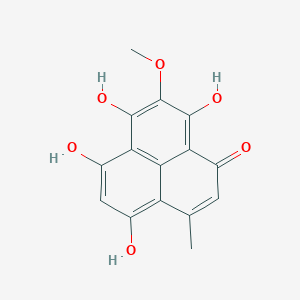
Funalenone
Übersicht
Beschreibung
Funalenone (C₁₅H₁₂O₅; molecular weight: 288.25 Da) is a phenalenone-type polyketide produced by fungal species such as Aspergillus niger and Aspergillus tubingensis . Beyond collagenase inhibition, this compound exhibits diverse bioactivities, including:
- HIV-1 integrase inhibition: Disrupts viral DNA integration into host genomes .
- Antibacterial activity: Inhibits bacterial cell wall synthesis enzymes MraY and MurG (IC₅₀: 25.5 μM in membrane plate assays) and suppresses Staphylococcus aureus growth (MIC: 64 μg/mL) .
- Matrix metalloproteinase (MMP) modulation: Selective inhibition of MMP-1 (IC₅₀: 170 μM) with weaker effects on MMP-2 (18.3% inhibition at 400 μM) and MMP-9 (38.2% inhibition at 400 μM) .
Structurally, this compound features a tricyclic phenalenone core with a C2 methoxy group and hydroxyl substituents, critical for its bioactivity . Its biosynthesis involves a non-reducing polyketide synthase (NR-PKS) and downstream modifying enzymes, as observed in A. niger gene clusters .
Vorbereitungsmethoden
Microbial Fermentation: Strain Selection and Culture Conditions
Funalenone is biosynthesized by the filamentous fungus Aspergillus niger FO-5904, a strain isolated for its high metabolite productivity . The fermentation process involves submerged culture techniques optimized for secondary metabolite production.
Fermentation Medium Composition
The medium comprises carbon and nitrogen sources designed to promote fungal growth and this compound biosynthesis :
Component | Concentration (%) | Role in Fermentation |
---|---|---|
Sucrose | 2.0 | Primary carbon source |
Glucose | 1.0 | Secondary carbon source |
Corn steep powder | 0.5 | Nitrogen and micronutrient supply |
Meat extract | 0.5 | Amino acids and growth factors |
KH₂PO₄ | 0.1 | Phosphorus source, pH buffer |
MgSO₄·7H₂O | 0.05 | Magnesium and sulfur supply |
CaCO₃ | 0.3 | pH stabilization |
Agar | 0.1 | Matrix for mycelial growth |
Fermentation Parameters
Critical parameters for maximizing this compound yield include :
-
Temperature : 27°C (±1°C)
-
Incubation Period : 13 days
-
Aeration : Static culture conditions in 1000-ml bottles containing 250 ml medium
-
pH : Initial pH 6.0, naturally acidifying to ~4.5 during growth
Under these conditions, the fungus produces approximately 120–150 mg of this compound per liter of culture broth .
Extraction of this compound from Fungal Biomass
Biomass Pretreatment
Post-fermentation, the mycelial mat is separated from the broth via filtration or centrifugation. The biomass undergoes homogenization to disrupt cell walls and release intracellular metabolites :
-
Homogenization : Ultra-Turrax® homogenizer at 7,000–9,000 rpm for 3–5 minutes in brine solution (300 ml per 500-ml batch)
-
Solvent System : Ethyl acetate (300 ml) added to create a biphasic mixture
Liquid-Liquid Extraction
The homogenate is transferred to a separating funnel and stirred at 1,100 rpm for 5 minutes to facilitate phase separation :
Step | Details |
---|---|
Primary extraction | Ethyl acetate layer separated and dried over anhydrous Na₂SO₄ |
Secondary extraction | Aqueous phase re-extracted twice with fresh ethyl acetate |
Combined organic phases | Filtered through Whatman No. 1 paper and concentrated via rotary evaporation |
This protocol achieves >90% recovery of this compound from the biomass .
