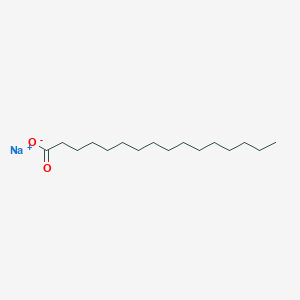
Sodium palmitate
Ăbersicht
Beschreibung
Sodium palmitate (CââHââOâNa) is the sodium salt of palmitic acid, a saturated long-chain fatty acid (16:0). It is widely utilized in biochemical research, particularly in studies of lipid metabolism, cellular steatosis induction, and surfactant applications. This compound is synthesized by dissolving palmitic acid in sodium hydroxide (NaOH) at elevated temperatures (70°C) and conjugating it with fatty acid-free bovine serum albumin (BSA) to enhance solubility in aqueous media . Its hydrophobic alkyl chain and hydrophilic carboxylate group confer amphiphilic properties, enabling interactions with biological membranes and proteins .
Vorbereitungsmethoden
Saponification of Palm Oil
Reaction Mechanism and Stoichiometry
Saponification is the traditional method for producing sodium palmitate, involving the alkaline hydrolysis of palm oil triglycerides. The reaction proceeds as follows:
{16}\text{H}{31}\text{O}2)3\text{C}3\text{H}5 + 3\ \text{NaOH} \rightarrow \text{C}3\text{H}5(\text{OH})3 + 3\ \text{C}{16}\text{H}{31}\text{O}2\text{Na}
Here, glyceryl tripalmitate (the primary triglyceride in palm oil) reacts with sodium hydroxide to yield glycerol and this compound . This exothermic reaction requires controlled heating to ensure complete conversion.
Industrial Protocol
Commercial production typically involves the following steps :
-
Mixing : Palm oil is heated to 60â80°C and combined with a 20â30% aqueous sodium hydroxide solution.
-
Saponification : The mixture is stirred vigorously for 4â6 hours to ensure complete hydrolysis.
-
Salting Out : Sodium chloride is added to precipitate this compound, separating it from glycerol-rich aqueous phases.
-
Purification : The precipitate is washed with water to remove residual alkali and dried into granules or paste.
Table 1: Industrial Saponification Parameters
Parameter | Value/Range | Source |
---|---|---|
Temperature | 60â80°C | |
NaOH Concentration | 20â30% (w/v) | |
Reaction Time | 4â6 hours | |
Final Product Form | White granules/paste |
Neutralization of Palmitic Acid
Direct Acid-Base Reaction
This compound can also be synthesized by neutralizing palmitic acid with sodium hydroxide:
{16}\text{H}{32}\text{O}2 + \text{NaOH} \rightarrow \text{C}{16}\text{H}{31}\text{O}2\text{Na} + \text{H}_2\text{O}
This method is preferred in laboratory settings for its simplicity and control over stoichiometry .
Laboratory-Scale Protocol
-
Dissolution : Palmitic acid is dissolved in ethanol or hot water (70â80°C).
-
Neutralization : A stoichiometric amount of sodium hydroxide solution is added dropwise under stirring.
-
Crystallization : The mixture is cooled to room temperature, inducing this compound precipitation.
-
Filtration and Drying : The precipitate is vacuum-filtered, washed with cold ethanol, and dried at 50°C .
Table 2: Neutralization Reaction Conditions
Parameter | Value/Range | Source |
---|---|---|
Solvent | Ethanol/Water | |
Reaction Temperature | 70â80°C | |
Drying Temperature | 50°C | |
Purity | >98% |
Comparative Analysis of Methods
Yield and Purity
-
Saponification : Yields ~85â90% this compound but may contain residual glycerol and alkali .
-
Neutralization : Achieves >98% purity (as confirmed by HPLC in commercial batches) but requires high-grade palmitic acid .
Industrial vs. Laboratory Applications
-
Industrial : Saponification is cost-effective for bulk production using palm oil, a renewable resource .
-
Laboratory : Neutralization allows precise control over stoichiometry, critical for research applications like lipid toxicity studies .
