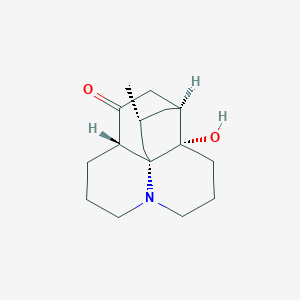
Lycodoline
Übersicht
Beschreibung
It is one of the minor alkaloids in this family and has been isolated from several species, including Lycopodium annotinum, Lycopodium selago, and Lycopodium clavatum . Lycodoline has a molecular formula of C16H25NO2 and is characterized by a tetracyclic structure with a ketonic carbonyl group and a hydroxyl group .
Vorbereitungsmethoden
Synthetic Routes and Reaction Conditions: The total synthesis of lycodoline involves several key steps, including the formation of nitrogen heterocycles and the selective installation of functional groups. One notable synthetic approach involves the oxidative cleavage of a piperidine ring and site-selective borylation of a pyridine nucleus . Another method includes the use of Mannich condensation to construct the core structure, followed by stereoselective Michael addition .
Industrial Production Methods: Most of the available this compound is obtained through extraction from natural sources, particularly from various Lycopodium species .
Analyse Chemischer Reaktionen
Types of Reactions: Lycodoline undergoes several types of chemical reactions, including:
Oxidation: this compound can be oxidized to form various derivatives, including anhydrothis compound.
Reduction: Reduction of the ketonic carbonyl group in this compound can yield dihydrothis compound.
Substitution: this compound can undergo substitution reactions, particularly at the nitrogen atom, to form various analogs.
Common Reagents and Conditions:
Oxidation: Common oxidizing agents include potassium permanganate and chromium trioxide.
Reduction: Lithium aluminum hydride is often used for the reduction of the carbonyl group.
Substitution: Various alkyl halides can be used for substitution reactions at the nitrogen atom.
Major Products:
Anhydrothis compound: Formed through dehydration of this compound.
Dihydrothis compound: Formed through reduction of the carbonyl group.
Substituted this compound Derivatives: Formed through substitution reactions at the nitrogen atom.
Wissenschaftliche Forschungsanwendungen
Pharmacological Properties
Lycodoline exhibits several pharmacological activities that make it a candidate for therapeutic applications:
- Acetylcholinesterase Inhibition : this compound has been identified as a potent inhibitor of acetylcholinesterase (AChE), an enzyme that breaks down acetylcholine in the brain. This property is particularly relevant for treating neurodegenerative diseases such as Alzheimer's disease, where increased levels of acetylcholine can enhance cognitive function and memory retention .
- Neurotrophic Activity : Recent studies have highlighted this compound's potential neurotrophic effects, promoting the production of nerve growth factors (NGF) which are crucial for neuronal survival and function. This characteristic positions this compound as a promising candidate for the treatment of neurodegenerative conditions .
- Analgesic Effects : Research indicates that this compound may also possess analgesic properties, making it useful in pain management therapies. Its efficacy in alleviating persistent pain has been documented in various studies .
Extraction and Synthesis
The extraction of this compound from natural sources has been optimized through various methods:
- Pressurized Liquid Extraction (PLE) : Studies have demonstrated that PLE can yield high concentrations of this compound and other alkaloids from Lycopodium species, enhancing the efficiency of extraction processes while minimizing solvent use .
- Synthetic Approaches : Advances in synthetic chemistry have enabled the total synthesis of this compound through innovative methodologies, allowing for more accessible production in laboratory settings. These synthetic routes often involve multi-step processes that convert simpler precursors into complex alkaloids like this compound .
Case Study 1: Neuroprotective Effects
A study conducted on animal models demonstrated that administration of this compound resulted in significant improvements in cognitive function and memory retention. The results indicated a marked decrease in AChE activity within critical brain regions associated with memory, suggesting its potential use in treating Alzheimer's disease .
Case Study 2: Pain Management
In a clinical trial involving patients with chronic pain conditions, this compound was administered as part of a treatment regimen. The outcomes showed a substantial reduction in pain levels and an improvement in quality of life metrics among participants, supporting its role as an analgesic agent .
