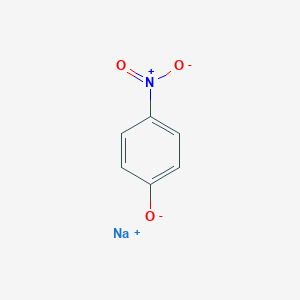
Natrium-4-nitrophenolat
Übersicht
Beschreibung
Sodium 4-nitrophenolate is an organic compound with the molecular formula C6H4NO3Na. It is the sodium salt of 4-nitrophenol, a compound known for its yellow crystalline appearance. Sodium 4-nitrophenolate is widely used in various scientific and industrial applications due to its unique chemical properties, including its role as an intermediate in organic synthesis and its use in the preparation of dyes and pigments.
Wirkmechanismus
Target of Action
Sodium 4-nitrophenolate primarily targets the optical properties of certain materials . It is used in the growth of single crystals, which are essential for various optical applications . The compound also plays a role in the catalytic reduction of 4-nitrophenol by nanostructured materials .
Mode of Action
Sodium 4-nitrophenolate interacts with its targets through a delocalized electron mechanism , which enhances the nonlinear optical (NLO) properties of the materials . This interaction results in the enhancement of the second and third harmonic generation efficiencies of the grown crystals . In the catalytic reduction process, sodium 4-nitrophenolate likely interacts with the reducing agents, such as NaBH4, to facilitate the reduction of 4-nitrophenol .
Biochemical Pathways
The compound affects the nitrate reduction pathway . It has been found to upregulate nitrate reductase, a key enzyme in this pathway . This leads to enhanced denitrification efficiency in certain bacterial strains .
Pharmacokinetics
The compound’s optical properties and its role in the growth of single crystals suggest that it may have unique physicochemical characteristics .
Result of Action
The action of Sodium 4-nitrophenolate results in enhanced optical properties of the materials it interacts with . For instance, it improves the optical transmittance in the visible and NIR regions of the grown crystals . In terms of its role in catalytic reduction, the compound enhances the efficiency of 4-nitrophenol reduction .
Action Environment
Environmental factors can influence the action of Sodium 4-nitrophenolate. For instance, the compound’s effectiveness in enhancing optical properties or catalytic reduction efficiency may vary depending on the specific conditions of the environment
Wissenschaftliche Forschungsanwendungen
Sodium 4-nitrophenolate has numerous applications in scientific research, including:
Chemistry: It is used as an intermediate in the synthesis of various organic compounds, including dyes, pigments, and pharmaceuticals.
Biology: Sodium 4-nitrophenolate is used in enzymatic assays to study enzyme kinetics and mechanisms.
Medicine: It serves as a precursor in the synthesis of drugs and other therapeutic agents.
Industry: The compound is used in the production of dyes, pigments, and other industrial chemicals.
Biochemische Analyse
Cellular Effects
It’s believed that Sodium 4-nitrophenolate could influence cell function, potentially impacting cell signaling pathways, gene expression, and cellular metabolism .
Molecular Mechanism
It’s thought that Sodium 4-nitrophenolate could exert its effects at the molecular level through binding interactions with biomolecules, enzyme inhibition or activation, and changes in gene expression .
Temporal Effects in Laboratory Settings
In laboratory settings, the effects of Sodium 4-nitrophenolate may change over time. This could include information on the product’s stability, degradation, and any long-term effects on cellular function observed in in vitro or in vivo studies .
Dosage Effects in Animal Models
The effects of Sodium 4-nitrophenolate can vary with different dosages in animal models. This could include any threshold effects observed in these studies, as well as any toxic or adverse effects at high doses .
Metabolic Pathways
Sodium 4-nitrophenolate may be involved in various metabolic pathways. This could include any enzymes or cofactors that it interacts with, as well as any effects on metabolic flux or metabolite levels .
Transport and Distribution
This could include any transporters or binding proteins that it interacts with, as well as any effects on its localization or accumulation .
Subcellular Localization
This could include any targeting signals or post-translational modifications that direct it to specific compartments or organelles .
Vorbereitungsmethoden
Synthetic Routes and Reaction Conditions: Sodium 4-nitrophenolate can be synthesized through the neutralization of 4-nitrophenol with sodium hydroxide. The reaction is typically carried out in an aqueous medium, where 4-nitrophenol is dissolved in water, and sodium hydroxide is added gradually until the pH reaches a neutral level. The resulting solution is then evaporated to obtain sodium 4-nitrophenolate in its solid form.