Quality Control and Characterization
Analytical Methods
-
FT-IR Spectroscopy : Confirms the presence of carboxylate peaks at 1,550â1,600 cmâ»Âč .
-
Titration : Measures residual alkali content (target: <0.1% w/w) .
-
Thermogravimetric Analysis (TGA) : Determines thermal stability, with decomposition onset at ~250°C .
Applications in Research
Lipid Toxicity Models
In cellular studies, this compound is dissolved in DMEM containing 1% bovine serum albumin (BSA) to create 25â150 ”M solutions. For example, 100 ”M this compound incubated with LO2 liver cells for 48 hours induces lipotoxicity, mimicking non-alcoholic fatty liver disease .
Table 3: Research Preparation Protocol
Parameter | Value/Range | Source |
---|---|---|
Solvent | DMEM + 1% BSA | |
Concentration | 25â150 ”M | |
Incubation Time | 12â48 hours |
Challenges and Optimization
Impurity Management
-
Saponification : Excess NaOH is neutralized with weak acids (e.g., citric acid) post-reaction .
-
Neutralization : Ethanol washing removes unreacted palmitic acid .
Solubility Considerations
This compound has limited aqueous solubility (8.33 mg/mL in DMSO at 60°C) , necessitating surfactants or organic solvents for biological studies.
Wissenschaftliche Forschungsanwendungen
Biological Research Applications
Cell Metabolism Studies
Sodium palmitate is widely used in cell culture to study lipid metabolism and lipotoxicity. It enhances lipogenesis and lipid droplet formation in various cell lines, making it an essential compound for establishing models of lipid droplet accumulation. For instance, concentrations of this compound (0.1, 0.25, or 0.5 mM) have been shown to increase mRNA levels of Notch receptors in liver cell lines such as LX2 and Huh7, indicating its role in cellular signaling pathways related to metabolism .
Lipotoxicity Models
this compound serves as a model for studying lipotoxicity. In experiments with LO2 liver cells, treatment with 100 ”mol/L this compound for 48 hours significantly inhibited cell viability, providing insights into the mechanisms of cellular stress induced by excess fatty acids .
Mitochondrial Function Studies
Research has demonstrated that prolonged exposure to this compound can lead to mitochondrial uncoupling in pancreatic islets, affecting insulin secretion and ATP levels. This highlights its relevance in diabetes research and the understanding of metabolic disorders .
Pharmaceutical Applications
Drug Formulation
this compound is utilized in the formulation of various pharmaceutical products due to its emulsifying properties. It aids in the solubilization of active ingredients and enhances drug delivery systems. The compound's ability to form micelles makes it suitable for use in oral and topical drug formulations.
Antimicrobial Activity
Recent studies have shown that this compound exhibits antimicrobial properties against both Gram-positive and Gram-negative bacteria. It has been demonstrated to inhibit bacterial growth effectively, which could lead to its application in developing antimicrobial agents for medical use .
Cosmetic and Personal Care Products
Soap Production
this compound is a key ingredient in the saponification process used to create soaps. It reacts with alkaline substances (like lye) to produce soap while contributing to the foaming and cleansing properties of the final product .
Skin Care Formulations
In cosmetic formulations, this compound is valued for its emollient properties, helping to improve skin texture and moisture retention. Its incorporation into lotions and creams enhances product stability and skin feel.
Nutritional Applications
Nutritional Supplementation
In nutritional research, this compound is sometimes used as a supplement to study its effects on lipid metabolism and energy balance in various animal models . Its role in enhancing lipogenesis makes it relevant for understanding dietary impacts on health.
Data Table: Summary of this compound Applications
Case Studies
Case Study 1: Lipotoxicity Induction
A study investigated the effects of this compound on liver cells (LO2), revealing that exposure led to significant reductions in cell viability after 48 hours at a concentration of 100 ”mol/L. This model was pivotal for understanding how excess fatty acids contribute to liver dysfunction.
Case Study 2: Insulin Secretion Impairment
Research involving isolated rat pancreatic islets demonstrated that prolonged exposure to this compound resulted in decreased insulin release due to mitochondrial dysfunction. The study provided insights into how fatty acids can disrupt normal pancreatic function, relevant for diabetes research .
