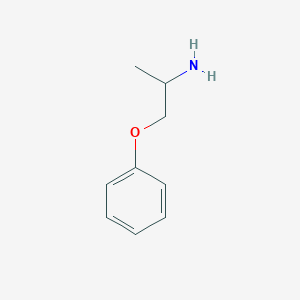
1-Phenoxypropan-2-amin
Übersicht
Beschreibung
1-Methyl-2-phenoxyethylamine is a useful research compound. Its molecular formula is C9H13NO and its molecular weight is 151.21 g/mol. The purity is usually 95%.
The exact mass of the compound 1-Methyl-2-phenoxyethylamine is unknown and the complexity rating of the compound is unknown. The compound has been submitted to the National Cancer Institute (NCI) for testing and evaluation and the Cancer Chemotherapy National Service Center (NSC) number is 137777. The United Nations designated GHS hazard class pictogram is Corrosive;Irritant, and the GHS signal word is DangerThe storage condition is unknown. Please store according to label instructions upon receipt of goods.
BenchChem offers high-quality 1-Methyl-2-phenoxyethylamine suitable for many research applications. Different packaging options are available to accommodate customers' requirements. Please inquire for more information about 1-Methyl-2-phenoxyethylamine including the price, delivery time, and more detailed information at info@benchchem.com.
Wissenschaftliche Forschungsanwendungen
Life Science Research
1-Phenoxypropan-2-amin wird in der Life-Science-Forschung zur Synthese von bioaktiven Molekülen verwendet. Seine Fähigkeit, eine Phenoxyethylamin-Gruppierung in größere Strukturen einzuführen, ist wertvoll für die Herstellung von Verbindungen mit potenzieller biologischer Aktivität. Dies ist besonders relevant bei der Entwicklung neuer Arzneimittel, bei denen Modifikationen der Molekülstruktur zu signifikanten Veränderungen der Wirksamkeit und Pharmakokinetik eines Arzneimittels führen können .
Material Science
In der Materialwissenschaft dient diese Verbindung als Vorläufer für die Synthese von Polymeren und Harzen. Die Phenoxygruppe kann mit anderen Komponenten interagieren, um stabile Polymerketten zu bilden, die nützlich sind bei der Herstellung von Materialien mit spezifischen mechanischen und chemischen Eigenschaften. Diese Materialien können in verschiedenen Industrien eingesetzt werden, darunter Automobil, Luft- und Raumfahrt und Konsumgüter .
Chemical Synthesis
This compound ist ein wichtiges Zwischenprodukt in der organischen Synthese. Es kann verschiedene chemische Reaktionen eingehen, wie z. B. Alkylierung, Acylierung und Oxidation, um eine Vielzahl von Derivaten zu erhalten. Diese Derivate sind entscheidend für die Synthese komplexer organischer Moleküle, die in Farbstoffen, Duftstoffen und Pflanzenschutzmitteln verwendet werden .
Chromatography
Diese Verbindung kann aufgrund ihrer einzigartigen chemischen Eigenschaften in der Chromatographie als Standard- oder Referenzverbindung verwendet werden. Sie hilft bei der Kalibrierung chromatographischer Systeme und dient als Vergleichspunkt für die Identifizierung und Quantifizierung ähnlicher Verbindungen in komplexen Gemischen .
Analytical Chemistry
In der analytischen Chemie wird this compound bei der Entwicklung analytischer Methoden eingesetzt. Es kann als Reagenz oder Reaktant bei der Detektion und Quantifizierung verschiedener chemischer Spezies dienen. Seine Reaktivität macht es für den Einsatz in spektrophotometrischen und elektrochemischen Analysen geeignet .
Biocatalysis
Die Verbindung wird in biokatalytischen Prozessen eingesetzt, insbesondere bei der Entwicklung von chiralen Amiden. Es dient als Substrat für Amintransaminase-Lipase-Kaskaden, die innovative Verfahren zur Synthese von chiralen Verbindungen unter Fließbedingungen sind. Diese Anwendung ist entscheidend für die pharmazeutische Industrie, in der die Herstellung enantiomerenreiner Substanzen unerlässlich ist .
Pharmaceutical Development
This compound ist an der Entwicklung von Arzneimitteln beteiligt, insbesondere bei der Herstellung neuer Wirkstoffe für das Zentralnervensystem (ZNS). Seine strukturelle Ähnlichkeit mit Phenethylamin, einer Verbindung, die in verschiedenen Neurotransmittern vorkommt, macht es zu einem wertvollen Gerüst für die Entwicklung von Medikamenten, die auf ZNS-Erkrankungen abzielen .
