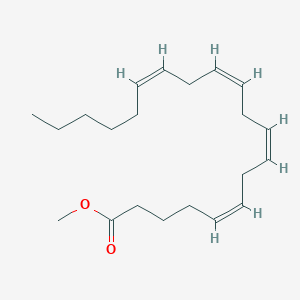
Methyl arachidonate
Übersicht
Beschreibung
Methyl arachidonate (C₂₁H₃₄O₂), also known as methyl (5Z,8Z,11Z,14Z)-eicosa-5,8,11,14-tetraenoate, is a fatty acid methyl ester derived from arachidonic acid (AA), a polyunsaturated omega-6 fatty acid . Its molecular weight is 318.50 g/mol, and it is characterized by four cis double bonds at positions 5, 8, 11, and 12. This compound is poorly water-soluble (3.8 × 10⁻⁵ g/L) but exhibits lipophilic properties (logP = 6.85), making it suitable for lipid-rich environments . It is synthesized via esterification of AA, a process that enhances its stability and bioavailability for research applications .
This compound has been implicated in diverse biological pathways, including modulation of the MAPK signaling cascade, protein kinase C (PKC) activation, and eicosanoid metabolism .
Vorbereitungsmethoden
Enzymatic Transesterification Using Lipases
Enzymatic methods have gained prominence due to their specificity and mild reaction conditions. A two-step enzymatic process described by Park et al. (2015) involves the ethanolysis of arachidonic acid-rich triglycerides to produce 2-monoacylglycerols (2-MAGs), followed by transesterification with methanol to yield methyl arachidonate . The first step employs Rhizomucor miehei lipase, which selectively cleaves ester bonds at the sn-1 and sn-3 positions, leaving the arachidonoyl group intact at the sn-2 position. The second step utilizes Candida antarctica lipase B to catalyze the methanolysis of 2-MAGs, achieving a 92% conversion rate under optimized conditions (40°C, 1:5 substrate-to-methanol ratio, 24 h) .
This method minimizes side reactions such as hydrolysis or isomerization, which are common in acid-catalyzed processes. However, enzyme stability remains a challenge, as prolonged exposure to methanol reduces lipase activity by 30% after five cycles .
Acid-Catalyzed Esterification
Traditional esterification using sulfuric acid (H₂SO₄) or hydrochloric acid (HCl) is widely employed for its simplicity and cost-effectiveness. Arachidonic acid reacts with methanol in a 1:10 molar ratio under reflux (65°C) for 12–24 hours, achieving yields of 85–90% . The mechanism involves protonation of the carboxylic acid, followed by nucleophilic attack by methanol to form the ester.
Optimization Data:
Parameter | Optimal Value | Yield Impact |
---|---|---|
Temperature | 65°C | +15% vs. 50°C |
Methanol Ratio | 1:10 | +20% vs. 1:5 |
Catalyst (H₂SO₄) | 2% v/v | +10% vs. 1% |
Post-reaction purification involves neutralization with sodium bicarbonate, followed by distillation under reduced pressure (0.1 mmHg, 120°C) to isolate this compound . While efficient, this method risks dehydration of arachidonic acid, leading to conjugated diene formation, which reduces purity .
Diazomethane-Mediated Methylation
Diazomethane (CH₂N₂) offers a rapid, room-temperature methylation method. Arachidonic acid reacts with CH₂N₂ in diethyl ether, forming this compound within 30 minutes with near-quantitative yields . The reaction proceeds via nucleophilic attack by the carboxylate anion on the electrophilic diazomethane, releasing nitrogen gas.
Safety Considerations:
-
Diazomethane is highly toxic and explosive, necessitating in-situ generation from precursors like N-methyl-N-nitrosourea.
-
Copper(I) chloride (CuCl) is often added to catalyze the reaction and suppress side reactions .
Despite its efficiency, this method is less favored industrially due to safety hazards and the difficulty of scaling up diazomethane synthesis.