Wirkmechanismus
Sodium palmitate exerts its effects through several molecular pathways:
Induction of Autophagy: this compound can induce autophagy in cells by activating the PI3K/AKT/mTOR signaling pathway.
Endoplasmic Reticulum Stress: It can induce endoplasmic reticulum stress, leading to the activation of the unfolded protein response.
Vergleich Mit Àhnlichen Verbindungen
Sodium Palmitate vs. Potassium Palmitate
Key Differences in Extraction Efficiency for Ce(IV) Ions:
Parameter | This compound | Potassium Palmitate |
---|---|---|
Extraction onset pH | 1.0 | 2.0 |
Peak extraction pH | 4.0 | 3.5 |
Extraction at pH 6.0 | 2% | 16% |
Rate of decline post-peak | Rapid | Gradual |
This compound initiates Ce(IV) ion extraction at lower pH but exhibits lower efficiency at neutral pH compared to potassium palmitate, which retains higher extraction capacity at pH 6.0. This divergence arises from differences in counterion (Naâș vs. Kâș) solubility and pH-dependent micelle stability .
This compound vs. Sodium Oleate
Hemolytic Activity and Structural Impact:
Parameter | This compound (C16:0) | Sodium Oleate (C18:1) |
---|---|---|
Minimum hemolytic dose | >0.4 mg | 0.03 mg |
Structural feature | Saturated (no double bond) | Unsaturated (one cis double bond) |
Membrane disruption | Lower | Higher |
The unsaturated structure of sodium oleate enhances its hemolytic potency by increasing membrane fluidity and integration, whereas this compoundâs saturated chain reduces cytotoxicity .
This compound vs. Short-Chain Fatty Acid Salts
Biological Transport Specificity:
Parameter | This compound (C16) | Sodium Laurate (C12) |
---|---|---|
Uptake inhibition | Competitive (by C12âC18 fatty acids) | Stimulatory effect on palmitate uptake |
pH optimum for uptake | 6.8 | Not applicable |
This compound transport in Hymenolepis diminuta involves a pH-sensitive, saturable system inhibited competitively by laurate (C12) and longer-chain acids.
Biologische AktivitÀt
Sodium palmitate, the sodium salt of palmitic acid, is a long-chain saturated fatty acid with significant biological activity. This compound is widely studied for its effects on cellular metabolism, insulin sensitivity, and potential therapeutic applications in various diseases. This article delves into the biological activities of this compound, supported by research findings, data tables, and case studies.
This compound is formed through the neutralization of palmitic acid with sodium hydroxide. Its molecular formula is C16H31NaO2, and it appears as a white solid at room temperature. The melting point of this compound is approximately 300 °C, indicating its stability under physiological conditions.
Property | Value |
---|---|
Molecular Formula | C16H31NaO2 |
Molecular Weight | 288.43 g/mol |
Melting Point | ~300 °C |
Solubility | Soluble in water |
1. Effect on Insulin Secretion
This compound has been shown to influence insulin secretion from pancreatic beta cells. Prolonged exposure to this compound can lead to a reduction in glucose-sensitive insulin release and decreased insulin content in isolated rat islets. This effect is associated with increased mitochondrial respiration and reactive oxygen species (ROS) production, which may contribute to lipotoxicity in beta cells .
2. Impact on Cellular Metabolism
This compound has been implicated in the modulation of fatty acid metabolism. In studies involving INS-1 beta cells, this compound exposure resulted in increased expression of endoplasmic reticulum (ER) stress markers and mitochondrial dysfunction. This suggests that high levels of saturated fatty acids can lead to cellular stress and apoptosis .
3. Stimulation of Epithelial Sodium Channels (ENaC)
Research indicates that this compound can stimulate ENaC activity by elevating intracellular calcium levels. This effect is mediated through oxidative stress pathways, highlighting the dual role of this compound as both a signaling molecule and a potential stressor within epithelial cells .