Purification Strategies
Initial Crude Purification
The concentrated extract undergoes sequential chromatography:
ODS Column Chromatography
-
Stationary Phase : Octadecylsilyl (ODS) silica gel
-
Mobile Phase : Gradient of methanol-water (50:50 → 80:20 v/v)
Sephadex LH-20 Chromatography
-
Column : Sephadex LH-20 in methanol
-
Elution : Isocratic mode with methanol
Final Purification via Preparative HPLC
For pharmaceutical-grade this compound (>98% purity), preparative HPLC is employed :
Parameter | Specification |
---|---|
Column | C18 reversed-phase (250 × 20 mm, 5 µm) |
Mobile Phase | Acetonitrile-water (55:45, 0.1% TFA) |
Flow Rate | 10 ml/min |
Detection | UV at 254 nm |
Retention Time | 14.2 minutes |
Synthetic Approaches: Challenges and Progress
While microbial fermentation remains the primary production method, chemical synthesis routes have been explored to overcome limitations in natural yield. Key synthetic steps include:
Retrosynthetic Analysis
Proposed routes involve phenalenone core construction via:
-
Friedel-Crafts Acylation : To establish the tricyclic framework
-
Selective Methylation : Using dimethyl sulfate under alkaline conditions
-
Oxidative Dearomatization : For introducing hydroxyl groups
Current Limitations
-
Step Count : 8–10 linear steps required
-
Overall Yield : <5% in optimized routes due to poor regioselectivity in hydroxylation steps
-
Cost : 3–5× higher than fermentation-derived material
Comparative Analysis of Preparation Methods
Parameter | Microbial Fermentation | Chemical Synthesis |
---|---|---|
Yield (g/L) | 0.12–0.15 | 0.02–0.05 (theoretical) |
Purity (%) | 98–99 (post-HPLC) | 85–90 (after chromatography) |
Production Cycle | 14–16 days | 4–6 weeks |
Cost (USD/g) | 120–150 | 400–600 |
Scalability | Industrial-scale feasible | Limited to lab-scale |
Industrial-Scale Optimization Strategies
Fermentation Enhancements
-
Strain Engineering : CRISPR-Cas9 modification of A. niger to overexpress polyketide synthase genes
-
Fed-Batch Culture : Sucrose feeding to maintain carbon levels without catabolite repression
Extraction Innovations
-
Microwave-Assisted Extraction : 30% reduction in solvent use with comparable yield
-
Ionic Liquid Systems : [C₄mim][PF₆]-water systems showing 95% partition coefficients
Quality Control and Characterization
Spectroscopic Identification
-
UV-Vis : λmax 254 nm (methanol)
-
IR (KBr) : 3400 cm⁻¹ (OH), 1660 cm⁻¹ (C=O), 1600 cm⁻¹ (aromatic C=C)
-
¹H NMR (DMSO-d₆) : δ 2.48 (s, 3H, CH₃), 3.89 (s, 3H, OCH₃), 6.20–7.15 (m, aromatic H)
Purity Assessment
-
HPLC-DAD : >98% purity threshold for biomedical applications
-
LC-MS : m/z 289.1 [M+H]⁺, 311.1 [M+Na]⁺
Condition | Stability Profile |
---|---|
Aqueous Solution (pH 7) | <24 hours at 25°C |
Dry Powder | 24 months at -20°C (under N₂ atmosphere) |
Organic Solvents | Stable in DMSO/ethanol (1 year at 4°C) |
Analyse Chemischer Reaktionen
Types of Reactions: Funalenone undergoes various chemical reactions, including:
Oxidation: this compound can be oxidized to form quinones and other oxidized derivatives.
Reduction: Reduction of this compound can lead to the formation of hydroxy derivatives.
Substitution: this compound can undergo substitution reactions, particularly at the hydroxyl and methoxy groups.
Common Reagents and Conditions:
Oxidation: Common oxidizing agents include potassium permanganate and chromium trioxide.
