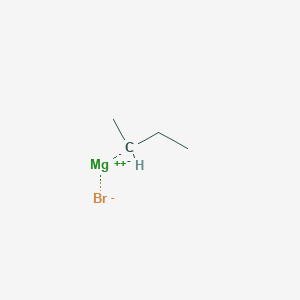
sek-Butylmagnesiumbromid
Übersicht
Beschreibung
Bromo(1-methylpropyl)magnesium is a useful research compound. Its molecular formula is C4H9BrMg and its molecular weight is 161.32 g/mol. The purity is usually 95%.
The exact mass of the compound Bromo(1-methylpropyl)magnesium is unknown and the complexity rating of the compound is unknown. The United Nations designated GHS hazard class pictogram is Flammable;Corrosive, and the GHS signal word is DangerThe storage condition is unknown. Please store according to label instructions upon receipt of goods.
BenchChem offers high-quality Bromo(1-methylpropyl)magnesium suitable for many research applications. Different packaging options are available to accommodate customers' requirements. Please inquire for more information about Bromo(1-methylpropyl)magnesium including the price, delivery time, and more detailed information at info@benchchem.com.
Wissenschaftliche Forschungsanwendungen
Organische Synthese
s-BuMgBr ist ein Grignard-Reagenz, das in der organischen Synthese eine zentrale Rolle spielt. Es fungiert als Nukleophil, das Kohlenstoff-Kohlenstoff-Bindungen bilden kann, wodurch die Synthese einer breiten Palette organischer Verbindungen ermöglicht wird. Beispielsweise wird es bei der Herstellung von Alkoholen, Carbonsäuren und anderen funktionellen Gruppen durch Reaktionen mit Carbonylverbindungen wie Aldehyden und Ketonen verwendet .
Pharmazeutische Zwischenprodukte
In der pharmazeutischen Industrie dient s-BuMgBr als wichtiges Zwischenprodukt bei der Synthese verschiedener Medikamente. Es ist an der Konstruktion komplexer Moleküle beteiligt, die als pharmazeutische Wirkstoffe (APIs) dienen. Dies beinhaltet die Synthese von Statinen, die bei der Kontrolle des Cholesterinspiegels eine wichtige Rolle spielen .
LED-Herstellung
Die Verbindung findet Anwendung bei der Herstellung von Leuchtdioden (LEDs). Sie wird verwendet, um dünne Schichten aus organischen Materialien abzuscheiden, die Licht emittieren, wenn ein elektrischer Strom durch sie geleitet wird. Dieser Prozess ist für die Herstellung hocheffizienter und langlebiger LEDs unerlässlich .
Dünnschichtdeposition
s-BuMgBr wird bei Dünnschichtdepositionstechniken wie der chemischen Gasphasenabscheidung (CVD) eingesetzt. Diese dünnen Schichten sind in verschiedenen Technologien, darunter Solarzellen, von entscheidender Bedeutung, wo sie die Effizienz der Lichtabsorption und der Energieumwandlung verbessern .
Industrielle Chemie
In der industriellen Chemie wird s-BuMgBr als Katalysator und Reagenz für verschiedene chemische Reaktionen eingesetzt. Seine Rolle in der Katalyse ist für großtechnische Produktionsprozesse von Bedeutung, insbesondere bei der Herstellung von Polymeren und anderen Makromolekülen .
Materialwissenschaft
Forscher in der Materialwissenschaft verwenden s-BuMgBr zur Synthese neuer Materialien mit einzigartigen Eigenschaften. Es ist bei der Herstellung von magnesiumhaltigen Verbindungen von Bedeutung, die auf ihr Potenzial als leichtgewichtige und hochfeste Materialien untersucht werden .
Agrochemische Synthese
s-BuMgBr wird auch bei der Synthese von Agrochemikalien verwendet. Es trägt zur Herstellung von Verbindungen bei, die als Pestizide oder Düngemittel dienen und zur Entwicklung effizienterer und umweltfreundlicherer landwirtschaftlicher Produkte beitragen .