Case Study 1: Lipotoxicity in Diabetes
A study investigated the effects of this compound on INS-1 beta cells, showing that treatment with this compound led to significant cell death due to lipotoxicity. The addition of protective agents like sodium fluorocitrate demonstrated a reduction in cell death and improved viability, indicating potential therapeutic strategies for managing diabetes-induced beta cell dysfunction .
Case Study 2: Effects on Adipocytes
In adipocyte models, this compound was found to enhance lipid accumulation and promote adipogenesis. This suggests that while this compound can induce adverse effects in pancreatic cells, it may also play a role in normal adipocyte function under controlled conditions .
Research Findings
Recent studies have highlighted the complex role of this compound in metabolic processes:
- Mitochondrial Function : Long-term exposure to this compound results in mitochondrial uncoupling and altered ATP production, which may impair insulin secretion mechanisms .
- Oxidative Stress : this compound induces oxidative stress through ROS generation, contributing to cellular damage and apoptosis in various cell types .
- Antimicrobial Properties : Interestingly, this compound has demonstrated antimicrobial activity against both Gram-positive and Gram-negative bacteria, suggesting potential applications in medical devices and antimicrobial systems .
Q & A
Basic Research Questions
Q. How can sodium palmitate be synthesized and characterized in laboratory settings?
- Methodological Answer : this compound is synthesized via saponification of palmitic acid with sodium hydroxide (1:1 molar ratio) under controlled temperature (70â80°C) and stirring. Post-synthesis, characterization should include:
- FTIR to confirm carboxylate anion formation (asymmetric stretching at ~1550 cmâ»Âč).
- ÂčH/ÂčÂłC NMR to verify alkyl chain integrity and absence of ester peaks.
- HPLC with evaporative light scattering detection (ELSD) to assess purity (>98.5%) .
Q. Which analytical techniques are optimal for assessing this compound purity and stability?
- Methodological Answer :
- Thermal Gravimetric Analysis (TGA) to evaluate thermal stability (decomposition onset ~250°C).
- Differential Scanning Calorimetry (DSC) to detect polymorphic transitions.
- Mass Spectrometry (MS) for molecular weight confirmation (MW: 278.4 g/mol).
- pH-dependent solubility studies in aqueous buffers (e.g., PBS) to assess colloidal stability .
Q. What in vitro protocols are used to study this compoundâs effects on lipid metabolism?
- Methodological Answer :
- Cell Culture : Use hepatocytes (e.g., HepG2) or adipocytes, treating with this compound conjugated to fatty-acid-free BSA (molar ratio 6:1).
- Lipid Accumulation Assays : Quantify intracellular triglycerides via Oil Red O staining or enzymatic kits.
- Gene Expression : RT-qPCR for lipid metabolism markers (e.g., FASN, CPT1A) .
Advanced Research Questions
Q. What experimental approaches elucidate this compoundâs role in endoplasmic reticulum (ER) stress pathways?
- Methodological Answer :
- Transcriptomics : Perform RNA-seq on treated cells to identify upregulated ER stress markers (e.g., CHOP, XBP1).
- Functional Assays : Use siRNA knockdown of IRE1α or ATF6 to validate pathway specificity.
- Live-Cell Imaging : Monitor calcium flux using Fura-2 AM dye .
Q. How can researchers resolve discrepancies in this compoundâs pro-apoptotic effects across studies?
- Methodological Answer :
- Standardized Conditions : Control variables such as:
- This compound concentration (typical range: 100â500 ÎŒM).
- Serum-free vs. serum-containing media.
- Exposure duration (acute vs. chronic).
- Meta-Analysis : Systematically review studies using PRISMA guidelines to identify confounding factors (e.g., BSA batch variability) .
Q. What strategies optimize this compound-protein binding assays given its amphiphilic nature?
- Methodological Answer :
- Surface Plasmon Resonance (SPR) : Use immobilized proteins (e.g., albumin) with this compound solubilized in micelles (critical micelle concentration: ~0.1 mM).
- Isothermal Titration Calorimetry (ITC) : Account for heat changes from micelle disruption during titration.
