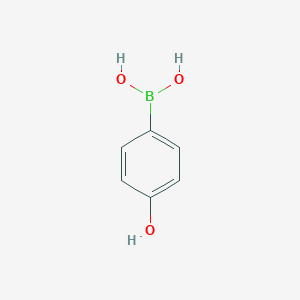
4-Hydroxyphenylboronsäure
Übersicht
Beschreibung
4-Hydroxyphenylboronic acid: is an organic compound with the molecular formula C6H7BO3 . It is a derivative of phenylboronic acid, where a hydroxyl group is attached to the para position of the phenyl ring. This compound is widely used in organic synthesis, particularly in Suzuki-Miyaura coupling reactions, due to its ability to form stable carbon-boron bonds.
Wissenschaftliche Forschungsanwendungen
Chemistry: 4-Hydroxyphenylboronic acid is extensively used in organic synthesis, particularly in the formation of carbon-carbon and carbon-nitrogen bonds. It is a key reagent in the Suzuki-Miyaura coupling reaction, which is widely used in the synthesis of pharmaceuticals, agrochemicals, and organic materials .
Biology and Medicine: This compound has shown potential in the development of enzyme inhibitors for treating Gram-negative bacterial infections. It is also used in the synthesis of cyclopamine analogs, which are inhibitors of the Sonic Hedgehog signaling pathway and have applications in cancer treatment .
Industry: In the industrial sector, 4-hydroxyphenylboronic acid is used to prepare bio-supported palladium nanoparticles, which serve as phosphine-free catalysts in various chemical reactions. It is also employed in the synthesis of rod-like dendronized polymers, which have applications in materials science .
Wirkmechanismus
Target of Action
The primary target of 4-Hydroxyphenylboronic acid is PDK1 , a kinase involved in cell growth, survival, and tumorigenesis . By inhibiting PDK1, 4-Hydroxyphenylboronic acid can potentially suppress cancer cell growth and survival .
Mode of Action
4-Hydroxyphenylboronic acid interacts with its target, PDK1, by binding to it and inhibiting its activity . This interaction results in the suppression of downstream signaling pathways that are crucial for cancer cell growth and survival .
Biochemical Pathways
The inhibition of PDK1 by 4-Hydroxyphenylboronic acid affects various biochemical pathways. These include the PI3K/Akt/mTOR pathway, which is involved in cell survival and growth . By inhibiting PDK1, these pathways are downregulated, leading to reduced cell growth and survival .
Result of Action
The result of 4-Hydroxyphenylboronic acid’s action is the inhibition of PDK1, leading to the suppression of downstream signaling pathways . This results in reduced cell growth and survival, particularly in cancer cells .
Action Environment
The action of 4-Hydroxyphenylboronic acid can be influenced by various environmental factors. For instance, the pH of the environment can affect the stability of the compound . Additionally, the presence of other compounds or drugs can potentially affect the efficacy of 4-Hydroxyphenylboronic acid through drug-drug interactions.
Biochemische Analyse
Biochemical Properties
4-Hydroxyphenylboronic acid plays a significant role in biochemical reactions. It is used as a reactant in Suzuki-Miyaura coupling and Stille coupling reactions . It also participates in palladium-catalyzed aminocarbonylation and cross-coupling reactions .
Cellular Effects
It has been shown to be effective in lipopolysaccharide (LPS) induced cell death .
Molecular Mechanism
The molecular mechanism of 4-Hydroxyphenylboronic acid involves its interaction with biomolecules during Suzuki-Miyaura coupling and Stille coupling reactions . It also participates in palladium-catalyzed aminocarbonylation and cross-coupling reactions .
Temporal Effects in Laboratory Settings
Vorbereitungsmethoden
Synthetic Routes and Reaction Conditions:
Starting from Bromophenol: One common method involves starting with 4-bromophenol. The hydroxyl group is protected using tert-butyldimethylsilyl, followed by the formation of a Grignard reagent.
Using p-Methoxyphenylboronic Acid: Another method involves the reaction of p-methoxyphenylboronic acid with acetyl chloride and aluminum chloride in toluene.
Industrial Production Methods: The industrial production of 4-hydroxyphenylboronic acid typically involves large-scale batch processes. The use of cheap and easily removable protecting groups during synthesis makes it feasible for industrial amplification. The process has been scaled up to produce dozens of kilograms with good process stability .
Analyse Chemischer Reaktionen
Types of Reactions:
Suzuki-Miyaura Coupling: This reaction involves the cross-coupling of 4-hydroxyphenylboronic acid with halides or triflates in the presence of a palladium catalyst and a base.
Stille Coupling: Similar to Suzuki-Miyaura coupling, this reaction uses a tin reagent instead of a boronic acid.
