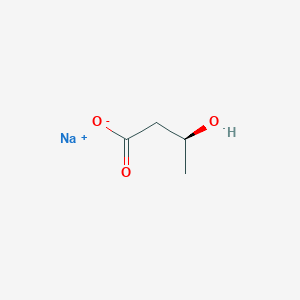
Sodium (S)-3-hydroxybutanoate
Übersicht
Beschreibung
Casopitant-Mesylat: ist ein Neurokinin-1-Rezeptor-Antagonist, der auf sein Potenzial untersucht wurde, Chemotherapie-induzierte Übelkeit und Erbrechen zu verhindern. Es wurde ursprünglich von GlaxoSmithKline entwickelt und hat in klinischen Studien vielversprechende Ergebnisse hinsichtlich seiner Wirksamkeit bei der Reduzierung von Übelkeit und Erbrechen bei Patienten gezeigt, die sich einer stark emetogenen Chemotherapie unterziehen .
Herstellungsmethoden
Synthesewege und Reaktionsbedingungen: : Die Synthese von Casopitant-Mesylat umfasst mehrere Schritte, darunter die Bildung des Piperidinrings und die Einführung verschiedener funktioneller Gruppen. Die wichtigsten Schritte umfassen:
- Bildung des Piperidinrings.
- Einführung der Trifluormethylgruppen.
- Kopplung des Piperidinderivats mit den entsprechenden Phenylgruppen.
- Bildung des Mesylat-Salzes.
Industrielle Produktionsmethoden: : Die industrielle Produktion von Casopitant-Mesylat folgt einem ähnlichen Syntheseweg, ist aber für die großtechnische Produktion optimiert. Dies beinhaltet die Verwendung von Reaktionen mit hoher Ausbeute, effizienten Reinigungsmethoden und strengen Qualitätskontrollmaßnahmen, um die Konsistenz und Reinheit des Endprodukts zu gewährleisten .
Wissenschaftliche Forschungsanwendungen
Chemie: Als Modellverbindung zur Untersuchung von Neurokinin-1-Rezeptor-Antagonisten verwendet.
Biologie: Untersucht auf seine Auswirkungen auf Neurokinin-1-Rezeptoren in verschiedenen biologischen Systemen.
Medizin: Hauptsächlich auf sein Potenzial untersucht, Chemotherapie-induzierte Übelkeit und Erbrechen zu verhindern. Es wurde auch auf sein Potenzial zur Behandlung anderer Erkrankungen wie Angstzustände und Depressionen untersucht.
Industrie: In der Entwicklung von Antiemetika-Formulierungen und als Referenzverbindung in der pharmazeutischen Forschung verwendet .
Wirkmechanismus
Casopitant-Mesylat entfaltet seine Wirkung durch Antagonisierung des Neurokinin-1-Rezeptors, der an der emetischen Reaktion beteiligt ist. Durch die Blockierung dieses Rezeptors verhindert Casopitant-Mesylat die Bindung von Substanz P, einem Neuropeptid, das Übelkeit und Erbrechen auslöst. Dieser Mechanismus macht es effektiv bei der Reduzierung von Chemotherapie-induzierter Übelkeit und Erbrechen .
Wirkmechanismus
Target of Action
Sodium (S)-3-hydroxybutanoate primarily targets voltage-gated sodium channels (Nav) in the body . These channels control the initiation and propagation of action potential, mediating a broad spectrum of physiological processes, including central and peripheral nervous systems’ function, skeletal muscle contraction, and heart rhythm .
Mode of Action
This compound interacts with its targets by altering the sodium currents. When the cell membrane is depolarized by a few millivolts, sodium channels activate and inactivate within milliseconds. The influx of sodium ions through the integral membrane proteins comprising the channel depolarizes the membrane further and initiates the rising phase of the action potential .
Biochemical Pathways
The compound affects several biochemical pathways. The primary pathway is the action potential pathway, where the compound influences the opening and closing of sodium channels, thus affecting the propagation of action potentials . .
Result of Action
The primary result of this compound’s action is the generation of action potentials in neurons and other electrically excitable cells such as myocytes and endocrine cells . This leads to various physiological responses, including muscle contraction and the regulation of heart rhythm .
Vorbereitungsmethoden
Synthetic Routes and Reaction Conditions: : The synthesis of casopitant mesylate involves multiple steps, including the formation of the piperidine ring and the introduction of various functional groups. The key steps include:
- Formation of the piperidine ring.
- Introduction of the trifluoromethyl groups.
- Coupling of the piperidine derivative with the appropriate phenyl groups.
- Formation of the mesylate salt.
Industrial Production Methods: : Industrial production of casopitant mesylate follows a similar synthetic route but is optimized for large-scale production. This involves the use of high-yield reactions, efficient purification methods, and stringent quality control measures to ensure the consistency and purity of the final product .
Analyse Chemischer Reaktionen
Arten von Reaktionen: : Casopitant-Mesylat unterliegt verschiedenen chemischen Reaktionen, darunter:
Oxidation: Die Verbindung kann unter bestimmten Bedingungen oxidiert werden, um verschiedene oxidierte Derivate zu bilden.
Reduktion: Reduktionsreaktionen können verwendet werden, um die funktionellen Gruppen am Molekül zu modifizieren.
Substitution: Substitutionsreaktionen sind üblich, wobei verschiedene funktionelle Gruppen eingeführt oder ersetzt werden können.
Häufige Reagenzien und Bedingungen
Oxidation: Häufige Oxidationsmittel sind Kaliumpermanganat und Wasserstoffperoxid.