- Molecular Dynamics Simulations : Model interactions with lipid bilayers or hydrophobic protein pockets .
Q. How can multi-omics integration improve understanding of this compoundâs systemic metabolic effects?
- Methodological Answer :
- Multi-Omics Workflow :
Transcriptomics : Identify dysregulated pathways (e.g., ÎČ-oxidation).
Metabolomics (LC-MS) : Quantify acyl-carnitines and ketone bodies.
Lipidomics : Profile membrane phospholipid remodeling.
Eigenschaften
Key on ui mechanism of action |
Vascular dysfunction is a major complication of metabolic disorders such as diabetes and obesity. The current studies were undertaken to determine whether inflammatory responses are activated in the vasculature of mice with diet-induced obesity, and if so, whether Toll-Like Receptor-4 (TLR4), a key mediator of innate immunity, contributes to these responses. Mice lacking TLR4 (TLR4(-/-)) and wild-type (WT) controls were fed either a low fat (LF) control diet or a diet high in saturated fat (HF) for 8 weeks. In response to HF feeding, both genotypes displayed similar increases of body weight, body fat content, and serum insulin and free fatty acid (FFA) levels compared with mice on a LF diet. In lysates of thoracic aorta from WT mice maintained on a HF diet, markers of vascular inflammation both upstream (IKK-beta activity) and downstream of the transcriptional regulator, NF-kappa-B (ICAM protein and IL-6 mRNA expression), were increased and this effect was associated with cellular insulin resistance and impaired insulin stimulation of endothelial nitric oxide synthase (eNOS). In contrast, vascular inflammation and impaired insulin responsiveness were not evident in aortic samples taken from TLR4(-/-) mice fed the same HF diet, despite comparable increases of body fat mass. Incubation of either aortic explants from WT mice or cultured human microvascular endothelial cells with the saturated FFA, palmitate (100 mol/L), similarly activated IKK-beta, inhibited insulin signal transduction and blocked insulin-stimulated NO production. Each of these effects was subsequently shown to be dependent on both TLR4 and NF-kappa-B activation. These findings identify the TLR4 signaling pathway as a key mediator of the deleterious effects of palmitate on endothelial NO signaling, and are the first to document a key role for TLR4 in the mechanism whereby diet-induced obesity induces vascular inflammation and insulin resistance. /Palmitate/ Insulin stimulates its own secretion and synthesis by pancreatic beta-cells. Although the exact molecular mechanism involved is unknown, changes in beta-cell insulin signalling have been recognized as a potential link between insulin resistance and its impaired release, as observed in non-insulin-dependent diabetes. However, insulin resistance is also associated with elevated plasma levels of free fatty acids (FFA) that are well known modulators of insulin secretion by pancreatic islets. This information led us to investigate the effect of FFA on insulin receptor signalling in pancreatic islets. Exposure of pancreatic islets to palmitate caused up-regulation of several insulin-induced activities including tyrosine phosphorylation of insulin receptor and pp185. This is the first evidence that short exposure of these cells to 100 microM palmitate activates the early steps of insulin receptor signalling. 