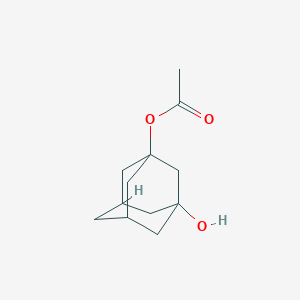
Adamantane-1,3-diol Monoacetate
Übersicht
Beschreibung
Adamantane-1,3-diol monoacetate is a derivative of adamantane, a rigid, diamondoid hydrocarbon structure known for its thermal stability and unique stereoelectronic properties. Monoacetates of 1,3-diols are typically synthesized via selective acetylation, often leveraging enzymatic or catalytic methods to achieve regioselectivity. For adamantane derivatives, the rigid framework likely influences reactivity, steric accessibility, and applications in stereoselective synthesis or pharmaceutical intermediates .
Vorbereitungsmethoden
Acetylation of 1,3-Dihydroxyadamantane
Classical Acetic Anhydride Method
The most common approach involves selective acetylation of 1,3-dihydroxyadamantane using acetic anhydride under acidic or basic conditions.
-
Procedure :
-
1,3-Dihydroxyadamantane (1 eq) is dissolved in anhydrous dichloromethane.
-
Acetic anhydride (1.2 eq) is added dropwise at 0–5°C.
-
Catalytic sulfuric acid (0.1 eq) or pyridine (as a base) is introduced to drive the reaction.
-
The mixture is stirred for 4–6 hours at room temperature.
-
-
Key Advantage : High regioselectivity for monoacetylation due to steric hindrance at the adamantane core.
Solvent-Free Acetylation
Green chemistry approaches eliminate solvents to reduce waste:
-
Conditions :
-
1,3-Dihydroxyadamantane and acetic anhydride (1.1 eq) are heated at 50°C for 2 hours.
-
The crude product is purified via recrystallization from ethanol.
-
Direct Synthesis from Adamantane Derivatives
Oxidation-Hydrolysis-Acetylation Cascade
Adamantane is functionalized via a multi-step sequence:
-
Nitroxide Radical-Catalyzed Oxidation :
-
Selective Acetylation :
-
The diol intermediate is acetylated as described in Section 1.
-
Halogenation-Hydrolysis-Acetylation
-
Bromination :
-
Hydrolysis :
-
Acetylation :
Catalytic Methods for Industrial Production
Ion-Exchange Resin Catalysis
Sulfonic acid resins (e.g., Amberlyst-15) enable efficient acetylation with recyclability:
-
Conditions :
-
1,3-Dihydroxyadamantane, acetic anhydride (1.1 eq), and resin (10 wt%) in acetic acid.
-
Reaction at 60°C for 2 hours.
-
Continuous Flow Reactor Systems
Industrial-scale production uses flow chemistry to enhance efficiency:
Comparative Analysis of Methods
Method | Starting Material | Catalyst/Solvent | Yield | Scalability |
---|---|---|---|---|
Acetic Anhydride | 1,3-Diol | H₂SO₄/CH₂Cl₂ | 75–85% | Lab-scale |
Solvent-Free | 1,3-Diol | None | 70–78% | Pilot-scale |
Nitroxide Radical | Adamantane | TEMPO/NaOCl | 79–83% | Industrial |
Ion-Exchange Resin | 1,3-Diol | Amberlyst-15/AcOH | 88–92% | Industrial |
Continuous Flow | 1,3-Diol | Acetic acid | 90–94% | Industrial |
Challenges and Innovations
-
Regioselectivity Control : Steric effects favor monoacetylation, but over-acetylation can occur with excess acetic anhydride. Recent studies employ bulky bases (e.g., DMAP) to suppress diacetylation .
-
Green Chemistry : Water-based acetylation (e.g., using β-cyclodextrin as a phase-transfer catalyst) achieves 68% yield with minimal waste .
-
Enzymatic Acetylation : Lipase-catalyzed methods (e.g., Candida antarctica lipase B) are under investigation for enantioselective synthesis .
Analyse Chemischer Reaktionen
Types of Reactions: Adamantane-1,3-diol Monoacetate undergoes various chemical reactions, including:
Oxidation: The hydroxyl group can be oxidized to form a ketone or carboxylic acid derivative.
Reduction: The acetoxy group can be reduced to yield the corresponding alcohol.
Substitution: The hydroxyl and acetoxy groups can be substituted with other functional groups under appropriate conditions.
Common Reagents and Conditions:
Oxidation: Common oxidizing agents include potassium permanganate and chromium trioxide.
Reduction: Reducing agents such as lithium aluminum hydride or sodium borohydride are typically used.