Aminocarbonylation and Cross-Coupling: These reactions involve the use of palladium catalysts to form carbon-nitrogen bonds, which are essential in the synthesis of various pharmaceuticals.
Common Reagents and Conditions:
Palladium Catalysts: Used in both Suzuki-Miyaura and Stille coupling reactions.
Bases: Such as potassium carbonate or sodium hydroxide, are commonly used to facilitate the reactions.
Solvents: Toluene, acetonitrile, and dimethyl sulfoxide are frequently used solvents.
Major Products:
Biaryl Compounds: Formed through Suzuki-Miyaura coupling.
Carbon-Nitrogen Bonded Compounds: Resulting from aminocarbonylation reactions.
Vergleich Mit ähnlichen Verbindungen
Phenylboronic Acid: Lacks the hydroxyl group, making it less reactive in certain coupling reactions.
4-Methoxyphenylboronic Acid: Contains a methoxy group instead of a hydroxyl group, which can influence its reactivity and selectivity in chemical reactions.
4-Aminophenylboronic Acid: Contains an amino group, which can participate in different types of reactions compared to the hydroxyl group.
Uniqueness: 4-Hydroxyphenylboronic acid is unique due to the presence of the hydroxyl group, which enhances its reactivity in various coupling reactions. This functional group also allows for additional modifications, making it a versatile reagent in organic synthesis .
Biologische Aktivität
4-Hydroxyphenylboronic acid (4-HBA) is a compound that has garnered attention for its diverse biological activities, particularly in the fields of cancer research and endothelial function. This article provides a comprehensive overview of the biological activity of 4-HBA, including its mechanisms of action, effects on various cell types, and potential therapeutic applications.
4-Hydroxyphenylboronic acid is a phenolic compound with the chemical formula CHBO. It contains a boronic acid functional group, which is known to form reversible covalent bonds with diols and has implications in biological systems, particularly in the modulation of protein interactions.
Protein Binding and Interaction
One of the key aspects of 4-HBA's biological activity is its interaction with proteins, particularly human serum albumin (HSA). Studies have shown that 4-HBA binds to HSA through static and dynamic quenching mechanisms, which are influenced by temperature and pH conditions. The binding affinity is attributed to hydrogen bonding and Van der Waals forces, which are crucial for its pharmacological effects .
In Vitro Studies
Research has demonstrated that 4-HBA exhibits significant anticancer properties. In studies involving doxorubicin-sensitive K562 leukemia cells and doxorubicin-resistant K562/Dox cells, 4-HBA was found to decrease cell viability in a dose- and time-dependent manner. For instance, treatment with 10 mM of 4-HBA resulted in cell viability percentages of approximately 90% at 72 hours for K562 cells and about 66% at 96 hours for K562/Dox cells .
Table 1: Effects of 4-Hydroxyphenylboronic Acid on Cell Viability
Concentration (mM) | K562 Cell Viability (%) | K562/Dox Cell Viability (%) |
---|---|---|
0 | 100 | 100 |
0.01 | ~95 | ~95 |
0.1 | ~90 | ~80 |
1 | ~85 | ~70 |
10 | ~90 (72h) | ~66 (96h) |
These findings suggest that the compound may enhance the efficacy of existing chemotherapy agents by overcoming resistance mechanisms in cancer cells.
LPS-Induced Damage Studies
Another significant aspect of 4-HBA's biological activity is its protective effect against lipopolysaccharide (LPS)-induced endothelial damage. In vitro studies using human umbilical vein endothelial cells (HUVECs) demonstrated that treatment with various concentrations of 4-HBA significantly reduced markers of endothelial damage, such as endocan levels, compared to untreated LPS groups. For instance, at a concentration of 62.5 µM, endocan levels were markedly lower than those in the LPS group, indicating a protective role against inflammation-induced cell death .
Table 2: Effects of 4-Hydroxyphenylboronic Acid on Endothelial Damage
Concentration (µM) | Endocan Level Reduction (%) |
---|---|
Control | - |
LPS Group | +50 (increase) |
31.25 | -20 |
62.5 | -45 |
125 | -30 |
250 | -25 |
These results highlight the potential of 4-HBA as a therapeutic agent in conditions characterized by endothelial dysfunction, such as sepsis.
