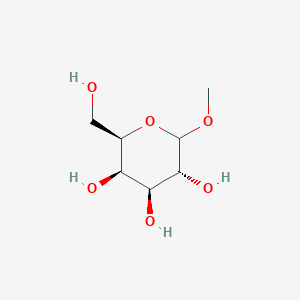
(2R,3R,4S,5R)-2-(Hydroxymethyl)-6-methoxytetrahydro-2H-pyran-3,4,5-triol
Übersicht
Beschreibung
(2R,3R,4S,5R)-2-(Hydroxymethyl)-6-methoxytetrahydro-2H-pyran-3,4,5-triol, also known as 2,3,4,5-tetrahydroxy-6-methoxy-2H-pyran-2-methanol, is a naturally occurring polyhydroxy alcohol (polyol) found in some plant species. It is an important intermediate in the synthesis of many complex natural products and has been used in a variety of pharmaceuticals, cosmetics, and food products. The compound has attracted significant attention due to its potential applications in the synthesis of drugs, as well as its ability to act as a potent antioxidant.
Wissenschaftliche Forschungsanwendungen
Applications in Green Chemistry and Biomass Conversion
One significant application of structurally related compounds is in the field of green chemistry, particularly in the conversion of plant biomass to valuable chemicals. A study by Chernyshev et al. (2017) highlights the importance of furan derivatives, such as 5-Hydroxymethylfurfural (HMF), in replacing non-renewable sources of hydrocarbons. These derivatives, including those structurally similar to the mentioned compound, are pivotal in producing monomers, polymers, and other functional materials from plant feedstocks, showcasing the potential of such compounds in sustainable chemistry and industry applications Chernyshev, Kravchenko, & Ananikov, 2017.
Pharmaceutical Applications
In the pharmaceutical sector, compounds with similar structures have been explored for their bioactivity. For example, derivatives of pyran, which is a core structural component of the compound , have been studied for their applications in medicinal chemistry. Parmar et al. (2023) discuss the importance of pyranopyrimidine scaffolds, derived from similar structural frameworks, in drug development due to their broad synthetic applications and bioavailability Parmar, Vala, & Patel, 2023.
Analytical Applications
Moreover, the compound's structural relatives have been utilized in developing sophisticated analytical methods for pharmaceuticals. Danao (2021) reviews analytical techniques for the determination of the anti-diabetic drug Empagliflozin, showcasing the role of complex molecules in enhancing the quality and reliability of pharmaceutical analysis Danao, 2021.
Material Science Applications
Furthermore, related compounds have found applications in materials science, particularly in improving the electrical conductivity of polymers. Shi et al. (2015) review methods to enhance the electrical conductivity of PEDOT:PSS, a conducting polymer, for applications in organic electronic devices. This indicates the potential utility of structurally similar compounds in modifying and improving the properties of materials for electronic applications Shi, Liu, Jiang, & Xu, 2015.
Biochemische Analyse
Biochemical Properties
Methyl D-galactopyranoside plays a crucial role in biochemical reactions. It interacts with various enzymes and proteins. For instance, it has been found to be a potent inhibitor against the Debaryomyces hansenii UFV-1 extracellular and intracellular α-galactosidases . The nature of these interactions is often complex and involves multiple biochemical pathways.
Cellular Effects
The effects of Methyl D-galactopyranoside on various types of cells and cellular processes are diverse. It influences cell function, including any impact on cell signaling pathways, gene expression, and cellular metabolism. For example, Methyl D-galactopyranoside esters have been studied as potential inhibitors for the SARS-CoV-2 protease enzyme .
Molecular Mechanism
Methyl D-galactopyranoside exerts its effects at the molecular level through various mechanisms. These include binding interactions with biomolecules, enzyme inhibition or activation, and changes in gene expression. For instance, molecular docking studies have shown that Methyl D-galactopyranoside esters strongly interact with the prime Cys145, His41, MET165, GLY143, THR26, and ASN142 residues of the SARS-CoV-2 main protease .
Metabolic Pathways
Methyl D-galactopyranoside is involved in various metabolic pathways. It interacts with enzymes or cofactors and can affect metabolic flux or metabolite levels. For instance, Methyl D-galactopyranoside is a hexose involved in the metabolism of 2-deoxygalactose .