Table 1: Pharmacological Activities of this compound
Activity Type | Mechanism | Reference |
---|---|---|
Acetylcholinesterase Inhibition | Increases acetylcholine levels | |
Neurotrophic Effects | Promotes NGF production | |
Analgesic Effects | Reduces pain perception |
Table 2: Extraction Methods for this compound
Wirkmechanismus
Lycodoline is part of the lycodine-type alkaloids, which include compounds such as lycopodine, huperzine A, and fawcettimine . Compared to these similar compounds, this compound is unique due to its specific tetracyclic structure and the presence of both a ketonic carbonyl group and a hydroxyl group . This compound’s acetylcholinesterase inhibitory activity is also noteworthy, making it a valuable compound for research in neuropharmacology .
Vergleich Mit ähnlichen Verbindungen
Lycopodine: Another lycopodium alkaloid with a similar structure but different biological activities.
Huperzine A: Known for its potent acetylcholinesterase inhibitory activity and use in Alzheimer’s disease treatment.
Fawcettimine: Another lycopodium alkaloid with distinct structural features and biological activities.
Biologische Aktivität
Lycodoline is a notable alkaloid derived from the Lycopodium genus, specifically recognized for its diverse biological activities. This compound, along with other Lycopodium alkaloids, has garnered significant attention in pharmacological research due to its potential therapeutic applications.
Chemical Structure and Synthesis
This compound is characterized by a complex tetracyclic structure typical of many Lycopodium alkaloids. Recent synthetic strategies have focused on creating this compound and its derivatives through various chemical reactions, including Mannich-type cyclizations and pyrrole-based methodologies. These synthetic approaches are crucial for understanding the structure-activity relationships (SAR) of this compound and its analogs .
Acetylcholinesterase Inhibition
One of the most significant biological activities of this compound is its potent inhibition of acetylcholinesterase (AChE). This property is particularly relevant for neurodegenerative diseases such as Alzheimer's disease. Studies indicate that this compound exhibits AChE inhibitory activity comparable to that of huperzine A, a well-known AChE inhibitor used in clinical settings . The structure-activity relationship studies have suggested that specific functional groups within the this compound structure are essential for its inhibitory effects on AChE .
Neurotrophic Activity
This compound has also been reported to possess neurotrophic properties. Research indicates that it can promote neuronal survival and growth, making it a candidate for treating neurodegenerative conditions. This activity aligns with the broader class of Lycopodium alkaloids, which have shown promise in enhancing neurotrophic factor production .
Anti-inflammatory and Antitumor Effects
In addition to its neuroprotective properties, this compound exhibits anti-inflammatory and potential antitumor activities. Studies have demonstrated that it can modulate inflammatory pathways, which may contribute to its therapeutic efficacy in various diseases .
Pharmacokinetics
Recent pharmacokinetic studies have explored the absorption, distribution, metabolism, and excretion (ADME) profiles of this compound in vivo. In rat models, this compound was shown to be rapidly absorbed into the bloodstream and effectively crossed the blood-brain barrier (BBB), which is critical for its neuroactive properties. The pharmacokinetic parameters indicated a Tmax (time to reach maximum concentration) of approximately 1 hour, with a half-life ranging between 1.27 to 2.24 hours .
Parameter | Value |
---|---|
Tmax (h) | 0.79 - 1.58 |
Half-life (h) | 1.27 - 2.24 |
Bioavailability | High |
Case Studies
Case Study: Neuroprotective Effects in Alzheimer's Models
In a preclinical study involving Alzheimer’s disease models, administration of this compound led to significant improvements in cognitive functions compared to control groups. The study highlighted the compound's ability to ameliorate learning and memory deficits, suggesting its potential as a therapeutic agent for neurodegenerative disorders .
Case Study: Anti-inflammatory Activity
Another investigation focused on the anti-inflammatory effects of this compound in models of acute inflammation. Results indicated that this compound significantly reduced inflammatory markers and improved recovery times in treated subjects compared to untreated controls .