Polymerisationsreaktionen
Schließlich kann s-BuMgBr als Initiator in Ringöffnungs-Polymerisationsreaktionen wirken. Diese Anwendung ist entscheidend für die Herstellung spezifischer Polymertypen mit gewünschten Eigenschaften für den Einsatz in Medizinprodukten, Verpackungen und biologisch abbaubaren Materialien .
Wirkmechanismus
Target of Action
Sec-Butylmagnesium Bromide is a Grignard reagent . Grignard reagents are organometallic compounds that are primarily used as reagents, catalysts, and precursor materials in various chemical reactions . They are typically used in reactions with carbonyl groups, where they act as nucleophiles .
Mode of Action
Sec-Butylmagnesium Bromide, like other Grignard reagents, is highly reactive. It can readily react with a variety of electrophilic compounds, particularly carbonyl compounds, to form new carbon-carbon bonds . This makes it a valuable tool in the synthesis of a wide range of organic compounds.
Biochemical Pathways
The primary biochemical pathway involving sec-Butylmagnesium Bromide is the Grignard reaction . In this reaction, the Grignard reagent acts as a nucleophile, attacking the electrophilic carbon atom of a carbonyl group. This results in the formation of a new carbon-carbon bond, leading to the synthesis of alcohols, ketones, aldehydes, and other organic compounds .
Result of Action
The primary result of the action of sec-Butylmagnesium Bromide is the formation of new organic compounds through the creation of new carbon-carbon bonds . This enables the synthesis of a wide range of organic compounds, including complex molecules for pharmaceuticals and other applications .
Action Environment
The action of sec-Butylmagnesium Bromide is highly dependent on the environment. It is typically used in anhydrous conditions, as it reacts vigorously with water . It also requires a polar aprotic solvent, such as diethyl ether, for the Grignard reaction to proceed . Temperature can also affect the reaction, with lower temperatures often used to control the rate of the reaction .
Eigenschaften
IUPAC Name |
magnesium;butane;bromide | |
---|---|---|
Details | Computed by Lexichem TK 2.7.0 (PubChem release 2021.05.07) | |
Source | PubChem | |
URL | https://pubchem.ncbi.nlm.nih.gov | |
Description | Data deposited in or computed by PubChem | |
InChI |
InChI=1S/C4H9.BrH.Mg/c1-3-4-2;;/h3H,4H2,1-2H3;1H;/q-1;;+2/p-1 | |
Details | Computed by InChI 1.0.6 (PubChem release 2021.05.07) | |
Source | PubChem | |
URL | https://pubchem.ncbi.nlm.nih.gov | |
Description | Data deposited in or computed by PubChem | |
InChI Key |
WSHFRLGXCNEKRX-UHFFFAOYSA-M | |
Details | Computed by InChI 1.0.6 (PubChem release 2021.05.07) | |
Source | PubChem | |
URL | https://pubchem.ncbi.nlm.nih.gov | |
Description | Data deposited in or computed by PubChem | |
Canonical SMILES |
CC[CH-]C.[Mg+2].[Br-] | |
Details | Computed by OEChem 2.3.0 (PubChem release 2021.05.07) | |
Source | PubChem | |
URL | https://pubchem.ncbi.nlm.nih.gov | |
Description | Data deposited in or computed by PubChem | |
Molecular Formula |
C4H9BrMg | |
Details | Computed by PubChem 2.1 (PubChem release 2021.05.07) | |
Source | PubChem | |
URL | https://pubchem.ncbi.nlm.nih.gov | |
Description | Data deposited in or computed by PubChem | |
DSSTOX Substance ID |
DTXSID20919307 | |
Record name | Magnesium bromide butan-2-ide (1/1/1) | |
Source | EPA DSSTox | |
URL | https://comptox.epa.gov/dashboard/DTXSID20919307 | |
Description | DSSTox provides a high quality public chemistry resource for supporting improved predictive toxicology. | |
Molecular Weight |
161.32 g/mol | |
Details | Computed by PubChem 2.1 (PubChem release 2021.05.07) | |
Source | PubChem | |
URL | https://pubchem.ncbi.nlm.nih.gov | |
Description | Data deposited in or computed by PubChem | |
CAS No. |
922-66-7 | |
Record name | Magnesium bromide butan-2-ide (1/1/1) | |
Source | EPA DSSTox | |
URL | https://comptox.epa.gov/dashboard/DTXSID20919307 | |
Description | DSSTox provides a high quality public chemistry resource for supporting improved predictive toxicology. | |
Q1: How does the steric bulk of the alkyl group in Grignard reagents influence the polymerization of methyl methacrylate?