Reduction: Reducing agents such as sodium borohydride and lithium aluminum hydride are used.
Substitution: Substitution reactions often involve reagents like halogens, acids, and bases under controlled conditions.
Major Products: The major products formed from these reactions include various hydroxylated, methoxylated, and quinone derivatives of this compound .
Wissenschaftliche Forschungsanwendungen
Funalenone has several scientific research applications, including:
Chemistry: this compound is used as a model compound in studies of phenalenone chemistry and its derivatives.
Biology: It is studied for its inhibitory effects on enzymes such as HIV-1 integrase and matrix metalloproteinase-1 (MMP-1).
Medicine: this compound’s potential as an antiviral and anticancer agent is being explored due to its ability to inhibit key enzymes involved in disease progression.
Wirkmechanismus
Funalenone exerts its effects by inhibiting specific enzymes. For example, it inhibits HIV-1 integrase by binding to the enzyme’s active site, preventing the integration of viral DNA into the host genome. Similarly, it inhibits matrix metalloproteinase-1 (MMP-1) by interacting with the enzyme’s catalytic domain, thereby blocking its activity. These interactions disrupt critical biological pathways, leading to the compound’s antiviral and anticancer properties .
Vergleich Mit ähnlichen Verbindungen
Comparison with Structurally Similar Compounds
Pinoquercetin (ChEBI 8224)
- Structural similarity: Shares 70% structural similarity with Funalenone, as identified via ChEBI and ZINC database screenings .
- Docking performance: In virtual screening for leptospiral collagenase inhibitors, Pinoquercetin analogs exhibited lower binding affinities compared to this compound derivatives .
Lachnanthocarpone
- Structural class: A plant-derived phenalenone with photodynamic therapy (PDT) applications .
- Comparison: Both compounds share a phenalenone core, but this compound’s methoxy and hydroxyl groups enhance solubility and enzyme interactions, unlike lachnanthocarpone’s alkylated structure .
Erabulenol B
- Bioactivity: Inhibits cholesteryl ester transfer protein (CETP), contrasting with this compound’s collagenase and MMP-1 inhibition .
- Structural divergence: Erabulenol B lacks the C2 methoxy group, reducing its overlap with this compound’s binding pockets .
Hispidulones A and B
- Source : Isolated from Chaetosphaeronema hispidulum .
- Activity: Exhibit antifungal properties, whereas this compound targets bacterial and human enzymes .
Functional Comparison with Pharmacologically Relevant Analogs
Mechanistic Insights from Docking Studies
Virtual screening of 67 this compound analogs identified key structural determinants for collagenase inhibition:
- Hydrophobic interactions: The phenalenone core anchors the compound in collagenase’s active site .
- Methoxy group role : Enhances binding affinity by forming hydrogen bonds with catalytic residues (e.g., Glu 143 and Zn²⁺ in MMP-1) .
- Superiority over Pinoquercetin: this compound’s rigid tricyclic system provides better steric complementarity compared to Pinoquercetin’s flexible flavonoid structure .
Biologische Aktivität
Funalenone is a phenalenone compound primarily isolated from the fungal strain Aspergillus niger FO-5904. This compound has garnered attention due to its various biological activities, particularly in the fields of collagenase inhibition and antiviral properties. This article provides a comprehensive overview of the biological activities of this compound, supported by data tables and relevant research findings.
Chemical Structure and Properties
This compound is characterized by the molecular formula and a molecular weight of 288.26 g/mol. It appears as a yellow powder, soluble in DMSO, methanol, ethanol, and ethyl acetate, but insoluble in water and several organic solvents like acetone and hexane .
1. Collagenase Inhibition
One of the most significant biological activities of this compound is its ability to inhibit collagenase, particularly type I collagenase (MMP-1). This enzyme plays a crucial role in extracellular matrix remodeling and is implicated in pathological conditions such as arthritis.