Base-Catalyzed Transesterification of Triglycerides
Saponification followed by methylation is another viable route. Arachidonic acid-rich oils (e.g., from Mortierella alpina) are saponified with potassium hydroxide (KOH) in ethanol, releasing free arachidonic acid, which is then methylated using boron trifluoride-methanol (BF₃-MeOH) . This two-step process achieves 88–92% purity, though BF₃ poses corrosion risks and requires careful handling .
Comparative Analysis of Methods:
Method | Yield (%) | Purity (%) | Scalability | Safety |
---|---|---|---|---|
Enzymatic | 92 | ≥99 | Moderate | High |
Acid-Catalyzed | 90 | 85–90 | High | Moderate |
Diazomethane | 95–98 | ≥99 | Low | Low |
Base-Catalyzed | 88 | 88–92 | High | Moderate |
Purification and Characterization
This compound is typically purified via silica gel chromatography or molecular distillation. Gas chromatography (GC) with flame ionization detection (FID) confirms purity, while nuclear magnetic resonance (NMR) spectroscopy validates structural integrity.
GC Conditions (Sigma-Aldrich Protocol) :
-
Column: DB-5MS (30 m × 0.25 mm × 0.25 µm)
-
Temperature Program: 50°C (2 min) → 10°C/min → 280°C (5 min)
-
Retention Time: 18.7 min
Analyse Chemischer Reaktionen
Reaktionstypen: Methylaarachidonat unterliegt verschiedenen chemischen Reaktionen, darunter Oxidation, Reduktion und Substitution.
Häufige Reagenzien und Bedingungen:
Oxidation: Methylaarachidonat kann mit Reagenzien wie Kaliumpermanganat oder Ozon oxidiert werden.
Substitution: Halogenierungsreaktionen können mit Reagenzien wie Brom oder Chlor auftreten, was zur Bildung von halogenierten Derivaten führt.
Hauptprodukte: Die Hauptprodukte, die aus diesen Reaktionen gebildet werden, umfassen Epoxide, Hydroperoxide und halogenierte Fettsäuremethylester .
Wissenschaftliche Forschungsanwendungen
Biochemical Properties and Mechanisms of Action
Methyl arachidonate is known for its potent biological activity, particularly in the activation of protein kinase C (PKC). Research indicates that it can activate PKC in intact platelets similarly to arachidonic acid. The activation mechanism varies with concentration; at lower concentrations (5 µM), activation is primarily through cyclooxygenase products, while at higher concentrations (50 µM), it involves the lipoxygenase pathway .
Pharmacological Applications
- Protein Kinase C Activation
- Neuroprotective Effects
- Lipid Peroxidation and DNA Damage
Nutritional Applications
This compound is also explored for its role in nutrition, particularly concerning fatty acid metabolism:
- Dietary Lipids : Its incorporation into diets has been studied for effects on meat quality in livestock, indicating potential benefits for animal nutrition and food science .
- Fatty Acid Composition : The balance of this compound with other fatty acids can influence health outcomes related to inflammatory processes and cardiovascular health.
Case Studies and Research Findings
Study | Findings | Applications |
---|---|---|
Park JW et al. (1992) | This compound mediates oxidative DNA damage through lipid peroxidation products. | Implications for aging and disease mechanisms. |
Research on PC12 Cells | This compound protects against MPP+-induced toxicity. | Potential neuroprotective agent in neurodegenerative diseases. |
Meat Quality Study | Investigated the impact of dietary this compound on lamb meat quality. | Enhancements in animal nutrition practices. |
Wirkmechanismus
Methyl arachidonate exerts its effects primarily through its conversion to arachidonic acid and subsequent metabolism by cyclooxygenases, lipoxygenases, and cytochrome P450 enzymes . These enzymes convert arachidonic acid into various eicosanoids, including prostaglandins, thromboxanes, and leukotrienes, which play crucial roles in inflammation, immune responses, and other physiological processes .
Vergleich Mit ähnlichen Verbindungen
Structural and Functional Comparison
Methyl arachidonate is structurally related to other fatty acid esters, including methyl linoleate (C₁₉H₃₄O₂) and ethyl arachidonate (C₂₂H₃₆O₂). Key differences include:
- Degree of Unsaturation: this compound contains four double bonds, whereas methyl linoleate has two. This higher unsaturation increases its reactivity and susceptibility to oxidation .
