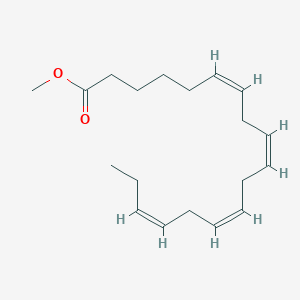
Methyl stearidonate
Übersicht
Beschreibung
Stearidonic acid methyl ester is a derivative of stearidonic acid, a polyunsaturated omega-3 fatty acid. It is known for its potential health benefits and is often used in dietary supplements and functional foods. Stearidonic acid is naturally found in certain plant oils, such as blackcurrant seed oil and echium oil, and is a precursor to eicosapentaenoic acid, an important fatty acid for human health .
Vorbereitungsmethoden
Synthetische Wege und Reaktionsbedingungen: Die Herstellung von Stearidon-Säuremethylester erfolgt in der Regel durch Veresterung von Stearidon-Säure mit Methanol. Dies kann durch säurekatalysierte Reaktionen mit Reagenzien wie Schwefelsäure oder Salzsäure erreicht werden. Die Reaktion wird in der Regel unter Rückflussbedingungen durchgeführt, um eine vollständige Umwandlung zu gewährleisten .
Industrielle Produktionsverfahren: Die industrielle Produktion von Stearidon-Säuremethylester beinhaltet häufig die Umesterung von Ölen, die reich an Stearidon-Säure sind, wie z. B. modifiziertes Sojaöl. Das Verfahren umfasst die Hydrolyse des Öls, um freie Fettsäuren freizusetzen, gefolgt von der Veresterung mit Methanol. Enzymatische Verfahren unter Verwendung von Lipasen können ebenfalls eingesetzt werden, um die Effizienz und Selektivität der Reaktion zu verbessern .
Analyse Chemischer Reaktionen
Arten von Reaktionen: Stearidon-Säuremethylester durchläuft verschiedene chemische Reaktionen, darunter:
Oxidation: Es kann oxidiert werden, um Hydroperoxide und andere Oxidationsprodukte zu bilden.
Reduktion: Es kann reduziert werden, um Stearidon-Säure zu bilden.
Veresterung und Umesterung: Es kann an Veresterungs- und Umesterungsreaktionen teilnehmen, um andere Ester zu bilden.
Häufige Reagenzien und Bedingungen:
Oxidation: Häufige Oxidationsmittel sind Sauerstoff und Peroxide.
Reduktion: Es können Reduktionsmittel wie Wasserstoffgas in Gegenwart eines Katalysators verwendet werden.
Veresterung: Säurekatalysatoren wie Schwefelsäure oder enzymatische Katalysatoren wie Lipasen werden häufig verwendet.
Hauptprodukte:
Oxidation: Hydroperoxide und andere Oxidationsprodukte.
Reduktion: Stearidon-Säure.
Veresterung: Verschiedene Ester, abhängig von dem verwendeten Alkohol.
Wissenschaftliche Forschungsanwendungen
Nutritional and Health Applications
Dietary Supplements and Functional Foods
Methyl stearidonate is recognized for its potential health benefits, particularly in dietary supplements. It serves as a precursor to eicosapentaenoic acid (EPA), an essential omega-3 fatty acid beneficial for cardiovascular health. Its incorporation into functional foods aims to enhance the nutritional profile, particularly in products targeting heart health and inflammation reduction .
Anti-inflammatory Properties
Research indicates that this compound exhibits anti-inflammatory effects by inhibiting the 5-lipoxygenase pathway and reducing tumor necrosis factor (TNF) and prostaglandin E2 levels. These properties suggest its potential in managing inflammatory diseases such as arthritis and cardiovascular conditions .
Pharmaceutical Applications
Drug Development
this compound is investigated for its role in drug formulation due to its biocompatibility and ability to enhance the solubility of active pharmaceutical ingredients (APIs). Its use as a nonionic surfactant aids in stabilizing emulsions and improving drug delivery systems .