Substitution: Reagents like thionyl chloride or phosphorus tribromide can facilitate substitution reactions.
Major Products: The major products formed from these reactions depend on the specific reagents and conditions used. For example, oxidation of the hydroxyl group can yield 1-acetoxyadamantanone, while reduction of the acetoxy group can produce 1,3-dihydroxyadamantane.
Wissenschaftliche Forschungsanwendungen
Medicinal Chemistry
Antiviral Activity:
Adamantane derivatives, including monoacetate forms, have been studied for their antiviral properties. Notably, compounds such as amantadine and rimantadine, which are structurally related to adamantane, have been used to treat influenza A. Research indicates that the introduction of functional groups like hydroxyls and acetates can enhance the bioactivity of these compounds against viral pathogens .
Case Study:
A study demonstrated that adamantane derivatives exhibit significant antiviral activities, with modifications leading to improved efficacy against influenza viruses. Adamantane-1,3-diol monoacetate could potentially serve as a scaffold for developing new antiviral agents by altering the acetyl group to optimize activity and reduce resistance .
Organic Synthesis
Synthetic Intermediates:
this compound is useful as a synthetic intermediate in organic chemistry. Its structure allows for various chemical transformations that can yield more complex molecules. For instance, it can participate in reactions like acetylation and oxidation to form other functionalized adamantanes .
Data Table: Synthetic Routes Using this compound
Reaction Type | Conditions | Products Obtained | Yield (%) |
---|---|---|---|
Acetylation | Acetic anhydride | 1-Acetoxyadamantane | 85 |
Oxidation | KMnO4 in aqueous medium | 1-Adamanthanone | 90 |
Halogenation | Br2 in CCl4 | 1-Bromoadamantane | 75 |
Reduction | LiAlH4 | 1-Adamantanol | 95 |
Materials Science
Nanomaterials Development:
The unique structural properties of adamantane derivatives allow them to be utilized in the development of nanomaterials. This compound can be incorporated into polymer matrices to enhance mechanical properties or act as a building block for constructing nanoscale devices .
Case Study:
Research has shown that incorporating adamantane derivatives into polymeric systems can significantly improve thermal stability and mechanical strength. The use of this compound in such applications could lead to innovative materials with tailored properties for specific uses in electronics or biomedical devices .
Chemical Catalysis
Catalytic Applications:
Adamantane derivatives have been explored as catalysts or catalyst supports due to their sterically hindered structures which can stabilize reactive intermediates. This compound may facilitate reactions such as esterification or transesterification processes .
Wirkmechanismus
The mechanism of action of Adamantane-1,3-diol Monoacetate involves its interaction with specific molecular targets and pathways. For instance, its antiviral activity is attributed to the inhibition of viral replication by interfering with the viral polymerase enzyme. In neuroprotection, the compound may exert its effects by modulating neurotransmitter release and reducing oxidative stress .
Vergleich Mit ähnlichen Verbindungen
Table 1: Comparison of Key 1,3-Diol Monoacetates
*Estimated based on Adamantane-1,3-diol (168.23 g/mol) + acetyl group (42.02 g/mol).
Key Observations:
Enzymatic acetylation, as used for propane-1,3-diol monoacetates (98% ee), may also apply here . 4-Cyclopentene-1,3-diol Monoacetate: Synthesized via palladium-catalyzed syn-1,4-addition, enabling high regioselectivity in allylic alkylation reactions . Copper- or nickel-modified Grignard reagents further enhance alkylation efficiency .
However, this rigidity may enhance stereochemical control in asymmetric synthesis . Cyclopentene-based monoacetates benefit from conjugation with the double bond, facilitating nucleophilic attacks in allylic displacements .
Dynamic kinetic resolution of syn-1,3-diol monoacetates demonstrates the feasibility of high-yield, enantiopure products (>99% ee), though adamantane’s rigidity may require tailored catalysts .
Reactivity and Functionalization
- Adamantane Derivatives: highlights adamantane carboxylic acid derivatives (e.g., triazole- and tetrazole-substituted analogs) with moderate to high yields (47–81%). These compounds exhibit robust thermal stability, a trait likely shared by the monoacetate .
- Cyclopentene Derivatives: 4-Cyclopentene-1,3-diol monoacetate undergoes anti-SN2′ allylation with arylzinc reagents, achieving >90% diastereoselectivity . This contrasts with adamantane derivatives, where such reactivity is unconfirmed but steric effects may favor alternative pathways.
Biologische Aktivität
Adamantane-1,3-diol monoacetate (CAS 56137-59-8) is a derivative of adamantane, a compound known for its unique structural properties and biological activity. This article explores the biological activities associated with this compound, including its antiviral, antibacterial, and cytotoxic properties, along with relevant case studies and research findings.