- Inhibition Data :
This inhibitory activity suggests potential therapeutic applications in treating diseases characterized by excessive collagen degradation.
2. Antiviral Activity
This compound has also been studied for its antiviral properties, particularly against HIV-1. The compound inhibits HIV-1 integrase, an essential enzyme for viral replication.
- Inhibition Data :
These results indicate that this compound could be a candidate for further development as an antiviral agent.
3. Antimicrobial Activity
Despite its promising activities against collagenase and HIV-1 integrase, this compound exhibited no significant antimicrobial activity against various bacteria and fungi at a concentration of 50 µg/disc .
Summary of Biological Activities
The following table summarizes the key biological activities of this compound:
Biological Activity | Target/Enzyme | IC50 Value (µM) | Comments |
---|---|---|---|
Collagenase Inhibition | Type I Collagenase (MMP-1) | 170 | Significant inhibitor; potential therapeutic use |
Type IV Collagenases | 400 | Moderate inhibition observed | |
HIV-1 Integrase Inhibition | HIV-1 Integrase | 10 | Effective inhibitor; promising antiviral agent |
Anti-HIV Activity | HIV-1 | 1.7 | Low cytotoxicity; potential for drug development |
Antimicrobial Activity | Various (bacteria/fungi) | N/A | No significant activity observed |
Case Studies and Research Findings
Recent studies have expanded on the potential applications of this compound:
- A study highlighted its role as an effective inhibitor of collagenase, suggesting it may help manage conditions involving joint degradation .
- Another research article discussed the molecular dynamics simulations indicating that this compound derivatives could serve as hGLUT1 inhibitors, opening avenues for cancer therapy .
Q & A
Q. What experimental models are optimal for studying Funalenone’s dual inhibitory activity against HIV-1 integrase and MMP-1?
Basic Research Focus
To assess this compound’s inhibitory effects, utilize in vitro enzyme inhibition assays. For HIV-1 integrase, employ strand-transfer assays using recombinant integrase and oligonucleotide substrates, measuring inhibition via fluorescence resonance energy transfer (FRET) or gel electrophoresis . For MMP-1, use fluorogenic substrates (e.g., Mca-Pro-Leu-Gly-Leu-Dpa-Ala-Arg-NH₂) to quantify enzymatic activity reduction via fluorescence intensity changes. Include positive controls (e.g., Raltegravir for HIV-1 integrase; Ilomastat for MMP-1) and validate results with dose-response curves (IC₅₀ calculations) .
Advanced Research Focus
Investigate synergistic or antagonistic effects of dual inhibition using cell-based models. For example, co-culture HIV-infected T-cells (e.g., MT-4 cells) with MMP-1-secreting fibroblasts to evaluate this compound’s impact on viral replication and extracellular matrix degradation simultaneously. Use RNA sequencing to identify off-target pathways affected by dual inhibition .
Q. How can researchers resolve contradictions in this compound’s role in quorum sensing (QS) inhibition across bacterial species?
Basic Research Focus
Standardize QS inhibition assays using reporter strains (e.g., Pseudomonas aeruginosa lasB-GFP, rhlA-GFP) to measure signal molecule suppression (e.g., 3-oxo-C12-HSL, C4-HSL). Normalize data by growth rates (OD₆₀₀) to distinguish true QS inhibition from growth inhibition artifacts .
Advanced Research Focus
Employ metabolomic profiling (LC-MS) to compare this compound’s effects on QS molecules in P. aeruginosa versus Staphylococcus aureus. Cross-validate findings with transcriptomic data to identify species-specific regulatory networks. Address contradictions by analyzing structural analogs (e.g., Aurasperone A) to determine if this compound’s activity is scaffold-dependent .
Q. What methodologies are recommended for elucidating this compound’s biosynthetic pathway in Aspergillus niger?
Basic Research Focus
Use gene knockout strains (e.g., ΔalbA, ΔaygA) and compare metabolite profiles via HPLC-DAD-MS. Monitor intermediates like kotanin and dimeric naphtho-γ-pyrones to map biosynthetic steps .