Industrial Production Methods: In industrial settings, sodium 4-nitrophenolate is produced on a larger scale using similar neutralization methods. The process involves the careful control of reaction conditions, such as temperature and concentration, to ensure high yield and purity of the final product. The industrial production also includes steps for purification and crystallization to obtain sodium 4-nitrophenolate with the desired specifications.
Analyse Chemischer Reaktionen
Types of Reactions: Sodium 4-nitrophenolate undergoes various chemical reactions, including:
Reduction: Sodium 4-nitrophenolate can be reduced to 4-aminophenol using reducing agents such as sodium borohydride. This reaction is commonly used in the synthesis of pharmaceuticals and other organic compounds.
Substitution: The nitro group in sodium 4-nitrophenolate can be substituted with other functional groups through nucleophilic substitution reactions. This property makes it a valuable intermediate in organic synthesis.
Oxidation: Although less common, sodium 4-nitrophenolate can undergo oxidation reactions under specific conditions to form different oxidation products.
Common Reagents and Conditions:
Reducing Agents: Sodium borohydride, hydrogen gas with a catalyst.
Substitution Reagents: Various nucleophiles, such as amines and thiols.
Oxidizing Agents: Potassium permanganate, hydrogen peroxide.
Major Products:
Reduction: 4-Aminophenol.
Substitution: Various substituted phenols depending on the nucleophile used.
Oxidation: Oxidized phenolic compounds.
Vergleich Mit ähnlichen Verbindungen
Sodium 4-nitrophenolate can be compared with other similar compounds, such as:
Sodium 2-nitrophenolate: Similar in structure but with the nitro group in the ortho position, leading to different reactivity and applications.
Sodium 3-nitrophenolate: The nitro group is in the meta position, affecting its chemical properties and uses.
Sodium 4-aminophenolate: The reduced form of sodium 4-nitrophenolate, used in different applications due to the presence of the amino group.
Uniqueness: Sodium 4-nitrophenolate is unique due to its specific positioning of the nitro group, which influences its reactivity and makes it suitable for a wide range of chemical reactions and applications.
Biologische Aktivität
Sodium 4-nitrophenolate, a sodium salt of 4-nitrophenol, is recognized for its diverse biological activities, particularly in agriculture as a plant growth regulator and in various biochemical applications. This article explores its biological mechanisms, effects on plant physiology, and relevant case studies, supported by comprehensive research findings.
Sodium 4-nitrophenolate has the chemical formula and is often represented as a white crystalline solid. It is soluble in water and exhibits properties that make it useful in both agricultural and laboratory settings.
Sodium 4-nitrophenolate acts primarily as a plant growth regulator through several mechanisms:
- Hormonal Regulation : It influences plant growth by modulating the levels of plant hormones such as auxins. The compound inhibits indole-3-acetic acid (IAA) oxidase, leading to increased IAA levels, which promote cell elongation and division .
- Nutrient Uptake Enhancement : Studies indicate that sodium 4-nitrophenolate enhances nutrient absorption in plants, improving their overall health and productivity .
- Stress Tolerance : The compound has been shown to increase plant resilience against environmental stresses such as drought and salinity by improving root development and water uptake efficiency .
Biological Effects on Plants
The biological activity of sodium 4-nitrophenolate manifests in various ways:
- Increased Biomass Accumulation : Application of sodium 4-nitrophenolate has been associated with significant increases in biomass for crops like oilseed rape and cucumber. In field trials, treated plants exhibited higher yields compared to controls .
- Enhanced Photosynthesis : The compound positively affects the photosynthetic apparatus, thereby increasing photosynthetic efficiency and transpiration rates without compromising water content .
- Improved Flowering and Fruit Set : Sodium 4-nitrophenolate regulates hormonal balance, leading to improved flowering rates and fruit set in various crops .
Case Study 1: Oilseed Rape
In a field study conducted over two growing seasons, oilseed rape plants treated with sodium 4-nitrophenolate showed:
Parameter | Control Group | Treated Group |
---|---|---|
Plant Height (cm) | 85 | 90 |
Pods per Plant | 15 | 16 |
Seeds per Pod | 30 | 32 |
The results indicated that treated plants had taller stature and increased reproductive success compared to controls .