- Ester Group : Ethyl arachidonate replaces the methyl group with an ethyl group, marginally increasing its hydrophobicity and altering metabolic stability .
Metabolic Pathways
- Arachidonate vs. Linoleate Metabolism: Compound Metabolic Role Key Enzymes Involved this compound Precursor for eicosanoids (e.g., prostaglandins, leukotrienes) via COX/LOX pathways . Cyclooxygenase (COX), 5-lipoxygenase (5-LOX) Methyl linoleate Converted to AA via elongation/desaturation; indirectly supports eicosanoid synthesis . Δ6-desaturase, elongases this compound directly integrates into AA metabolism, bypassing the need for enzymatic conversion, whereas methyl linoleate requires elongation and desaturation to become bioactive .
Oxidative Stability and Byproducts
This compound is highly prone to oxidation, generating volatile aldehydes (e.g., hexanal, 2,4-decadienal) and ketones that contribute to off-odors in lipid-rich systems . In contrast, methyl linoleate produces fewer oxidation byproducts due to lower unsaturation .
Data Tables
Table 1. Key Physicochemical Properties
Property | This compound | Methyl Linoleate | Ethyl Arachidonate |
---|---|---|---|
Molecular Formula | C₂₁H₃₄O₂ | C₁₉H₃₄O₂ | C₂₂H₃₆O₂ |
logP | 6.85 | 6.30 | 7.10 |
Water Solubility (g/L) | 3.8 × 10⁻⁵ | 1.2 × 10⁻⁴ | 2.5 × 10⁻⁵ |
Key Metabolic Pathway | Direct COX/LOX | Elongation | Direct COX/LOX |
Table 2. Oxidation Byproducts
Compound | Major Byproducts |
---|---|
This compound | Hexanal, 2,4-decadienal, pentane |
Methyl linoleate | Hexanal, nonanal |
Biologische Aktivität
Methyl arachidonate, a methyl ester of arachidonic acid, exhibits significant biological activities that are crucial for various physiological processes. This article explores its biochemical roles, mechanisms of action, and the implications of its activity in health and disease through detailed research findings, data tables, and case studies.
Overview of this compound
This compound is derived from arachidonic acid (AA), a polyunsaturated fatty acid that serves as a precursor for bioactive lipid mediators such as prostaglandins and leukotrienes. These mediators play essential roles in inflammation, immunity, and cellular signaling. This compound is particularly noted for its potent activation of protein kinase C (PKC), which is involved in various cellular processes including proliferation, differentiation, and apoptosis.
- Activation of Protein Kinase C (PKC)
- Influence on Ion Channels
- Role in Inflammation
Biological Activity Data
The following table summarizes key biological activities associated with this compound:
Case Study 1: Cardiovascular Implications
A study investigated the effects of this compound on cardiac myocytes under ischemic conditions. Results demonstrated that this compound effectively reduced the incidence of arrhythmias by modulating Na+ channel activity, highlighting its potential therapeutic role in cardiac protection.
Case Study 2: Inflammatory Responses
In vitro studies showed that this compound enhances the production of pro-inflammatory cytokines in macrophages. This suggests that while it plays a role in promoting inflammation, it may also contribute to the resolution phase through its metabolites.
Research Findings
Recent research has elucidated several key findings regarding the biological activity of this compound:
- Oxidative Stability : this compound undergoes autoxidation to produce various monohydroperoxide isomers, which have been shown to exhibit distinct biological effects .
- Neuroprotective Properties : Studies indicate that this compound may enhance synaptic plasticity and neuroprotection through its action on neuronal ion channels .
- Role in Cancer Biology : Investigations into the role of this compound in cancer have revealed its dual nature; it can promote tumorigenesis via inflammatory pathways while also exhibiting anti-tumor properties depending on the context .