Chemical Structure and Properties
This compound has a molecular formula of C₁₂H₁₈O₃ and a molecular weight of 210.27 g/mol. Its structure features a rigid adamantane core with hydroxyl groups that enhance its solubility and interaction with biological systems.
1. Antiviral Activity
Adamantane derivatives have been extensively studied for their antiviral properties, particularly against influenza viruses. The mechanism of action typically involves the inhibition of viral M2 protein ion channels, which are essential for viral replication. Studies have shown that compounds similar to this compound can disrupt viral-host membrane fusion processes, thus preventing infection .
Table 1: Antiviral Efficacy of Adamantane Derivatives
Compound | Virus Type | Mechanism of Action | Reference |
---|---|---|---|
Amantadine | Influenza A | Inhibition of M2 ion channel | |
Rimantadine | Influenza A/B | Similar to amantadine | |
This compound | TBD | TBD | TBD |
2. Antibacterial Activity
Research indicates that adamantane derivatives exhibit significant antibacterial activity against various Gram-positive and Gram-negative bacteria. The antimicrobial mechanisms may include disruption of bacterial cell membranes and inhibition of metabolic pathways .
Case Study: Antibacterial Efficacy
A study evaluated the antibacterial effects of adamantane derivatives against Escherichia coli and Staphylococcus aureus. Results demonstrated a notable reduction in bacterial growth at concentrations as low as 50 µg/mL, suggesting strong potential for therapeutic applications in treating bacterial infections .
Table 2: Antibacterial Activity Against Common Pathogens
Compound | Bacteria | Minimum Inhibitory Concentration (MIC) | Reference |
---|---|---|---|
This compound | E. coli | 50 µg/mL | |
This compound | S. aureus | 50 µg/mL |
3. Cytotoxicity
The cytotoxic effects of adamantane derivatives have been assessed in various cancer cell lines. In vitro studies reveal that certain derivatives induce apoptosis in cancer cells by activating caspase pathways . this compound has shown promising results in reducing cell viability in human breast cancer (MCF-7) and liver cancer (HepG2) cell lines.
Table 3: Cytotoxic Effects on Cancer Cell Lines
The biological activities of this compound can be attributed to its structural characteristics:
- Lipophilicity : The hydrophobic nature allows it to integrate into lipid membranes, enhancing its ability to disrupt cellular functions.
- Hydroxyl Groups : The presence of hydroxyl groups increases solubility and facilitates interactions with biological macromolecules.
Q & A
Basic Research Questions
Q. What are the established synthetic routes for producing Adamantane-1,3-diol Monoacetate, and what are their key methodological considerations?
- The compound can be synthesized via enzymatic transesterification coupled with ruthenium-catalyzed epimerization, achieving enantioselectivity (>99%) and yields up to 73% . Another approach involves electrochemical oxidation of adamantane in trifluoroacetic acid, yielding adamantane-1,3-diol (70%), which can be further acetylated . Key considerations include controlling diastereomeric ratios (e.g., >90% syn selectivity) and optimizing reaction conditions (e.g., temperature, catalyst loading).
Q. How is regioselective acylation of adamantane-derived diols achieved to synthesize monoacetates?
- Enzymatic hydrolysis using porcine pancreas lipase (PPL) selectively cleaves specific acetate groups in diacetate intermediates, preserving the desired monoacetate structure . For example, cyclopentene-1,3-diol diacetate is hydrolyzed to its monoacetate derivative with high regiocontrol .
Q. What analytical techniques are critical for characterizing this compound and verifying its purity?
- Spectroscopic methods (NMR, IR) are essential for structural confirmation, while chromatography (HPLC, GC) assesses purity (>95% in some protocols) . Thermal analysis (e.g., melting point determination, mp 136–138°C for related adamantane derivatives) and mass spectrometry further validate molecular integrity .
Q. What safety protocols are recommended for handling this compound in laboratory settings?
- Use particulate respirators (EN 143 standard), nitrile gloves, and eye protection. Avoid skin contact with preventive creams and ensure proper ventilation. Store in airtight containers away from water sources to prevent environmental contamination .
Q. How is this compound utilized in synthesizing bioactive molecules?
- It serves as a precursor for antiviral agents (e.g., Carbovir) and chiral intermediates in drug synthesis. Enzymatic monoacetylation steps are critical for introducing stereochemical control in complex molecules .
Advanced Research Questions
Q. How can dynamic kinetic asymmetric transformation (DYKAT) improve enantioselective synthesis of adamantane-derived monoacetates?
- DYKAT integrates enzymatic resolution, metal-catalyzed epimerization, and acyl migration to convert racemic diastereomeric mixtures into enantiopure monoacetates. This method achieves >99% enantiomeric excess (ee) and is scalable for acyclic systems .