Cancer Research
Studies have shown that this compound may possess anti-cancer properties, potentially modulating cell signaling pathways involved in cancer progression. This makes it a candidate for further research in oncology, particularly regarding its effects on tumor growth inhibition .
Industrial Applications
Cosmetics and Personal Care Products
In the cosmetics industry, this compound is utilized for its emollient properties. It enhances the texture and feel of products while providing moisturizing benefits. Its role as a stabilizer in emulsions is crucial for formulating creams and lotions .
Agricultural Sciences
The compound's amphiphilic nature allows it to be used in pesticide formulations, improving the efficacy of active ingredients through better dispersion and adherence to plant surfaces. Additionally, it has potential applications in soil remediation strategies .
Biochemical Research
Lipidomic Studies
this compound serves as a valuable tool in lipidomics, facilitating studies on lipid metabolism and cellular processes. Its incorporation into experimental models helps elucidate the roles of polyunsaturated fatty acids in cellular functions .
Microbial Metabolism Studies
Recent findings suggest that certain gut bacteria can metabolize stearidonic acid into conjugated forms, indicating a previously unexplored metabolic pathway that could have implications for human health and nutrition .
Data Table: Summary of Applications
Application Area | Description | Key Benefits |
---|---|---|
Nutritional Supplements | Precursor to EPA, enhances heart health | Anti-inflammatory effects |
Pharmaceutical | Enhances solubility of APIs, stabilizes drug formulations | Improved drug delivery |
Cosmetics | Used as an emollient and stabilizer in creams and lotions | Enhanced texture and moisturizing properties |
Agriculture | Improves pesticide formulations and soil remediation strategies | Increased efficacy of active ingredients |
Biochemical Research | Aids in lipid metabolism studies | Insights into cellular lipid functions |
Case Studies
- Anti-inflammatory Effects Study : A study demonstrated that this compound significantly reduced inflammation markers in animal models of arthritis, suggesting its potential therapeutic role in inflammatory diseases.
- Cancer Cell Line Research : Research involving various cancer cell lines showed that this compound inhibited proliferation, indicating its potential use as an adjunct therapy in cancer treatment.
- Lipidomic Analysis : A lipidomic study utilizing this compound revealed critical insights into membrane fluidity changes associated with different dietary fats, contributing to the understanding of dietary impacts on health.
Wirkmechanismus
Stearidonic acid methyl ester exerts its effects primarily through its conversion to eicosapentaenoic acid in the body. This conversion involves the enzyme delta-6-desaturase, which removes hydrogen atoms from stearidonic acid. Eicosapentaenoic acid is known to modulate inflammatory pathways and reduce the production of pro-inflammatory eicosanoids .
Vergleich Mit ähnlichen Verbindungen
Alpha-linolenic acid: Another omega-3 fatty acid that is a precursor to stearidonic acid.
Eicosapentaenoic acid: A direct product of stearidonic acid metabolism with similar health benefits.
Docosahexaenoic acid: Another omega-3 fatty acid with significant health benefits.
Uniqueness: Stearidonic acid methyl ester is unique due to its intermediate role in the metabolic pathway from alpha-linolenic acid to eicosapentaenoic acid. It is less prone to oxidation compared to eicosapentaenoic acid and docosahexaenoic acid, making it more stable for use in dietary supplements .
Biologische Aktivität
Methyl stearidonate, a secondary metabolite derived from marine sources such as the seaweed Padina tenuis, has garnered attention for its potential biological activities. This compound is structurally related to stearidonic acid, an omega-3 fatty acid, and has been studied for various health-related applications, including its effects on metabolic processes and antimicrobial properties.
Antimicrobial Properties
Research indicates that this compound exhibits significant antimicrobial activity. A study highlighted the isolation of active compounds from Enteromorpha linza, which included stearidonic acid and gamma-linolenic acid, demonstrating potent effects against pathogenic bacteria such as Prevotella intermedia and Porphyromonas gingivalis. The minimum inhibitory concentration (MIC) values for these compounds were determined to be 39.06 μg/mL against P. intermedia and 9.76 μg/mL against P. gingivalis .