Advanced Research Focus
Conduct isotopic labeling (¹³C-glucose) to trace carbon flux through polyketide synthase (PKS) pathways. Combine with heterologous expression of candidate PKS genes (e.g., albA) in S. cerevisiae to confirm enzyme functionality. Resolve discrepancies in pathway models (e.g., this compound detection in ΔalbA mutants) by probing alternative PKS clusters .
Q. How should researchers design dose-response studies to account for this compound’s cytotoxicity in mammalian cells?
Basic Research Focus
Perform MTT/WST-1 assays on human cell lines (e.g., HEK-293, HepG2) to establish cytotoxicity thresholds (IC₅₀). Use sub-cytotoxic doses (≤IC₁₀) for functional studies (e.g., MMP-1 inhibition) .
Advanced Research Focus
Apply high-content screening (HCS) to quantify apoptosis (Annexin V/PI staining) and mitochondrial membrane potential (JC-1 dye) in real-time. Integrate pharmacokinetic modeling to predict tissue-specific toxicity in vivo .
Q. What statistical approaches are suitable for analyzing contradictory data on this compound’s stability under varying pH conditions?
Basic Research Focus
Conduct accelerated stability studies (pH 2–9, 37°C) and quantify degradation via HPLC. Use ANOVA with post-hoc Tukey tests to compare half-lives across pH groups .
Advanced Research Focus
Apply multivariate analysis (e.g., PCA) to correlate degradation products with pH and temperature. Validate degradation pathways using Q-TOF-MS/MS and molecular dynamics simulations to predict hydrolysis-prone sites .
Q. How can researchers validate this compound’s off-target effects in complex biological systems?
Basic Research Focus
Perform kinome-wide profiling (e.g., KinomeScan) to identify unintended kinase interactions. Cross-reference with STRING database to predict affected signaling cascades .
Advanced Research Focus
Use CRISPR-Cas9 screens in haploid cell lines to identify synthetic lethal interactions. Combine with thermal proteome profiling (TPP) to map this compound-binding proteins .
Q. What strategies address the low solubility of this compound in aqueous assays?
Basic Research Focus
Optimize solvent systems (e.g., DMSO ≤0.1% v/v) and use surfactants (e.g., Pluronic F-68) to enhance dispersion. Validate solubility via nephelometry .
Advanced Research Focus
Develop nanoformulations (e.g., liposomes, cyclodextrin complexes) and characterize encapsulation efficiency (DSC, TEM). Assess bioactivity retention using in vitro infectivity models .
Q. How should conflicting reports on this compound’s role in fungal secondary metabolism be reconciled?
Basic Research Focus
Compare metabolite profiles of wild-type and Δpks mutants under varied culture conditions (e.g., carbon source, light exposure). Use NMR to structurally confirm intermediates .
Advanced Research Focus
Apply multi-omics integration (transcriptomics, proteomics, metabolomics) to identify regulatory cross-talk between PKS and non-ribosomal peptide synthase (NRPS) clusters. Test hypotheses via chromatin immunoprecipitation (ChIP) of transcription factors .
