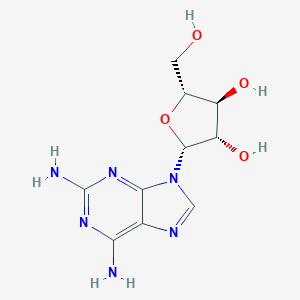
2,6-Diaminopurine arabinoside
Übersicht
Beschreibung
2,6-Diaminopurine arabinoside, also known as this compound, is a useful research compound. Its molecular formula is C10H14N6O4 and its molecular weight is 282.26 g/mol. The purity is usually 95%.
The exact mass of the compound (2R,3S,4S,5R)-2-(2,6-diaminopurin-9-yl)-5-(hydroxymethyl)oxolane-3,4-diol is unknown and the complexity rating of the compound is unknown. Its Medical Subject Headings (MeSH) category is Chemicals and Drugs Category - Carbohydrates - Glycosides - Nucleosides - Arabinonucleosides - Supplementary Records. The United Nations designated GHS hazard class pictogram is Acute Toxic, and the GHS signal word is DangerThe storage condition is unknown. Please store according to label instructions upon receipt of goods.
BenchChem offers high-quality this compound suitable for many research applications. Different packaging options are available to accommodate customers' requirements. Please inquire for more information about this compound including the price, delivery time, and more detailed information at info@benchchem.com.
Wirkmechanismus
Target of Action
The primary targets of AraDAP are currently unknown. Identifying the specific targets of a compound is a complex process that involves extensive biochemical and molecular biology studies. These studies often involve techniques such as affinity chromatography, mass spectrometry, and genetic screens .
Mode of Action
The interaction of a compound with its targets can lead to a variety of changes, including alteration of the target’s function, inhibition or activation of enzymatic activity, or modulation of signal transduction pathways .
Biochemical Pathways
These pathways can include those involved in cell growth and proliferation, apoptosis, signal transduction, and metabolic processes .
Pharmacokinetics
These properties are crucial for understanding the bioavailability of a compound, its distribution within the body, how it is metabolized, and how it is eliminated from the body .
Result of Action
The molecular and cellular effects of AraDAP’s action are not well documented. Understanding these effects requires detailed studies at the molecular, cellular, and organismal levels. These studies can provide insights into how the compound affects cellular processes, alters gene expression, and impacts overall cellular function .
Action Environment
Environmental factors can significantly influence the action, efficacy, and stability of a compound. Factors such as temperature, pH, and the presence of other molecules can affect a compound’s stability and its ability to interact with its targets. Additionally, the physiological environment within the body, including the presence of other metabolites and the specific characteristics of different tissues, can also impact a compound’s action .
Biologische Aktivität
2,6-Diaminopurine arabinoside (DAPD) is a nucleoside analog that has garnered attention for its potential therapeutic applications, particularly in the treatment of viral infections such as HIV and herpesviruses. This article provides a comprehensive overview of the biological activity of DAPD, including its mechanisms of action, efficacy against various pathogens, and relevant case studies.
DAPD is a purine analog that mimics the natural nucleosides in the body. Its structure allows it to interfere with nucleic acid synthesis, which is crucial for viral replication. The compound acts primarily as a reverse transcriptase inhibitor (NRTI), blocking the replication of retroviruses by preventing the conversion of viral RNA into DNA.
1. Anti-HIV Activity
Research has demonstrated that DAPD exhibits significant anti-HIV activity. A study highlighted that modified prodrugs of DAPD showed enhanced antiviral potency against HIV-1, particularly in drug-resistant strains. For instance, certain derivatives were found to be up to 17 times more potent than DAPD itself while maintaining low cytotoxicity levels in human lymphocytes .
Compound | Potency (fold increase) | Target Virus |
---|---|---|
DAPD | 1 | HIV-1 |
Modified Prodrug | 17 | HIV-1 |
This increased potency can be attributed to structural modifications at the C6 position of the purine ring, which enhances binding affinity to viral enzymes .