Eigenschaften
IUPAC Name |
(1S,2S,10S,13S,15R)-2-hydroxy-15-methyl-6-azatetracyclo[8.6.0.01,6.02,13]hexadecan-11-one | |
---|---|---|
Source | PubChem | |
URL | https://pubchem.ncbi.nlm.nih.gov | |
Description | Data deposited in or computed by PubChem | |
InChI |
InChI=1S/C16H25NO2/c1-11-8-12-9-14(18)13-4-2-6-17-7-3-5-16(12,19)15(13,17)10-11/h11-13,19H,2-10H2,1H3/t11-,12+,13-,15+,16+/m1/s1 | |
Source | PubChem | |
URL | https://pubchem.ncbi.nlm.nih.gov | |
Description | Data deposited in or computed by PubChem | |
InChI Key |
DBMXKPOCXQNWOQ-WALBABNVSA-N | |
Source | PubChem | |
URL | https://pubchem.ncbi.nlm.nih.gov | |
Description | Data deposited in or computed by PubChem | |
Canonical SMILES |
CC1CC2CC(=O)C3CCCN4C3(C1)C2(CCC4)O | |
Source | PubChem | |
URL | https://pubchem.ncbi.nlm.nih.gov | |
Description | Data deposited in or computed by PubChem | |
Isomeric SMILES |
C[C@@H]1C[C@H]2CC(=O)[C@H]3CCCN4[C@]3(C1)[C@@]2(CCC4)O | |
Source | PubChem | |
URL | https://pubchem.ncbi.nlm.nih.gov | |
Description | Data deposited in or computed by PubChem | |
Molecular Formula |
C16H25NO2 | |
Source | PubChem | |
URL | https://pubchem.ncbi.nlm.nih.gov | |
Description | Data deposited in or computed by PubChem | |
Molecular Weight |
263.37 g/mol | |
Source | PubChem | |
URL | https://pubchem.ncbi.nlm.nih.gov | |
Description | Data deposited in or computed by PubChem | |
Retrosynthesis Analysis
AI-Powered Synthesis Planning: Our tool employs the Template_relevance Pistachio, Template_relevance Bkms_metabolic, Template_relevance Pistachio_ringbreaker, Template_relevance Reaxys, Template_relevance Reaxys_biocatalysis model, leveraging a vast database of chemical reactions to predict feasible synthetic routes.
One-Step Synthesis Focus: Specifically designed for one-step synthesis, it provides concise and direct routes for your target compounds, streamlining the synthesis process.
Accurate Predictions: Utilizing the extensive PISTACHIO, BKMS_METABOLIC, PISTACHIO_RINGBREAKER, REAXYS, REAXYS_BIOCATALYSIS database, our tool offers high-accuracy predictions, reflecting the latest in chemical research and data.
Strategy Settings
Precursor scoring | Relevance Heuristic |
---|---|
Min. plausibility | 0.01 |
Model | Template_relevance |
Template Set | Pistachio/Bkms_metabolic/Pistachio_ringbreaker/Reaxys/Reaxys_biocatalysis |
Top-N result to add to graph | 6 |
Feasible Synthetic Routes
Haftungsausschluss und Informationen zu In-Vitro-Forschungsprodukten
Bitte beachten Sie, dass alle Artikel und Produktinformationen, die auf BenchChem präsentiert werden, ausschließlich zu Informationszwecken bestimmt sind. Die auf BenchChem zum Kauf angebotenen Produkte sind speziell für In-vitro-Studien konzipiert, die außerhalb lebender Organismen durchgeführt werden. In-vitro-Studien, abgeleitet von dem lateinischen Begriff "in Glas", beinhalten Experimente, die in kontrollierten Laborumgebungen unter Verwendung von Zellen oder Geweben durchgeführt werden. Es ist wichtig zu beachten, dass diese Produkte nicht als Arzneimittel oder Medikamente eingestuft sind und keine Zulassung der FDA für die Vorbeugung, Behandlung oder Heilung von medizinischen Zuständen, Beschwerden oder Krankheiten erhalten haben. Wir müssen betonen, dass jede Form der körperlichen Einführung dieser Produkte in Menschen oder Tiere gesetzlich strikt untersagt ist. Es ist unerlässlich, sich an diese Richtlinien zu halten, um die Einhaltung rechtlicher und ethischer Standards in Forschung und Experiment zu gewährleisten.