Environmental Science
In der Umweltwissenschaft werden die Derivate dieser Verbindung auf ihr Potenzial als umweltfreundliche Pestizide und Herbizide untersucht. Die Forschung konzentriert sich auf die Entwicklung von Verbindungen, die gegen Schädlinge wirksam sind, aber nur minimale Auswirkungen auf Nicht-Zielarten und Ökosysteme haben .
Wirkmechanismus
Target of Action
1-Phenoxypropan-2-amine, also known as 1-Methyl-2-phenoxyethylamine or 1-Phenoxy-2-propanamine, is primarily targeted by transaminases . Transaminases are enzymes that catalyze the transfer of an amino group from one molecule to another, playing a crucial role in amino acid metabolism .
Mode of Action
The compound interacts with its target, the transaminase enzyme, in a process known as transamination . In this process, the enzyme converts a prochiral ketone into 1-phenoxypropan-2-amine . This reaction is facilitated by an amine donor, such as isopropylamine .
Biochemical Pathways
The transamination process involving 1-phenoxypropan-2-amine affects the amino acid metabolism pathway . The conversion of prochiral ketones to 1-phenoxypropan-2-amine results in the production of novel disubstituted 1-phenylpropan-2-amines . These compounds are pharmaceutically relevant and can be used in the synthesis of various drugs .
Result of Action
The result of the action of 1-phenoxypropan-2-amine involves the production of enantiopure, pharmaceutically relevant disubstituted 1-phenylpropan-2-amines . These compounds have potential applications in the pharmaceutical industry, particularly in the synthesis of various drugs .
Action Environment
The action of 1-phenoxypropan-2-amine is influenced by various environmental factors. For instance, the transamination process is facilitated by the presence of an amine donor . Additionally, the use of immobilized whole-cell biocatalysts with ®-transaminase activity has been reported to enhance the synthesis of the compound . .
Biochemische Analyse
Biochemical Properties
For instance, it can be converted from 1-phenoxypropan-2-one using isopropylamine as an amine donor
Cellular Effects
For example, primary amine-containing compounds exhibit high Golgi localization with no toxicity
Molecular Mechanism
It’s known that this compound can participate in enzymatic reactions, such as the conversion from 1-phenoxypropan-2-one
Biologische Aktivität
1-Methyl-2-phenoxyethylamine (MPEA) is an organic compound with potential biological activity that has garnered interest in pharmacological research. This article delves into its biological properties, mechanisms of action, and relevant case studies, supported by data tables and research findings.
Chemical Profile
- Molecular Formula : C10H15NO
- Molecular Weight : 165.24 g/mol
- IUPAC Name : 1-Methyl-2-phenoxyethanamine
- Classification : Secondary amine
MPEA features a phenoxy group, which contributes to its unique chemical properties and potential interactions with biological systems.
Neurotransmitter Interaction
Research indicates that MPEA may interact with various neurotransmitter systems, particularly in the central nervous system (CNS). Preliminary studies suggest that it could modulate neurotransmission, potentially affecting conditions such as anxiety and depression. Specifically, it may act as a substrate or inhibitor for certain enzymes involved in amino acid metabolism, particularly transaminases, which are crucial for neurotransmitter synthesis .
Antimicrobial Properties
MPEA has demonstrated antimicrobial activity in laboratory settings. In vitro studies have shown its effectiveness against a range of bacterial strains, suggesting its potential as a lead compound for developing new antimicrobial agents. The mechanism of action appears to involve disruption of bacterial cell wall synthesis .
Case Studies
- Neuropharmacological Effects : A study evaluated the effects of MPEA on neurotransmitter levels in animal models. Results indicated that administration of MPEA led to increased levels of serotonin and norepinephrine, suggesting its potential use in treating mood disorders .
- Antimicrobial Efficacy : In a controlled study, MPEA was tested against Staphylococcus aureus and Escherichia coli. The compound exhibited significant inhibitory effects on both strains, with minimum inhibitory concentrations (MICs) recorded at 32 µg/mL for S. aureus and 64 µg/mL for E. coli .