Reduktion: Reduktionsmittel wie Lithiumaluminiumhydrid und Natriumborhydrid werden verwendet.
Substitution: Verschiedene Halogenierungsmittel und Nukleophile werden in Substitutionsreaktionen eingesetzt.
Hauptprodukte: : Die aus diesen Reaktionen entstehenden Hauptprodukte hängen von den spezifischen Bedingungen und den verwendeten Reagenzien ab. Beispielsweise kann die Oxidation hydroxylierte Derivate ergeben, während Substitutionsreaktionen neue funktionelle Gruppen einführen können .
Vergleich Mit ähnlichen Verbindungen
Ähnliche Verbindungen
Aprepitant: Ein weiterer Neurokinin-1-Rezeptor-Antagonist, der zur Vorbeugung von Chemotherapie-induzierter Übelkeit und Erbrechen eingesetzt wird.
Fosaprepitant: Ein Prodrug von Aprepitant mit ähnlichen Anwendungsgebieten.
Rolapitant: Ein langwirksamer Neurokinin-1-Rezeptor-Antagonist.
Einzigartigkeit: : Casopitant-Mesylat ist einzigartig in seiner spezifischen Bindungsaffinität und seinem pharmakokinetischen Profil, was im Vergleich zu anderen Neurokinin-1-Rezeptor-Antagonisten Vorteile in Bezug auf Wirksamkeit und Wirkdauer bieten kann .
Eigenschaften
IUPAC Name |
sodium;(3S)-3-hydroxybutanoate | |
---|---|---|
Source | PubChem | |
URL | https://pubchem.ncbi.nlm.nih.gov | |
Description | Data deposited in or computed by PubChem | |
InChI |
InChI=1S/C4H8O3.Na/c1-3(5)2-4(6)7;/h3,5H,2H2,1H3,(H,6,7);/q;+1/p-1/t3-;/m0./s1 | |
Source | PubChem | |
URL | https://pubchem.ncbi.nlm.nih.gov | |
Description | Data deposited in or computed by PubChem | |
InChI Key |
NBPUSGBJDWCHKC-DFWYDOINSA-M | |
Source | PubChem | |
URL | https://pubchem.ncbi.nlm.nih.gov | |
Description | Data deposited in or computed by PubChem | |
Canonical SMILES |
CC(CC(=O)[O-])O.[Na+] | |
Source | PubChem | |
URL | https://pubchem.ncbi.nlm.nih.gov | |
Description | Data deposited in or computed by PubChem | |
Isomeric SMILES |
C[C@@H](CC(=O)[O-])O.[Na+] | |
Source | PubChem | |
URL | https://pubchem.ncbi.nlm.nih.gov | |
Description | Data deposited in or computed by PubChem | |
Molecular Formula |
C4H7NaO3 | |
Source | PubChem | |
URL | https://pubchem.ncbi.nlm.nih.gov | |
Description | Data deposited in or computed by PubChem | |
Molecular Weight |
126.09 g/mol | |
Source | PubChem | |
URL | https://pubchem.ncbi.nlm.nih.gov | |
Description | Data deposited in or computed by PubChem | |
Retrosynthesis Analysis
AI-Powered Synthesis Planning: Our tool employs the Template_relevance Pistachio, Template_relevance Bkms_metabolic, Template_relevance Pistachio_ringbreaker, Template_relevance Reaxys, Template_relevance Reaxys_biocatalysis model, leveraging a vast database of chemical reactions to predict feasible synthetic routes.
One-Step Synthesis Focus: Specifically designed for one-step synthesis, it provides concise and direct routes for your target compounds, streamlining the synthesis process.
Accurate Predictions: Utilizing the extensive PISTACHIO, BKMS_METABOLIC, PISTACHIO_RINGBREAKER, REAXYS, REAXYS_BIOCATALYSIS database, our tool offers high-accuracy predictions, reflecting the latest in chemical research and data.
Strategy Settings
Precursor scoring | Relevance Heuristic |
---|---|
Min. plausibility | 0.01 |
Model | Template_relevance |
Template Set | Pistachio/Bkms_metabolic/Pistachio_ringbreaker/Reaxys/Reaxys_biocatalysis |
Top-N result to add to graph | 6 |
Feasible Synthetic Routes
Haftungsausschluss und Informationen zu In-Vitro-Forschungsprodukten
Bitte beachten Sie, dass alle Artikel und Produktinformationen, die auf BenchChem präsentiert werden, ausschließlich zu Informationszwecken bestimmt sind. Die auf BenchChem zum Kauf angebotenen Produkte sind speziell für In-vitro-Studien konzipiert, die außerhalb lebender Organismen durchgeführt werden. In-vitro-Studien, abgeleitet von dem lateinischen Begriff "in Glas", beinhalten Experimente, die in kontrollierten Laborumgebungen unter Verwendung von Zellen oder Geweben durchgeführt werden. Es ist wichtig zu beachten, dass diese Produkte nicht als Arzneimittel oder Medikamente eingestuft sind und keine Zulassung der FDA für die Vorbeugung, Behandlung oder Heilung von medizinischen Zuständen, Beschwerden oder Krankheiten erhalten haben. Wir müssen betonen, dass jede Form der körperlichen Einführung dieser Produkte in Menschen oder Tiere gesetzlich strikt untersagt ist. Es ist unerlässlich, sich an diese Richtlinien zu halten, um die Einhaltung rechtlicher und ethischer Standards in Forschung und Experiment zu gewährleisten.