Case Study 2: Cucumber Growth
Research on cucumber plants demonstrated that those treated with sodium 4-nitrophenolate had:
Growth Parameter | Control Group | Treated Group |
---|---|---|
Biomass (g) | 50 | 65 |
Leaf Area (cm²) | 200 | 250 |
This study highlighted the effectiveness of sodium 4-nitrophenolate in promoting vegetative growth under controlled conditions .
Safety and Environmental Impact
While sodium 4-nitrophenolate is effective for agricultural purposes, its safety profile is crucial. It is classified with certain hazards, including potential skin irritation and respiratory effects upon inhalation. However, it is noted for its environmental compatibility as it degrades into non-toxic byproducts in soil ecosystems .
Eigenschaften
CAS-Nummer |
824-78-2 |
---|---|
Molekularformel |
C6H5NNaO3 |
Molekulargewicht |
162.10 g/mol |
IUPAC-Name |
sodium;4-nitrophenolate |
InChI |
InChI=1S/C6H5NO3.Na/c8-6-3-1-5(2-4-6)7(9)10;/h1-4,8H; |
InChI-Schlüssel |
OORLTLMFPORJLV-UHFFFAOYSA-N |
SMILES |
C1=CC(=CC=C1[N+](=O)[O-])[O-].[Na+] |
Kanonische SMILES |
C1=CC(=CC=C1[N+](=O)[O-])O.[Na] |
Color/Form |
Yellow crystalline solid |
Key on ui other cas no. |
824-78-2 |
Piktogramme |
Flammable; Irritant; Health Hazard; Environmental Hazard |
Verwandte CAS-Nummern |
100-02-7 (Parent) |
Löslichkeit |
Sol in wate |
Synonyme |
Sodium-4-nitrophenoxide |
Herkunft des Produkts |
United States |
Retrosynthesis Analysis
AI-Powered Synthesis Planning: Our tool employs the Template_relevance Pistachio, Template_relevance Bkms_metabolic, Template_relevance Pistachio_ringbreaker, Template_relevance Reaxys, Template_relevance Reaxys_biocatalysis model, leveraging a vast database of chemical reactions to predict feasible synthetic routes.
One-Step Synthesis Focus: Specifically designed for one-step synthesis, it provides concise and direct routes for your target compounds, streamlining the synthesis process.
Accurate Predictions: Utilizing the extensive PISTACHIO, BKMS_METABOLIC, PISTACHIO_RINGBREAKER, REAXYS, REAXYS_BIOCATALYSIS database, our tool offers high-accuracy predictions, reflecting the latest in chemical research and data.
Strategy Settings
Precursor scoring | Relevance Heuristic |
---|---|
Min. plausibility | 0.01 |
Model | Template_relevance |
Template Set | Pistachio/Bkms_metabolic/Pistachio_ringbreaker/Reaxys/Reaxys_biocatalysis |
Top-N result to add to graph | 6 |
Feasible Synthetic Routes
Haftungsausschluss und Informationen zu In-Vitro-Forschungsprodukten
Bitte beachten Sie, dass alle Artikel und Produktinformationen, die auf BenchChem präsentiert werden, ausschließlich zu Informationszwecken bestimmt sind. Die auf BenchChem zum Kauf angebotenen Produkte sind speziell für In-vitro-Studien konzipiert, die außerhalb lebender Organismen durchgeführt werden. In-vitro-Studien, abgeleitet von dem lateinischen Begriff "in Glas", beinhalten Experimente, die in kontrollierten Laborumgebungen unter Verwendung von Zellen oder Geweben durchgeführt werden. Es ist wichtig zu beachten, dass diese Produkte nicht als Arzneimittel oder Medikamente eingestuft sind und keine Zulassung der FDA für die Vorbeugung, Behandlung oder Heilung von medizinischen Zuständen, Beschwerden oder Krankheiten erhalten haben. Wir müssen betonen, dass jede Form der körperlichen Einführung dieser Produkte in Menschen oder Tiere gesetzlich strikt untersagt ist. Es ist unerlässlich, sich an diese Richtlinien zu halten, um die Einhaltung rechtlicher und ethischer Standards in Forschung und Experiment zu gewährleisten.