Q & A
Basic Research Questions
Q. What are the recommended analytical methods for quantifying methyl arachidonate in complex lipid mixtures, and how do researchers address its instability during analysis?
this compound (C20:4n6) is typically quantified using gas chromatography (GC) with flame ionization detection (FID) or mass spectrometry (MS). High-resolution GC columns (e.g., DEGS-coated) are employed to resolve polyunsaturated fatty acid methyl esters (FAMEs) from co-eluting species . To mitigate auto-oxidation, samples should be stored at -20°C under inert gas, and antioxidants (e.g., α-tocopherol) should be added during extraction . Standard protocols recommend using fresh reference materials (≥95% purity) and validating recovery rates via internal standards (e.g., methyl heneicosanoate) .
Q. How is this compound synthesized and purified for experimental use in lipid metabolism studies?
this compound is synthesized via esterification of free arachidonic acid using methanol under acidic catalysis. Post-synthesis, purification involves silica column chromatography to remove unreacted arachidonate and tocopherols. High-performance liquid chromatography (HPLC) with UV detection (λmax ~235 nm) ensures isolation of specific HPETE (hydroperoxyeicosatetraenoic acid) isomers, which are critical intermediates in eicosanoid biosynthesis . Purity validation requires GC-MS or NMR to confirm structural integrity and absence of oxidative byproducts .
Advanced Research Questions
Q. What experimental strategies resolve contradictions in this compound's role in platelet activation versus cyclooxygenase inhibition?
Conflicting reports on this compound’s dual effects—activating platelets via thromboxane A2 synthesis while inhibiting cyclooxygenase (COX)—highlight the need for precise experimental design. Researchers use receptor-specific agonists (e.g., thrombin) and antagonists (e.g., aspirin) to isolate COX-dependent pathways. Parallel assays measuring thromboxane B2 (TXB2) and 12-HETE production under varying H₂O₂ concentrations can clarify redox-sensitive mechanisms . Dose-response studies (0.1–20 µM this compound) are critical, as low doses may enhance aggregation, while higher doses saturate COX active sites .
Q. How do phospholipase A₂ (PLA₂) and reacylation pathways dynamically regulate this compound bioavailability in cellular models?
PLA₂ liberates arachidonate from phosphatidylcholine, which is subsequently esterified to this compound for experimental use. Competing reacylation by lysophospholipid acyltransferases complicates kinetic studies. Researchers employ isotopic tracing (³H/¹⁴C-arachidonate) and inhibitors like methylmercury chloride to block reacylation. Time-course experiments in thrombin-stimulated platelets reveal that >85% of mobilized arachidonate originates from PLA₂, with <15% from phospholipase C (PLC) pathways . Data normalization to total phospholipid content is essential to account for cell-specific variability .
Q. What methodologies are used to investigate this compound’s contribution to advanced glycation end products (AGEs) under oxidative stress?
this compound participates in lipid peroxidation, generating glyoxal, a precursor of N-(carboxymethyl)lysine (CML). In vitro models incubate this compound with proteins (e.g., RNase) under copper-catalyzed aerobic conditions. CML quantification via ELISA or LC-MS/MS reveals arachidonate-derived CML yields (~0.7 mmol/mol lysine) far exceed glucose-derived CML, implicating lipid peroxidation as a dominant AGE source in atherosclerosis and diabetes . Antioxidant co-treatment (e.g., Trolox) validates oxidative specificity .
Q. Methodological Challenges and Solutions
Q. How do researchers address discrepancies in this compound’s stability across storage conditions and experimental systems?
this compound’s susceptibility to auto-oxidation necessitates strict storage protocols: anhydrous solvents (e.g., DMSO), -80°C for long-term stability, and argon/vacuum sealing . Degradation is monitored via conjugated diene formation (UV absorbance at 234 nm) and compared to fresh standards . In kinetic studies, real-time oxidation is minimized by conducting assays under nitrogen atmospheres and using chelators (e.g., EDTA) to sequester metal catalysts .
Q. What advanced techniques differentiate this compound’s signaling roles in eicosanoid vs. non-eicosanoid pathways?
CRISPR-mediated knockout of 5-lipoxygenase (5-LOX) or cyclooxygenase-2 (COX-2) in cell lines isolates eicosanoid-specific effects. Non-eicosanoid roles (e.g., ion channel modulation) are studied using electrophysiology (patch-clamp) with this compound (20 mM) applied extracellularly to observe delayed rectifier K⁺ channel (Kv1.1) activation kinetics . Data are normalized to structurally similar esters (e.g., methyl oleate) to exclude nonspecific lipid interactions .