A1: Research indicates that increasing the steric bulk of the alkyl group in Grignard reagents significantly impacts the polymerization of methyl methacrylate []. Specifically, bulkier alkyl groups, like sec-butyl, lead to higher initiation efficiency and overall polymer yield. Additionally, the resulting polymers tend to exhibit narrower molecular weight distributions []. This observation can be attributed to the reduced occurrence of side reactions during the polymerization initiation stage when bulkier Grignard reagents are used.
Q2: Can sec-butylmagnesium bromide be used to synthesize syndiotactic poly(methyl methacrylate) with high stereoregularity?
A2: Yes, sec-butylmagnesium bromide has proven effective in achieving high syndiotacticity in poly(methyl methacrylate) synthesis []. When polymerization is conducted at low temperatures (-110°C) in a tetrahydrofuran-dimethoxyethane solvent mixture, using sec-butylmagnesium bromide as the initiator, the resulting polymer exhibits a high syndiotactic triad content (94.9%) and a narrow molecular weight distribution (Mw/Mn = 1.39) [].
Q3: Beyond polymerization, what other synthetic applications utilize sec-butylmagnesium bromide?
A3: sec-Butylmagnesium bromide acts as a valuable reagent in various synthetic transformations. One example is its use in the preparation of deuterium-labeled aldehydes. Specifically, it reacts with N-(1,1,3,3-tetramethylbutyl)formamide to yield 2-methylbutanal-1-d, a deuterated aldehyde, through a multi-step process involving isonitrile formation and hydrolysis [].
Q4: Are there any examples of sec-butylmagnesium bromide participating in transition-metal-catalyzed reactions?
A4: Research demonstrates the utility of sec-butylmagnesium bromide in iron-catalyzed cross-coupling reactions []. This Grignard reagent reacts with 3-chloroprop-2-en-1-ylamines in the presence of an iron catalyst, offering a novel route for the construction of complex amine derivatives.
Haftungsausschluss und Informationen zu In-Vitro-Forschungsprodukten
Bitte beachten Sie, dass alle Artikel und Produktinformationen, die auf BenchChem präsentiert werden, ausschließlich zu Informationszwecken bestimmt sind. Die auf BenchChem zum Kauf angebotenen Produkte sind speziell für In-vitro-Studien konzipiert, die außerhalb lebender Organismen durchgeführt werden. In-vitro-Studien, abgeleitet von dem lateinischen Begriff "in Glas", beinhalten Experimente, die in kontrollierten Laborumgebungen unter Verwendung von Zellen oder Geweben durchgeführt werden. Es ist wichtig zu beachten, dass diese Produkte nicht als Arzneimittel oder Medikamente eingestuft sind und keine Zulassung der FDA für die Vorbeugung, Behandlung oder Heilung von medizinischen Zuständen, Beschwerden oder Krankheiten erhalten haben. Wir müssen betonen, dass jede Form der körperlichen Einführung dieser Produkte in Menschen oder Tiere gesetzlich strikt untersagt ist. Es ist unerlässlich, sich an diese Richtlinien zu halten, um die Einhaltung rechtlicher und ethischer Standards in Forschung und Experiment zu gewährleisten.