The biological activity of MPEA is thought to stem from its ability to interact with specific receptors and enzymes:
- Receptor Interaction : MPEA may bind to adrenergic receptors, influencing cardiovascular responses and neurotransmitter release.
- Enzyme Inhibition : Its interaction with transaminases suggests a role in modulating metabolic pathways related to amino acids and neurotransmitters .
Data Table: Summary of Biological Activities
Eigenschaften
IUPAC Name |
1-phenoxypropan-2-amine | |
---|---|---|
Source | PubChem | |
URL | https://pubchem.ncbi.nlm.nih.gov | |
Description | Data deposited in or computed by PubChem | |
InChI |
InChI=1S/C9H13NO/c1-8(10)7-11-9-5-3-2-4-6-9/h2-6,8H,7,10H2,1H3 | |
Source | PubChem | |
URL | https://pubchem.ncbi.nlm.nih.gov | |
Description | Data deposited in or computed by PubChem | |
InChI Key |
IKYFHRVPKIFGMH-UHFFFAOYSA-N | |
Source | PubChem | |
URL | https://pubchem.ncbi.nlm.nih.gov | |
Description | Data deposited in or computed by PubChem | |
Canonical SMILES |
CC(COC1=CC=CC=C1)N | |
Source | PubChem | |
URL | https://pubchem.ncbi.nlm.nih.gov | |
Description | Data deposited in or computed by PubChem | |
Molecular Formula |
C9H13NO | |
Source | PubChem | |
URL | https://pubchem.ncbi.nlm.nih.gov | |
Description | Data deposited in or computed by PubChem | |
DSSTOX Substance ID |
DTXSID301313534 | |
Record name | 1-Phenoxy-2-propanamine | |
Source | EPA DSSTox | |
URL | https://comptox.epa.gov/dashboard/DTXSID301313534 | |
Description | DSSTox provides a high quality public chemistry resource for supporting improved predictive toxicology. | |
Molecular Weight |
151.21 g/mol | |
Source | PubChem | |
URL | https://pubchem.ncbi.nlm.nih.gov | |
Description | Data deposited in or computed by PubChem | |
CAS No. |
35205-54-0 | |
Record name | 1-Phenoxy-2-propanamine | |
Source | CAS Common Chemistry | |
URL | https://commonchemistry.cas.org/detail?cas_rn=35205-54-0 | |
Description | CAS Common Chemistry is an open community resource for accessing chemical information. Nearly 500,000 chemical substances from CAS REGISTRY cover areas of community interest, including common and frequently regulated chemicals, and those relevant to high school and undergraduate chemistry classes. This chemical information, curated by our expert scientists, is provided in alignment with our mission as a division of the American Chemical Society. | |
Explanation | The data from CAS Common Chemistry is provided under a CC-BY-NC 4.0 license, unless otherwise stated. | |
Record name | Ethylamine, 1-methyl-2-phenoxy- | |
Source | ChemIDplus | |
URL | https://pubchem.ncbi.nlm.nih.gov/substance/?source=chemidplus&sourceid=0035205540 | |
Description | ChemIDplus is a free, web search system that provides access to the structure and nomenclature authority files used for the identification of chemical substances cited in National Library of Medicine (NLM) databases, including the TOXNET system. | |
Record name | 35205-54-0 | |
Source | DTP/NCI | |
URL | https://dtp.cancer.gov/dtpstandard/servlet/dwindex?searchtype=NSC&outputformat=html&searchlist=137777 | |
Description | The NCI Development Therapeutics Program (DTP) provides services and resources to the academic and private-sector research communities worldwide to facilitate the discovery and development of new cancer therapeutic agents. | |
Explanation | Unless otherwise indicated, all text within NCI products is free of copyright and may be reused without our permission. Credit the National Cancer Institute as the source. | |
Record name | 1-Phenoxy-2-propanamine | |
Source | EPA DSSTox | |
URL | https://comptox.epa.gov/dashboard/DTXSID301313534 | |
Description | DSSTox provides a high quality public chemistry resource for supporting improved predictive toxicology. | |
Record name | 1-methyl-2-phenoxyethylamine | |
Source | European Chemicals Agency (ECHA) | |
URL | https://echa.europa.eu/substance-information/-/substanceinfo/100.047.653 | |
Description | The European Chemicals Agency (ECHA) is an agency of the European Union which is the driving force among regulatory authorities in implementing the EU's groundbreaking chemicals legislation for the benefit of human health and the environment as well as for innovation and competitiveness. | |
Explanation | Use of the information, documents and data from the ECHA website is subject to the terms and conditions of this Legal Notice, and subject to other binding limitations provided for under applicable law, the information, documents and data made available on the ECHA website may be reproduced, distributed and/or used, totally or in part, for non-commercial purposes provided that ECHA is acknowledged as the source: "Source: European Chemicals Agency, http://echa.europa.eu/". Such acknowledgement must be included in each copy of the material. ECHA permits and encourages organisations and individuals to create links to the ECHA website under the following cumulative conditions: Links can only be made to webpages that provide a link to the Legal Notice page. | |
Synthesis routes and methods
Procedure details
Retrosynthesis Analysis
AI-Powered Synthesis Planning: Our tool employs the Template_relevance Pistachio, Template_relevance Bkms_metabolic, Template_relevance Pistachio_ringbreaker, Template_relevance Reaxys, Template_relevance Reaxys_biocatalysis model, leveraging a vast database of chemical reactions to predict feasible synthetic routes.