Eigenschaften
IUPAC Name |
methyl (5Z,8Z,11Z,14Z)-icosa-5,8,11,14-tetraenoate | |
---|---|---|
Source | PubChem | |
URL | https://pubchem.ncbi.nlm.nih.gov | |
Description | Data deposited in or computed by PubChem | |
InChI |
InChI=1S/C21H34O2/c1-3-4-5-6-7-8-9-10-11-12-13-14-15-16-17-18-19-20-21(22)23-2/h7-8,10-11,13-14,16-17H,3-6,9,12,15,18-20H2,1-2H3/b8-7-,11-10-,14-13-,17-16- | |
Source | PubChem | |
URL | https://pubchem.ncbi.nlm.nih.gov | |
Description | Data deposited in or computed by PubChem | |
InChI Key |
OFIDNKMQBYGNIW-ZKWNWVNESA-N | |
Source | PubChem | |
URL | https://pubchem.ncbi.nlm.nih.gov | |
Description | Data deposited in or computed by PubChem | |
Canonical SMILES |
CCCCCC=CCC=CCC=CCC=CCCCC(=O)OC | |
Source | PubChem | |
URL | https://pubchem.ncbi.nlm.nih.gov | |
Description | Data deposited in or computed by PubChem | |
Isomeric SMILES |
CCCCC/C=C\C/C=C\C/C=C\C/C=C\CCCC(=O)OC | |
Source | PubChem | |
URL | https://pubchem.ncbi.nlm.nih.gov | |
Description | Data deposited in or computed by PubChem | |
Molecular Formula |
C21H34O2 | |
Source | PubChem | |
URL | https://pubchem.ncbi.nlm.nih.gov | |
Description | Data deposited in or computed by PubChem | |
DSSTOX Substance ID |
DTXSID801015890 | |
Record name | Methyl arachidonate | |
Source | EPA DSSTox | |
URL | https://comptox.epa.gov/dashboard/DTXSID801015890 | |
Description | DSSTox provides a high quality public chemistry resource for supporting improved predictive toxicology. | |
Molecular Weight |
318.5 g/mol | |
Source | PubChem | |
URL | https://pubchem.ncbi.nlm.nih.gov | |
Description | Data deposited in or computed by PubChem | |
CAS No. |
2566-89-4 | |
Record name | Methyl arachidonate | |
Source | CAS Common Chemistry | |
URL | https://commonchemistry.cas.org/detail?cas_rn=2566-89-4 | |
Description | CAS Common Chemistry is an open community resource for accessing chemical information. Nearly 500,000 chemical substances from CAS REGISTRY cover areas of community interest, including common and frequently regulated chemicals, and those relevant to high school and undergraduate chemistry classes. This chemical information, curated by our expert scientists, is provided in alignment with our mission as a division of the American Chemical Society. | |
Explanation | The data from CAS Common Chemistry is provided under a CC-BY-NC 4.0 license, unless otherwise stated. | |
Record name | Methyl arachidonate | |
Source | ChemIDplus | |
URL | https://pubchem.ncbi.nlm.nih.gov/substance/?source=chemidplus&sourceid=0002566894 | |
Description | ChemIDplus is a free, web search system that provides access to the structure and nomenclature authority files used for the identification of chemical substances cited in National Library of Medicine (NLM) databases, including the TOXNET system. | |
Record name | Methyl arachidonate | |
Source | EPA DSSTox | |
URL | https://comptox.epa.gov/dashboard/DTXSID801015890 | |
Description | DSSTox provides a high quality public chemistry resource for supporting improved predictive toxicology. | |
Record name | Methyl (5Z,8Z,11Z,14Z)-icosa-5,8,11,14-tetraenoate | |
Source | European Chemicals Agency (ECHA) | |
URL | https://echa.europa.eu/substance-information/-/substanceinfo/100.018.092 | |
Description | The European Chemicals Agency (ECHA) is an agency of the European Union which is the driving force among regulatory authorities in implementing the EU's groundbreaking chemicals legislation for the benefit of human health and the environment as well as for innovation and competitiveness. | |