2. Anti-Herpesvirus Activity
DAPD also exhibits antiviral properties against herpesviruses. A comparative study indicated that 4'-thioarabinofuranosyl derivatives of DAPD showed potent activity against human cytomegalovirus (HCMV) and other herpesviruses, outperforming traditional antiviral agents like ganciclovir . The effective concentration (ED50) for these derivatives was significantly lower than that for ganciclovir, suggesting a promising alternative for treatment-resistant cases.
Compound | ED50 (µM) | Virus Type |
---|---|---|
Ganciclovir | Higher | HCMV |
4'-thio-FaraDAP | Lower | HCMV |
Case Studies and Clinical Implications
Several clinical studies have explored the therapeutic potential of DAPD in patients with chronic viral infections. One notable case involved patients with HIV strains resistant to multiple nucleoside analogs. Treatment with DAPD resulted in significant viral load reductions and improved immune function markers .
Additionally, studies on the pharmacokinetics of DAPD indicate that while its oral bioavailability is limited (approximately 30%), its effectiveness remains promising when administered intravenously or through optimized formulations .
Toxicity and Side Effects
Despite its efficacy, the use of DAPD is not without concerns regarding toxicity. Research indicates that while modified forms exhibit reduced toxicity compared to conventional therapies, careful monitoring is necessary due to potential side effects related to cellular metabolism interference .
Wissenschaftliche Forschungsanwendungen
Antiviral Activity
Herpes Simplex Virus (HSV) Inhibition
Ara-DAP has demonstrated significant antiviral activity against herpes simplex virus types 1 and 2 (HSV-1 and HSV-2). Research conducted by Wellcome Research Laboratories in the late 1960s revealed that Ara-DAP effectively inhibited viral replication. The compound was synthesized as an analogue of adenine, capitalizing on its structural similarities to enhance its antiviral efficacy against DNA viruses .
Mechanism of Action
The antiviral mechanism of Ara-DAP involves its incorporation into viral DNA during replication, leading to premature termination of the viral genome synthesis. This results in reduced viral load and alleviation of symptoms associated with herpes infections .
Antitumor Properties
Cytotoxicity Against Cancer Cells
Ara-DAP has been investigated for its cytotoxic effects on various cancer cell lines, including HeLa cells. Studies have shown that purine arabinosides exhibit notable antitumor activity, making them potential candidates for cancer therapy . The compound's ability to interfere with nucleic acid synthesis in rapidly dividing cells is a key factor contributing to its anticancer properties.
Combination Therapies
The use of Ara-DAP in combination with other chemotherapeutic agents is being explored to enhance therapeutic efficacy while minimizing side effects. Its role as a nucleoside analogue allows it to synergize with existing treatments, potentially improving outcomes in cancer patients .
Prebiotic Chemistry and Origins of Life Studies
Role in Nucleic Acid Synthesis
Research indicates that 2,6-diaminopurine could have played a pivotal role in the prebiotic synthesis of RNA and DNA. The compound enhances the repair of photodamaged nucleic acids, specifically cyclobutane pyrimidine dimers (CPDs), which are common lesions formed under UV light exposure . Its electron-donating properties facilitate efficient repair mechanisms, suggesting a possible pathway for the emergence of life on early Earth.
Implications for Astrobiology
The presence of purines like 2,6-diaminopurine in meteoritic samples supports theories regarding the extraterrestrial origins of life's building blocks. This highlights the compound's significance not only in biological contexts but also in understanding the chemical pathways that may have led to life beyond Earth .
Synthesis and Modification
Chemical Synthesis Techniques
Recent advancements in synthetic methodologies have allowed for the efficient production of Ara-DAP and its derivatives. Techniques such as postsynthetic modification enable the incorporation of 2,6-diaminopurine into oligonucleotides, expanding its potential applications in genetic research and therapeutic development .