Eigenschaften
IUPAC Name |
4,6,7,9-tetrahydroxy-8-methoxy-3-methylphenalen-1-one | |
---|---|---|
Source | PubChem | |
URL | https://pubchem.ncbi.nlm.nih.gov | |
Description | Data deposited in or computed by PubChem | |
InChI |
InChI=1S/C15H12O6/c1-5-3-6(16)10-12-9(5)7(17)4-8(18)11(12)14(20)15(21-2)13(10)19/h3-4,17-20H,1-2H3 | |
Source | PubChem | |
URL | https://pubchem.ncbi.nlm.nih.gov | |
Description | Data deposited in or computed by PubChem | |
InChI Key |
LHEJVMYQRYQFKB-UHFFFAOYSA-N | |
Source | PubChem | |
URL | https://pubchem.ncbi.nlm.nih.gov | |
Description | Data deposited in or computed by PubChem | |
Canonical SMILES |
CC1=CC(=O)C2=C(C(=C(C3=C2C1=C(C=C3O)O)O)OC)O | |
Source | PubChem | |
URL | https://pubchem.ncbi.nlm.nih.gov | |
Description | Data deposited in or computed by PubChem | |
Molecular Formula |
C15H12O6 | |
Source | PubChem | |
URL | https://pubchem.ncbi.nlm.nih.gov | |
Description | Data deposited in or computed by PubChem | |
DSSTOX Substance ID |
DTXSID00438324 | |
Record name | 1H-Phenalen-1-one, 3,4,7,9-tetrahydroxy-2-methoxy-6-methyl- | |
Source | EPA DSSTox | |
URL | https://comptox.epa.gov/dashboard/DTXSID00438324 | |
Description | DSSTox provides a high quality public chemistry resource for supporting improved predictive toxicology. | |
Molecular Weight |
288.25 g/mol | |
Source | PubChem | |
URL | https://pubchem.ncbi.nlm.nih.gov | |
Description | Data deposited in or computed by PubChem | |
CAS No. |
259728-61-5 | |
Record name | 1H-Phenalen-1-one, 3,4,7,9-tetrahydroxy-2-methoxy-6-methyl- | |
Source | EPA DSSTox | |
URL | https://comptox.epa.gov/dashboard/DTXSID00438324 | |
Description | DSSTox provides a high quality public chemistry resource for supporting improved predictive toxicology. | |
Retrosynthesis Analysis
AI-Powered Synthesis Planning: Our tool employs the Template_relevance Pistachio, Template_relevance Bkms_metabolic, Template_relevance Pistachio_ringbreaker, Template_relevance Reaxys, Template_relevance Reaxys_biocatalysis model, leveraging a vast database of chemical reactions to predict feasible synthetic routes.
One-Step Synthesis Focus: Specifically designed for one-step synthesis, it provides concise and direct routes for your target compounds, streamlining the synthesis process.
Accurate Predictions: Utilizing the extensive PISTACHIO, BKMS_METABOLIC, PISTACHIO_RINGBREAKER, REAXYS, REAXYS_BIOCATALYSIS database, our tool offers high-accuracy predictions, reflecting the latest in chemical research and data.
Strategy Settings
Precursor scoring | Relevance Heuristic |
---|---|
Min. plausibility | 0.01 |
Model | Template_relevance |
Template Set | Pistachio/Bkms_metabolic/Pistachio_ringbreaker/Reaxys/Reaxys_biocatalysis |
Top-N result to add to graph | 6 |
Feasible Synthetic Routes
Haftungsausschluss und Informationen zu In-Vitro-Forschungsprodukten
Bitte beachten Sie, dass alle Artikel und Produktinformationen, die auf BenchChem präsentiert werden, ausschließlich zu Informationszwecken bestimmt sind. Die auf BenchChem zum Kauf angebotenen Produkte sind speziell für In-vitro-Studien konzipiert, die außerhalb lebender Organismen durchgeführt werden. In-vitro-Studien, abgeleitet von dem lateinischen Begriff "in Glas", beinhalten Experimente, die in kontrollierten Laborumgebungen unter Verwendung von Zellen oder Geweben durchgeführt werden. Es ist wichtig zu beachten, dass diese Produkte nicht als Arzneimittel oder Medikamente eingestuft sind und keine Zulassung der FDA für die Vorbeugung, Behandlung oder Heilung von medizinischen Zuständen, Beschwerden oder Krankheiten erhalten haben. Wir müssen betonen, dass jede Form der körperlichen Einführung dieser Produkte in Menschen oder Tiere gesetzlich strikt untersagt ist. Es ist unerlässlich, sich an diese Richtlinien zu halten, um die Einhaltung rechtlicher und ethischer Standards in Forschung und Experiment zu gewährleisten.