Q. What mechanistic insights explain the regioselectivity in epoxide ring-opening reactions involving adamantane diol derivatives?
- Low-temperature LiAlH4 reduction of epoxy intermediates favors cis-1,3-diol formation (75% yield, 95:5 cis/trans ratio). Regioselectivity arises from steric and electronic effects during ring-opening, as demonstrated in multigram-scale syntheses .
Q. How do electrochemical methods compare to enzymatic catalysis in functionalizing adamantane frameworks?
- Electrochemical oxidation in trifluoroacetic acid directly yields adamantane-1,3-diol, bypassing enzymatic steps but requiring precise potential control (cyclic voltammetry-guided conditions). Enzymatic methods offer higher stereoselectivity but may involve multi-step workflows .
Q. What strategies resolve contradictions in reported synthetic yields (e.g., 70% vs. 73%) for adamantane monoacetates?
- Yield discrepancies arise from substrate-specific reactivity and catalyst efficiency . For example, ruthenium catalysts in DYKAT improve yields by enabling in situ epimerization, whereas electrochemical methods may suffer from competing side reactions . Optimization of reaction time and catalyst loading is critical.
Q. How can computational modeling guide the design of adamantane monoacetate derivatives with tailored physicochemical properties?
- Thermodynamic data (e.g., ΔfH°gas = 34.4 kJ/mol for adamantane) and molecular dynamics simulations predict stability and reactivity. This aids in designing derivatives like 1,3-adamantanedicarboxylic acid or triazole-thiol analogs for enhanced bioavailability .
Q. Methodological Tables
Table 1. Comparison of Synthetic Routes
Table 2. Key Physicochemical Properties
Property | Value/Description | Reference |
---|---|---|
Purity (HPLC/GC) | ≥95% | |
Melting Point (related) | 136–138°C (1-Adamantaneacetic acid) | |
Storage Conditions | Dark, inert atmosphere, RT |
Eigenschaften
IUPAC Name |
(3-hydroxy-1-adamantyl) acetate | |
---|---|---|
Source | PubChem | |
URL | https://pubchem.ncbi.nlm.nih.gov | |
Description | Data deposited in or computed by PubChem | |
InChI |
InChI=1S/C12H18O3/c1-8(13)15-12-5-9-2-10(6-12)4-11(14,3-9)7-12/h9-10,14H,2-7H2,1H3 | |
Source | PubChem | |
URL | https://pubchem.ncbi.nlm.nih.gov | |
Description | Data deposited in or computed by PubChem | |
InChI Key |
HUJWKUYIFQXZEA-UHFFFAOYSA-N | |
Source | PubChem | |
URL | https://pubchem.ncbi.nlm.nih.gov | |
Description | Data deposited in or computed by PubChem | |
Canonical SMILES |
CC(=O)OC12CC3CC(C1)CC(C3)(C2)O | |
Source | PubChem | |
URL | https://pubchem.ncbi.nlm.nih.gov | |
Description | Data deposited in or computed by PubChem | |
Molecular Formula |
C12H18O3 | |
Source | PubChem | |
URL | https://pubchem.ncbi.nlm.nih.gov | |
Description | Data deposited in or computed by PubChem | |
Molecular Weight |
210.27 g/mol | |
Source | PubChem | |
URL | https://pubchem.ncbi.nlm.nih.gov | |
Description | Data deposited in or computed by PubChem | |
Haftungsausschluss und Informationen zu In-Vitro-Forschungsprodukten
Bitte beachten Sie, dass alle Artikel und Produktinformationen, die auf BenchChem präsentiert werden, ausschließlich zu Informationszwecken bestimmt sind. Die auf BenchChem zum Kauf angebotenen Produkte sind speziell für In-vitro-Studien konzipiert, die außerhalb lebender Organismen durchgeführt werden. In-vitro-Studien, abgeleitet von dem lateinischen Begriff "in Glas", beinhalten Experimente, die in kontrollierten Laborumgebungen unter Verwendung von Zellen oder Geweben durchgeführt werden. Es ist wichtig zu beachten, dass diese Produkte nicht als Arzneimittel oder Medikamente eingestuft sind und keine Zulassung der FDA für die Vorbeugung, Behandlung oder Heilung von medizinischen Zuständen, Beschwerden oder Krankheiten erhalten haben. Wir müssen betonen, dass jede Form der körperlichen Einführung dieser Produkte in Menschen oder Tiere gesetzlich strikt untersagt ist. Es ist unerlässlich, sich an diese Richtlinien zu halten, um die Einhaltung rechtlicher und ethischer Standards in Forschung und Experiment zu gewährleisten.