Eigenschaften
IUPAC Name |
(2R,3R,4S,5R)-2-(hydroxymethyl)-6-methoxyoxane-3,4,5-triol | |
---|---|---|
Source | PubChem | |
URL | https://pubchem.ncbi.nlm.nih.gov | |
Description | Data deposited in or computed by PubChem | |
InChI |
InChI=1S/C7H14O6/c1-12-7-6(11)5(10)4(9)3(2-8)13-7/h3-11H,2H2,1H3/t3-,4+,5+,6-,7?/m1/s1 | |
Source | PubChem | |
URL | https://pubchem.ncbi.nlm.nih.gov | |
Description | Data deposited in or computed by PubChem | |
InChI Key |
HOVAGTYPODGVJG-TVPFVARWSA-N | |
Source | PubChem | |
URL | https://pubchem.ncbi.nlm.nih.gov | |
Description | Data deposited in or computed by PubChem | |
Canonical SMILES |
COC1C(C(C(C(O1)CO)O)O)O | |
Source | PubChem | |
URL | https://pubchem.ncbi.nlm.nih.gov | |
Description | Data deposited in or computed by PubChem | |
Isomeric SMILES |
COC1[C@@H]([C@H]([C@H]([C@H](O1)CO)O)O)O | |
Source | PubChem | |
URL | https://pubchem.ncbi.nlm.nih.gov | |
Description | Data deposited in or computed by PubChem | |
Molecular Formula |
C7H14O6 | |
Source | PubChem | |
URL | https://pubchem.ncbi.nlm.nih.gov | |
Description | Data deposited in or computed by PubChem | |
DSSTOX Substance ID |
DTXSID00472662 | |
Record name | Methyl D-galactopyranoside | |
Source | EPA DSSTox | |
URL | https://comptox.epa.gov/dashboard/DTXSID00472662 | |
Description | DSSTox provides a high quality public chemistry resource for supporting improved predictive toxicology. | |
Molecular Weight |
194.18 g/mol | |
Source | PubChem | |
URL | https://pubchem.ncbi.nlm.nih.gov | |
Description | Data deposited in or computed by PubChem | |
CAS RN |
93302-26-2 | |
Record name | Methyl D-galactopyranoside | |
Source | EPA DSSTox | |
URL | https://comptox.epa.gov/dashboard/DTXSID00472662 | |
Description | DSSTox provides a high quality public chemistry resource for supporting improved predictive toxicology. | |
Q & A
Q1: How does Methyl D-galactopyranoside interact with lectins?
A1: Methyl D-galactopyranoside acts as an inhibitor for certain lectins, specifically those with an affinity for galactose. It binds to the carbohydrate recognition domain (CRD) of the lectin, competing with natural ligands like galactose and potentially disrupting lectin-mediated biological processes. [, , , ]
Q2: What is the significance of Methyl D-galactopyranoside's interaction with the lactose permease of Escherichia coli?
A2: This interaction provides insights into the substrate specificity of the lactose permease. Studies have shown that the C-2, C-3, C-4, and C-6 hydroxyl groups of Methyl D-galactopyranoside are crucial for binding to the permease. This interaction is driven by hydrogen bonding and suggests that the cyclic pyranose conformation is the recognized form. []
Q3: What is the molecular formula and weight of Methyl D-galactopyranoside?
A3: The molecular formula is C7H14O6, and the molecular weight is 194.18 g/mol. []
Q4: Which spectroscopic techniques are useful for characterizing Methyl D-galactopyranoside?
A4: Nuclear Magnetic Resonance (NMR) spectroscopy, Infrared (IR) spectroscopy, and Mass Spectrometry (MS) are commonly employed to characterize Methyl D-galactopyranoside. [, , , , ]
Q5: Has Methyl D-galactopyranoside been investigated for catalytic applications?
A5: While Methyl D-galactopyranoside itself might not be catalytically active, its derivatives, particularly those with modifications at the C-3 position, have been explored as potential inhibitors for galectin-8. []
Q6: How have computational methods been applied in research involving Methyl D-galactopyranoside?
A6: Ab initio molecular dynamics (AIMD) simulations and vibrational self-consistent field (VSCF) calculations have been used to study the conformational dynamics and vibrational spectra of protonated forms of Methyl D-galactopyranoside. These methods contribute to understanding its reactivity and interactions in biological systems. []
Q7: How do structural modifications of Methyl D-galactopyranoside impact its interactions with lectins?
A7: Modifications at the C-1, C-2, C-3, C-4, and C-6 positions can significantly affect binding affinity to lectins. For instance, the presence and orientation of the C-1 substituent play a role in the binding affinity to the lactose permease. [, ]
Q8: Are there specific formulation strategies to enhance the stability or bioavailability of Methyl D-galactopyranoside?
A8: While the provided research does not delve into specific formulation strategies for Methyl D-galactopyranoside, its stability and solubility characteristics would inform such development. Common approaches like encapsulation, complexation, or the use of suitable excipients could be explored. []
Q9: Are there specific SHE (Safety, Health, and Environment) regulations concerning Methyl D-galactopyranoside?
A9: Specific SHE regulations might vary depending on the application and geographical location. Researchers should consult relevant safety data sheets and regulatory guidelines for handling and disposal. []
Haftungsausschluss und Informationen zu In-Vitro-Forschungsprodukten
Bitte beachten Sie, dass alle Artikel und Produktinformationen, die auf BenchChem präsentiert werden, ausschließlich zu Informationszwecken bestimmt sind. Die auf BenchChem zum Kauf angebotenen Produkte sind speziell für In-vitro-Studien konzipiert, die außerhalb lebender Organismen durchgeführt werden. In-vitro-Studien, abgeleitet von dem lateinischen Begriff "in Glas", beinhalten Experimente, die in kontrollierten Laborumgebungen unter Verwendung von Zellen oder Geweben durchgeführt werden. Es ist wichtig zu beachten, dass diese Produkte nicht als Arzneimittel oder Medikamente eingestuft sind und keine Zulassung der FDA für die Vorbeugung, Behandlung oder Heilung von medizinischen Zuständen, Beschwerden oder Krankheiten erhalten haben. Wir müssen betonen, dass jede Form der körperlichen Einführung dieser Produkte in Menschen oder Tiere gesetzlich strikt untersagt ist. Es ist unerlässlich, sich an diese Richtlinien zu halten, um die Einhaltung rechtlicher und ethischer Standards in Forschung und Experiment zu gewährleisten.