One-Step Synthesis Focus: Specifically designed for one-step synthesis, it provides concise and direct routes for your target compounds, streamlining the synthesis process.
Accurate Predictions: Utilizing the extensive PISTACHIO, BKMS_METABOLIC, PISTACHIO_RINGBREAKER, REAXYS, REAXYS_BIOCATALYSIS database, our tool offers high-accuracy predictions, reflecting the latest in chemical research and data.
Strategy Settings
Precursor scoring | Relevance Heuristic |
---|---|
Min. plausibility | 0.01 |
Model | Template_relevance |
Template Set | Pistachio/Bkms_metabolic/Pistachio_ringbreaker/Reaxys/Reaxys_biocatalysis |
Top-N result to add to graph | 6 |
Feasible Synthetic Routes
Q1: Why was 1-Methyl-2-phenoxyethylamine chosen as the internal standard for quantifying 3-amino-1-phenylbutane (APB) in urine?
A1: While the research paper [] doesn't explicitly explain the rationale behind choosing 1-Methyl-2-phenoxyethylamine as the internal standard, several factors likely contributed to this decision. Ideal internal standards share similar chemical properties and chromatographic behavior with the analyte of interest. It's plausible that 1-Methyl-2-phenoxyethylamine possesses a similar structure and polarity to APB, allowing for comparable extraction efficiencies and similar retention times during gas chromatography. This similarity helps ensure accurate and reliable quantification of APB in urine samples.
Q2: What specific analytical method was used to quantify 1-Methyl-2-phenoxyethylamine and how was the method validated?
A2: The researchers used a highly specific and sensitive method called gas chromatography/mass spectrometry (GC/MS) to quantify both 1-Methyl-2-phenoxyethylamine (internal standard) and 3-amino-1-phenylbutane (APB) []. Before analysis, urine samples underwent alkalization and extraction with ether. The extracted compounds, including the internal standard, were derivatized with a chiral reagent to enable the separation and quantification of enantiomers. Detection was achieved using electron capture negative ion chemical ionization mass spectrometry, specifically targeting the [M--32]- ions of both the analyte and internal standard.
Haftungsausschluss und Informationen zu In-Vitro-Forschungsprodukten
Bitte beachten Sie, dass alle Artikel und Produktinformationen, die auf BenchChem präsentiert werden, ausschließlich zu Informationszwecken bestimmt sind. Die auf BenchChem zum Kauf angebotenen Produkte sind speziell für In-vitro-Studien konzipiert, die außerhalb lebender Organismen durchgeführt werden. In-vitro-Studien, abgeleitet von dem lateinischen Begriff "in Glas", beinhalten Experimente, die in kontrollierten Laborumgebungen unter Verwendung von Zellen oder Geweben durchgeführt werden. Es ist wichtig zu beachten, dass diese Produkte nicht als Arzneimittel oder Medikamente eingestuft sind und keine Zulassung der FDA für die Vorbeugung, Behandlung oder Heilung von medizinischen Zuständen, Beschwerden oder Krankheiten erhalten haben. Wir müssen betonen, dass jede Form der körperlichen Einführung dieser Produkte in Menschen oder Tiere gesetzlich strikt untersagt ist. Es ist unerlässlich, sich an diese Richtlinien zu halten, um die Einhaltung rechtlicher und ethischer Standards in Forschung und Experiment zu gewährleisten.