Fluorinated Derivatives
The development of fluorinated analogues of Ara-DAP has also been explored, aiming to enhance its pharmacological properties while providing insights into structure-activity relationships within nucleoside analogues .
Q & A
Basic Research Questions
Q. What are the established synthetic routes for 2,6-diaminopurine arabinoside, and what are the critical intermediates?
The synthesis of this compound typically involves converting precursors like 2-amino-6-chloropurine riboside. Key steps include ammonolysis under controlled temperature and pressure to replace chlorine with an amino group, followed by deprotection using methylamine or ammonia. This method avoids side reactions like 6-O-methylation, achieving yields up to 65% . Alternative routes use 2-amino-6-azidopurine intermediates, which are reduced to form the diaminopurine moiety. These approaches require benzoyl or acetyl protecting groups for amino functionalities, with subsequent deprotection under mild conditions (e.g., 40% methylamine at 60–65°C) .
Q. How does this compound exhibit antiviral activity against DNA viruses like herpes simplex?
The compound acts as a nucleoside analog, inhibiting viral DNA polymerase. Its arabinose sugar configuration enhances selectivity for viral enzymes over host polymerases. In vitro studies show activity against herpes simplex virus (HSV-1/2) and vaccinia virus, with reduced cytotoxicity in mammalian cells compared to adenine arabinoside (ara-A). Mechanistic studies involve measuring IC50 values in viral plaque reduction assays and comparing thymidine kinase phosphorylation rates in infected vs. uninfected cells .
Q. What analytical methods are used to characterize this compound purity and stability?
High-performance liquid chromatography (HPLC) with UV detection (λ = 260–280 nm) is standard for purity assessment. Stability studies under varying pH, temperature, and humidity conditions are conducted using accelerated degradation protocols (e.g., 40°C/75% relative humidity for 3 months). Mass spectrometry (MS) and nuclear magnetic resonance (NMR) confirm structural integrity, particularly monitoring deamination or glycosidic bond hydrolysis .
Advanced Research Questions
Q. How can this compound address UGA nonsense mutations in gene editing?
The compound acts as a translational readthrough agent by pairing with inosine (formed via deamination of adenine) at the wobble position of tRNA. This suppresses premature termination caused by UGA codons. Experimental designs involve transfecting mammalian cells with reporter plasmids containing UGA mutations and quantifying full-length protein restoration via Western blot or luciferase assays. Dose-response curves (0.1–100 µM) and cytotoxicity assays (e.g., MTT) optimize therapeutic windows .
Q. What strategies mitigate off-target effects of this compound in antiviral therapy?
Host toxicity arises from incorporation into mitochondrial DNA. To minimize this, researchers use prodrug approaches (e.g., phosphonate derivatives) that require viral kinase activation for intracellular conversion. Comparative transcriptomics (RNA-seq) of treated vs. untreated cells identifies pathways affected by off-target DNA damage. Additionally, molecular dynamics simulations predict binding affinities to human DNA polymerases γ vs. viral polymerases .
Q. How does substituting adenine with 2,6-diaminopurine enhance oligonucleotide duplex stability?
The additional amino group forms a third hydrogen bond with thymine/uracil, increasing melting temperatures (Tm) by 0.7–2.7 kcal/mol. Thermodynamic parameters (ΔG°, ΔH°) are quantified via UV-monitored melting curves. For example, in 2'-O-methyl RNA/RNA duplexes, internal substitutions improve stability by ~1.2 kcal/mol, while terminal substitutions show smaller effects. This property is exploited in isoenergetic probe design for RNA structure mapping and CRISPR guide RNA optimization .
Q. How do researchers resolve discrepancies in reported cytotoxicity profiles of this compound?
Contradictory data may arise from cell line-specific metabolic activation (e.g., adenosine deaminase activity). Systematic comparisons use isogenic cell pairs differing in deaminase expression (e.g., ADA⁺ vs. ADA⁻). Metabolomic profiling (LC-MS) tracks intracellular conversion to toxic deaminated products. Cross-study harmonization includes standardizing culture conditions (e.g., serum-free media to avoid exogenous enzyme interference) .