Eigenschaften
IUPAC Name |
(4-hydroxyphenyl)boronic acid | |
---|---|---|
Source | PubChem | |
URL | https://pubchem.ncbi.nlm.nih.gov | |
Description | Data deposited in or computed by PubChem | |
InChI |
InChI=1S/C6H7BO3/c8-6-3-1-5(2-4-6)7(9)10/h1-4,8-10H | |
Source | PubChem | |
URL | https://pubchem.ncbi.nlm.nih.gov | |
Description | Data deposited in or computed by PubChem | |
InChI Key |
COIQUVGFTILYGA-UHFFFAOYSA-N | |
Source | PubChem | |
URL | https://pubchem.ncbi.nlm.nih.gov | |
Description | Data deposited in or computed by PubChem | |
Canonical SMILES |
B(C1=CC=C(C=C1)O)(O)O | |
Source | PubChem | |
URL | https://pubchem.ncbi.nlm.nih.gov | |
Description | Data deposited in or computed by PubChem | |
Molecular Formula |
C6H7BO3 | |
Source | PubChem | |
URL | https://pubchem.ncbi.nlm.nih.gov | |
Description | Data deposited in or computed by PubChem | |
DSSTOX Substance ID |
DTXSID00370250 | |
Record name | 4-Hydroxyphenylboronic acid | |
Source | EPA DSSTox | |
URL | https://comptox.epa.gov/dashboard/DTXSID00370250 | |
Description | DSSTox provides a high quality public chemistry resource for supporting improved predictive toxicology. | |
Molecular Weight |
137.93 g/mol | |
Source | PubChem | |
URL | https://pubchem.ncbi.nlm.nih.gov | |
Description | Data deposited in or computed by PubChem | |
CAS No. |
71597-85-8 | |
Record name | (4-Hydroxyphenyl)boronic acid | |
Source | CAS Common Chemistry | |
URL | https://commonchemistry.cas.org/detail?cas_rn=71597-85-8 | |
Description | CAS Common Chemistry is an open community resource for accessing chemical information. Nearly 500,000 chemical substances from CAS REGISTRY cover areas of community interest, including common and frequently regulated chemicals, and those relevant to high school and undergraduate chemistry classes. This chemical information, curated by our expert scientists, is provided in alignment with our mission as a division of the American Chemical Society. | |
Explanation | The data from CAS Common Chemistry is provided under a CC-BY-NC 4.0 license, unless otherwise stated. | |
Record name | 4-Hydroxyphenylboronic acid | |
Source | EPA DSSTox | |
URL | https://comptox.epa.gov/dashboard/DTXSID00370250 | |
Description | DSSTox provides a high quality public chemistry resource for supporting improved predictive toxicology. | |
Retrosynthesis Analysis
AI-Powered Synthesis Planning: Our tool employs the Template_relevance Pistachio, Template_relevance Bkms_metabolic, Template_relevance Pistachio_ringbreaker, Template_relevance Reaxys, Template_relevance Reaxys_biocatalysis model, leveraging a vast database of chemical reactions to predict feasible synthetic routes.
One-Step Synthesis Focus: Specifically designed for one-step synthesis, it provides concise and direct routes for your target compounds, streamlining the synthesis process.
Accurate Predictions: Utilizing the extensive PISTACHIO, BKMS_METABOLIC, PISTACHIO_RINGBREAKER, REAXYS, REAXYS_BIOCATALYSIS database, our tool offers high-accuracy predictions, reflecting the latest in chemical research and data.
Strategy Settings
Precursor scoring | Relevance Heuristic |
---|---|
Min. plausibility | 0.01 |
Model | Template_relevance |
Template Set | Pistachio/Bkms_metabolic/Pistachio_ringbreaker/Reaxys/Reaxys_biocatalysis |
Top-N result to add to graph | 6 |
Feasible Synthetic Routes
Haftungsausschluss und Informationen zu In-Vitro-Forschungsprodukten
Bitte beachten Sie, dass alle Artikel und Produktinformationen, die auf BenchChem präsentiert werden, ausschließlich zu Informationszwecken bestimmt sind. Die auf BenchChem zum Kauf angebotenen Produkte sind speziell für In-vitro-Studien konzipiert, die außerhalb lebender Organismen durchgeführt werden. In-vitro-Studien, abgeleitet von dem lateinischen Begriff "in Glas", beinhalten Experimente, die in kontrollierten Laborumgebungen unter Verwendung von Zellen oder Geweben durchgeführt werden. Es ist wichtig zu beachten, dass diese Produkte nicht als Arzneimittel oder Medikamente eingestuft sind und keine Zulassung der FDA für die Vorbeugung, Behandlung oder Heilung von medizinischen Zuständen, Beschwerden oder Krankheiten erhalten haben. Wir müssen betonen, dass jede Form der körperlichen Einführung dieser Produkte in Menschen oder Tiere gesetzlich strikt untersagt ist. Es ist unerlässlich, sich an diese Richtlinien zu halten, um die Einhaltung rechtlicher und ethischer Standards in Forschung und Experiment zu gewährleisten.