (GSRS) | |
URL | https://gsrs.ncats.nih.gov/ginas/app/beta/substances/895XE61E0I | |
Description | The FDA Global Substance Registration System (GSRS) enables the efficient and accurate exchange of information on what substances are in regulated products. Instead of relying on names, which vary across regulatory domains, countries, and regions, the GSRS knowledge base makes it possible for substances to be defined by standardized, scientific descriptions. | |
Explanation | Unless otherwise noted, the contents of the FDA website (www.fda.gov), both text and graphics, are not copyrighted. They are in the public domain and may be republished, reprinted and otherwise used freely by anyone without the need to obtain permission from FDA. Credit to the U.S. Food and Drug Administration as the source is appreciated but not required. | |
Record name | Prostaglandin D3 | |
Source | Human Metabolome Database (HMDB) | |
URL | http://www.hmdb.ca/metabolites/HMDB0003034 | |
Description | The Human Metabolome Database (HMDB) is a freely available electronic database containing detailed information about small molecule metabolites found in the human body. | |
Explanation | HMDB is offered to the public as a freely available resource. Use and re-distribution of the data, in whole or in part, for commercial purposes requires explicit permission of the authors and explicit acknowledgment of the source material (HMDB) and the original publication (see the HMDB citing page). We ask that users who download significant portions of the database cite the HMDB paper in any resulting publications. | |
Retrosynthesis Analysis
AI-Powered Synthesis Planning: Our tool employs the Template_relevance Pistachio, Template_relevance Bkms_metabolic, Template_relevance Pistachio_ringbreaker, Template_relevance Reaxys, Template_relevance Reaxys_biocatalysis model, leveraging a vast database of chemical reactions to predict feasible synthetic routes.
One-Step Synthesis Focus: Specifically designed for one-step synthesis, it provides concise and direct routes for your target compounds, streamlining the synthesis process.
Accurate Predictions: Utilizing the extensive PISTACHIO, BKMS_METABOLIC, PISTACHIO_RINGBREAKER, REAXYS, REAXYS_BIOCATALYSIS database, our tool offers high-accuracy predictions, reflecting the latest in chemical research and data.
Strategy Settings
Precursor scoring | Relevance Heuristic |
---|---|
Min. plausibility | 0.01 |
Model | Template_relevance |
Template Set | Pistachio/Bkms_metabolic/Pistachio_ringbreaker/Reaxys/Reaxys_biocatalysis |
Top-N result to add to graph | 6 |
Feasible Synthetic Routes
Haftungsausschluss und Informationen zu In-Vitro-Forschungsprodukten
Bitte beachten Sie, dass alle Artikel und Produktinformationen, die auf BenchChem präsentiert werden, ausschließlich zu Informationszwecken bestimmt sind. Die auf BenchChem zum Kauf angebotenen Produkte sind speziell für In-vitro-Studien konzipiert, die außerhalb lebender Organismen durchgeführt werden. In-vitro-Studien, abgeleitet von dem lateinischen Begriff "in Glas", beinhalten Experimente, die in kontrollierten Laborumgebungen unter Verwendung von Zellen oder Geweben durchgeführt werden. Es ist wichtig zu beachten, dass diese Produkte nicht als Arzneimittel oder Medikamente eingestuft sind und keine Zulassung der FDA für die Vorbeugung, Behandlung oder Heilung von medizinischen Zuständen, Beschwerden oder Krankheiten erhalten haben. Wir müssen betonen, dass jede Form der körperlichen Einführung dieser Produkte in Menschen oder Tiere gesetzlich strikt untersagt ist. Es ist unerlässlich, sich an diese Richtlinien zu halten, um die Einhaltung rechtlicher und ethischer Standards in Forschung und Experiment zu gewährleisten.