Q. Methodological Tables
Table 1. Key thermodynamic parameters for 2,6-diaminopurine-containing oligonucleotides.
Modification | ΔTm (°C) | ΔΔG° (kcal/mol) | Reference |
---|---|---|---|
Internal DM (2'-OMe) | +4.2 | -1.1 | |
Terminal DM | +1.8 | -0.4 | |
Internal DL (LNA) | +8.5 | -2.6 |
Table 2. Antiviral activity of this compound.
Virus | EC50 (µM) | Selectivity Index (CC50/EC50) | Assay Type | Reference |
---|---|---|---|---|
HSV-1 | 0.3 | 300 | Plaque reduction | |
Vaccinia | 1.2 | 75 | Cytopathic effect |
Eigenschaften
IUPAC Name |
(2R,3S,4S,5R)-2-(2,6-diaminopurin-9-yl)-5-(hydroxymethyl)oxolane-3,4-diol | |
---|---|---|
Source | PubChem | |
URL | https://pubchem.ncbi.nlm.nih.gov | |
Description | Data deposited in or computed by PubChem | |
InChI |
InChI=1S/C10H14N6O4/c11-7-4-8(15-10(12)14-7)16(2-13-4)9-6(19)5(18)3(1-17)20-9/h2-3,5-6,9,17-19H,1H2,(H4,11,12,14,15)/t3-,5-,6+,9-/m1/s1 | |
Source | PubChem | |
URL | https://pubchem.ncbi.nlm.nih.gov | |
Description | Data deposited in or computed by PubChem | |
InChI Key |
ZDTFMPXQUSBYRL-FJFJXFQQSA-N | |
Source | PubChem | |
URL | https://pubchem.ncbi.nlm.nih.gov | |
Description | Data deposited in or computed by PubChem | |
Canonical SMILES |
C1=NC2=C(N=C(N=C2N1C3C(C(C(O3)CO)O)O)N)N | |
Source | PubChem | |
URL | https://pubchem.ncbi.nlm.nih.gov | |
Description | Data deposited in or computed by PubChem | |
Isomeric SMILES |
C1=NC2=C(N=C(N=C2N1[C@H]3[C@H]([C@@H]([C@H](O3)CO)O)O)N)N | |
Source | PubChem | |
URL | https://pubchem.ncbi.nlm.nih.gov | |
Description | Data deposited in or computed by PubChem | |
Molecular Formula |
C10H14N6O4 | |
Source | PubChem | |
URL | https://pubchem.ncbi.nlm.nih.gov | |
Description | Data deposited in or computed by PubChem | |
DSSTOX Substance ID |
DTXSID70941537 | |
Record name | 2-Imino-9-pentofuranosyl-2,9-dihydro-1H-purin-6-amine | |
Source | EPA DSSTox | |
URL | https://comptox.epa.gov/dashboard/DTXSID70941537 | |
Description | DSSTox provides a high quality public chemistry resource for supporting improved predictive toxicology. | |
Molecular Weight |
282.26 g/mol | |
Source | PubChem | |
URL | https://pubchem.ncbi.nlm.nih.gov | |
Description | Data deposited in or computed by PubChem | |
CAS No. |
19768-89-9, 34079-68-0 | |
Record name | 9H-Purine-2,6-diamine, 9-b-D-xylofuranosyl- | |
Source | ChemIDplus | |
URL | https://pubchem.ncbi.nlm.nih.gov/substance/?source=chemidplus&sourceid=0019768899 | |
Description | ChemIDplus is a free, web search system that provides access to the structure and nomenclature authority files used for the identification of chemical substances cited in National Library of Medicine (NLM) databases, including the TOXNET system. | |
Record name | sra-DAP | |
Source | ChemIDplus | |
URL | https://pubchem.ncbi.nlm.nih.gov/substance/?source=chemidplus&sourceid=0034079680 | |
Description | ChemIDplus is a free, web search system that provides access to the structure and nomenclature authority files used for the identification of chemical substances cited in National Library of Medicine (NLM) databases, including the TOXNET system. | |
Record name | 2-Imino-9-pentofuranosyl-2,9-dihydro-1H-purin-6-amine | |
Source | EPA DSSTox | |
URL | https://comptox.epa.gov/dashboard/DTXSID70941537 | |
Description | DSSTox provides a high quality public chemistry resource for supporting improved predictive toxicology. | |
Record name | 34079-68-0 | |