Explanation | Use of the information, documents and data from the ECHA website is subject to the terms and conditions of this Legal Notice, and subject to other binding limitations provided for under applicable law, the information, documents and data made available on the ECHA website may be reproduced, distributed and/or used, totally or in part, for non-commercial purposes provided that ECHA is acknowledged as the source: "Source: European Chemicals Agency, http://echa.europa.eu/". Such acknowledgement must be included in each copy of the material. ECHA permits and encourages organisations and individuals to create links to the ECHA website under the following cumulative conditions: Links can only be made to webpages that provide a link to the Legal Notice page. | |
Record name | METHYL ARACHIDONATE | |
Source | FDA Global Substance Registration System (GSRS) | |
URL | https://gsrs.ncats.nih.gov/ginas/app/beta/substances/22AF6IJ1IJ | |
Description | The FDA Global Substance Registration System (GSRS) enables the efficient and accurate exchange of information on what substances are in regulated products. Instead of relying on names, which vary across regulatory domains, countries, and regions, the GSRS knowledge base makes it possible for substances to be defined by standardized, scientific descriptions. | |
Explanation | Unless otherwise noted, the contents of the FDA website (www.fda.gov), both text and graphics, are not copyrighted. They are in the public domain and may be republished, reprinted and otherwise used freely by anyone without the need to obtain permission from FDA. Credit to the U.S. Food and Drug Administration as the source is appreciated but not required. | |
Retrosynthesis Analysis
AI-Powered Synthesis Planning: Our tool employs the Template_relevance Pistachio, Template_relevance Bkms_metabolic, Template_relevance Pistachio_ringbreaker, Template_relevance Reaxys, Template_relevance Reaxys_biocatalysis model, leveraging a vast database of chemical reactions to predict feasible synthetic routes.
One-Step Synthesis Focus: Specifically designed for one-step synthesis, it provides concise and direct routes for your target compounds, streamlining the synthesis process.
Accurate Predictions: Utilizing the extensive PISTACHIO, BKMS_METABOLIC, PISTACHIO_RINGBREAKER, REAXYS, REAXYS_BIOCATALYSIS database, our tool offers high-accuracy predictions, reflecting the latest in chemical research and data.
Strategy Settings
Precursor scoring | Relevance Heuristic |
---|---|
Min. plausibility | 0.01 |
Model | Template_relevance |
Template Set | Pistachio/Bkms_metabolic/Pistachio_ringbreaker/Reaxys/Reaxys_biocatalysis |
Top-N result to add to graph | 6 |
Feasible Synthetic Routes
Haftungsausschluss und Informationen zu In-Vitro-Forschungsprodukten
Bitte beachten Sie, dass alle Artikel und Produktinformationen, die auf BenchChem präsentiert werden, ausschließlich zu Informationszwecken bestimmt sind. Die auf BenchChem zum Kauf angebotenen Produkte sind speziell für In-vitro-Studien konzipiert, die außerhalb lebender Organismen durchgeführt werden. In-vitro-Studien, abgeleitet von dem lateinischen Begriff "in Glas", beinhalten Experimente, die in kontrollierten Laborumgebungen unter Verwendung von Zellen oder Geweben durchgeführt werden. Es ist wichtig zu beachten, dass diese Produkte nicht als Arzneimittel oder Medikamente eingestuft sind und keine Zulassung der FDA für die Vorbeugung, Behandlung oder Heilung von medizinischen Zuständen, Beschwerden oder Krankheiten erhalten haben. Wir müssen betonen, dass jede Form der körperlichen Einführung dieser Produkte in Menschen oder Tiere gesetzlich strikt untersagt ist. Es ist unerlässlich, sich an diese Richtlinien zu halten, um die Einhaltung rechtlicher und ethischer Standards in Forschung und Experiment zu gewährleisten.