2-Bromopalmitate, a carnitine palmitoyl-CoA transferase-1 inhibitor, did not affect the effect of the fatty acid. Cerulenin, an acylation inhibitor, abolished the palmitate effect on protein levels and phosphorylation of insulin receptor. This result supports the proposition that protein acylation may be an important mechanism by which palmitate exerts its modulating effect on the intracellular insulin signalling pathway in rat pancreatic islets. Accumulation of long-chain fatty acids in the heart has been proposed to play a role in the development of heart failure and diabetic cardiomyopathy. Several animal models with increased cardiomyocyte lipid accumulation suggest a link between the accumulation of lipid, cardiomyocyte cell death and the development of cardiomyopathy. In this review, we discuss the mechanism through which fatty acid accumulation may contribute to the development or progression of heart failure by initiation of apoptotic cell death. Long-chain saturated fatty acids induce apoptosis through a mechanism involving the generation of reactive intermediates. Reactive intermediate production occurs in concert with de novo ceramide synthesis, but ceramide production is not required for cell death. Cardiomyocyte dysfunction and death from reactive intermediates generated by long-chain saturated fatty acids may contribute to the pathogenesis of human heart disease. /Long-chain Fatty Acids/ |
---|---|
CAS-Nummer |
408-35-5 |
Molekularformel |
C16H32NaO2 |
Molekulargewicht |
279.41 g/mol |
IUPAC-Name |
sodium;hexadecanoate |
InChI |
InChI=1S/C16H32O2.Na/c1-2-3-4-5-6-7-8-9-10-11-12-13-14-15-16(17)18;/h2-15H2,1H3,(H,17,18); |
InChI-SchlĂŒssel |
ZUWJMSFTDBLXRA-UHFFFAOYSA-N |
SMILES |
CCCCCCCCCCCCCCCC(=O)[O-].[Na+] |
Isomerische SMILES |
CCCCCCCCCCCCCCCC(=O)[O-].[Na+] |
Kanonische SMILES |
CCCCCCCCCCCCCCCC(=O)O.[Na] |
Color/Form |
WHITE CRYSTALS White to yellow powde |
melting_point |
286.5 °C 270 °C |
Key on ui other cas no. |
408-35-5 |
Physikalische Beschreibung |
Liquid |
Piktogramme |
Irritant |
Synonyme |
Acid, Hexadecanoic Acid, Palmitic Calcium Palmitate Hexadecanoic Acid Palmitate, Calcium Palmitate, Sodium Palmitic Acid Sodium Palmitate |
Herkunft des Produkts |
United States |
Retrosynthesis Analysis
AI-Powered Synthesis Planning: Our tool employs the Template_relevance Pistachio, Template_relevance Bkms_metabolic, Template_relevance Pistachio_ringbreaker, Template_relevance Reaxys, Template_relevance Reaxys_biocatalysis model, leveraging a vast database of chemical reactions to predict feasible synthetic routes.
One-Step Synthesis Focus: Specifically designed for one-step synthesis, it provides concise and direct routes for your target compounds, streamlining the synthesis process.
Accurate Predictions: Utilizing the extensive PISTACHIO, BKMS_METABOLIC, PISTACHIO_RINGBREAKER, REAXYS, REAXYS_BIOCATALYSIS database, our tool offers high-accuracy predictions, reflecting the latest in chemical research and data.
Strategy Settings
Precursor scoring | Relevance Heuristic |
---|---|
Min. plausibility | 0.01 |
Model | Template_relevance |
Template Set | Pistachio/Bkms_metabolic/Pistachio_ringbreaker/Reaxys/Reaxys_biocatalysis |
Top-N result to add to graph | 6 |
Feasible Synthetic Routes
Haftungsausschluss und Informationen zu In-Vitro-Forschungsprodukten
Bitte beachten Sie, dass alle Artikel und Produktinformationen, die auf BenchChem prĂ€sentiert werden, ausschlieĂlich zu Informationszwecken bestimmt sind. Die auf BenchChem zum Kauf angebotenen Produkte sind speziell fĂŒr In-vitro-Studien konzipiert, die auĂerhalb lebender Organismen durchgefĂŒhrt werden. In-vitro-Studien, abgeleitet von dem lateinischen Begriff "in Glas", beinhalten Experimente, die in kontrollierten Laborumgebungen unter Verwendung von Zellen oder Geweben durchgefĂŒhrt werden. Es ist wichtig zu beachten, dass diese Produkte nicht als Arzneimittel oder Medikamente eingestuft sind und keine Zulassung der FDA fĂŒr die Vorbeugung, Behandlung oder Heilung von medizinischen ZustĂ€nden, Beschwerden oder Krankheiten erhalten haben. Wir mĂŒssen betonen, dass jede Form der körperlichen EinfĂŒhrung dieser Produkte in Menschen oder Tiere gesetzlich strikt untersagt ist. Es ist unerlĂ€sslich, sich an diese Richtlinien zu halten, um die Einhaltung rechtlicher und ethischer Standards in Forschung und Experiment zu gewĂ€hrleisten.