Source | European Chemicals Agency (ECHA) | |
URL | https://echa.europa.eu/information-on-chemicals | |
Description | The European Chemicals Agency (ECHA) is an agency of the European Union which is the driving force among regulatory authorities in implementing the EU's groundbreaking chemicals legislation for the benefit of human health and the environment as well as for innovation and competitiveness. | |
Explanation | Use of the information, documents and data from the ECHA website is subject to the terms and conditions of this Legal Notice, and subject to other binding limitations provided for under applicable law, the information, documents and data made available on the ECHA website may be reproduced, distributed and/or used, totally or in part, for non-commercial purposes provided that ECHA is acknowledged as the source: "Source: European Chemicals Agency, http://echa.europa.eu/". Such acknowledgement must be included in each copy of the material. ECHA permits and encourages organisations and individuals to create links to the ECHA website under the following cumulative conditions: Links can only be made to webpages that provide a link to the Legal Notice page. | |
Synthesis routes and methods I
Procedure details
Synthesis routes and methods II
Procedure details
Retrosynthesis Analysis
AI-Powered Synthesis Planning: Our tool employs the Template_relevance Pistachio, Template_relevance Bkms_metabolic, Template_relevance Pistachio_ringbreaker, Template_relevance Reaxys, Template_relevance Reaxys_biocatalysis model, leveraging a vast database of chemical reactions to predict feasible synthetic routes.
One-Step Synthesis Focus: Specifically designed for one-step synthesis, it provides concise and direct routes for your target compounds, streamlining the synthesis process.
Accurate Predictions: Utilizing the extensive PISTACHIO, BKMS_METABOLIC, PISTACHIO_RINGBREAKER, REAXYS, REAXYS_BIOCATALYSIS database, our tool offers high-accuracy predictions, reflecting the latest in chemical research and data.
Strategy Settings
Precursor scoring | Relevance Heuristic |
---|---|
Min. plausibility | 0.01 |
Model | Template_relevance |
Template Set | Pistachio/Bkms_metabolic/Pistachio_ringbreaker/Reaxys/Reaxys_biocatalysis |
Top-N result to add to graph | 6 |
Feasible Synthetic Routes
Haftungsausschluss und Informationen zu In-Vitro-Forschungsprodukten
Bitte beachten Sie, dass alle Artikel und Produktinformationen, die auf BenchChem präsentiert werden, ausschließlich zu Informationszwecken bestimmt sind. Die auf BenchChem zum Kauf angebotenen Produkte sind speziell für In-vitro-Studien konzipiert, die außerhalb lebender Organismen durchgeführt werden. In-vitro-Studien, abgeleitet von dem lateinischen Begriff "in Glas", beinhalten Experimente, die in kontrollierten Laborumgebungen unter Verwendung von Zellen oder Geweben durchgeführt werden. Es ist wichtig zu beachten, dass diese Produkte nicht als Arzneimittel oder Medikamente eingestuft sind und keine Zulassung der FDA für die Vorbeugung, Behandlung oder Heilung von medizinischen Zuständen, Beschwerden oder Krankheiten erhalten haben. Wir müssen betonen, dass jede Form der körperlichen Einführung dieser Produkte in Menschen oder Tiere gesetzlich strikt untersagt ist. Es ist unerlässlich, sich an diese Richtlinien zu halten, um die Einhaltung rechtlicher und ethischer Standards in Forschung und Experiment zu gewährleisten.