Effects on Fatty Acid Metabolism
This compound plays a role in regulating fatty acid metabolism, particularly in the context of cancer prevention. A study involving Apc-mutant mice showed that a methyl donor depleted (MDD) diet significantly altered systemic fatty acid profiles, decreasing levels of cancer-promoting fatty acids while increasing beneficial ones like eicosapentaenoic acid (EPA). This suggests that this compound may contribute to protective mechanisms against colorectal cancer .
Antioxidant Activity
The antioxidant capacity of this compound has also been explored. Various extracts containing this compound have been evaluated for their ability to scavenge free radicals and reduce oxidative stress. For instance, studies on seaweed extracts have demonstrated their high ferric-reducing antioxidant power (FRAP), indicating that this compound could play a role in enhancing antioxidant defenses in biological systems .
Table: Biological Activities of this compound
Activity | Effect | Reference |
---|---|---|
Antimicrobial | Effective against P. intermedia and P. gingivalis | |
Cancer Prevention | Alters fatty acid metabolism favorably | |
Antioxidant | High FRAP values in seaweed extracts |
Case Study: Methyl Donor Depletion and Cancer
In a controlled study involving Apc-mutant mice, researchers observed that those subjected to a methyl donor depleted diet exhibited significant reductions in tumor development compared to controls. The study highlighted the importance of dietary components, including this compound, in modulating metabolic pathways associated with cancer risk .
The biological activities of this compound can be attributed to several mechanisms:
- Antimicrobial Action : The compound disrupts bacterial cell membranes or interferes with metabolic pathways critical for bacterial survival.
- Fatty Acid Regulation : By modifying the levels of various fatty acids in the body, this compound may influence inflammation and tumorigenesis.
- Antioxidant Defense : It may enhance cellular antioxidant capacity, thereby protecting cells from oxidative damage.
Eigenschaften
IUPAC Name |
methyl (6Z,9Z,12Z,15Z)-octadeca-6,9,12,15-tetraenoate | |
---|---|---|
Source | PubChem | |
URL | https://pubchem.ncbi.nlm.nih.gov | |
Description | Data deposited in or computed by PubChem | |
InChI |
InChI=1S/C19H30O2/c1-3-4-5-6-7-8-9-10-11-12-13-14-15-16-17-18-19(20)21-2/h4-5,7-8,10-11,13-14H,3,6,9,12,15-18H2,1-2H3/b5-4-,8-7-,11-10-,14-13- | |
Source | PubChem | |
URL | https://pubchem.ncbi.nlm.nih.gov | |
Description | Data deposited in or computed by PubChem | |
InChI Key |
BIRKCHKCDPCDEG-GJDCDIHCSA-N | |
Source | PubChem | |
URL | https://pubchem.ncbi.nlm.nih.gov | |
Description | Data deposited in or computed by PubChem | |
Canonical SMILES |
CCC=CCC=CCC=CCC=CCCCCC(=O)OC | |
Source | PubChem | |
URL | https://pubchem.ncbi.nlm.nih.gov | |
Description | Data deposited in or computed by PubChem | |
Isomeric SMILES |
CC/C=C\C/C=C\C/C=C\C/C=C\CCCCC(=O)OC | |
Source | PubChem | |
URL | https://pubchem.ncbi.nlm.nih.gov | |
Description | Data deposited in or computed by PubChem | |
Molecular Formula |
C19H30O2 | |
Source | PubChem | |
URL | https://pubchem.ncbi.nlm.nih.gov | |
Description | Data deposited in or computed by PubChem | |
DSSTOX Substance ID |
DTXSID801015995 | |
Record name | Methyl stearidonate | |
Source | EPA DSSTox | |
URL | https://comptox.epa.gov/dashboard/DTXSID801015995 | |
Description | DSSTox provides a high quality public chemistry resource for supporting improved predictive toxicology. | |
Molecular Weight |
290.4 g/mol | |
Source | PubChem | |
URL | https://pubchem.ncbi.nlm.nih.gov | |
Description | Data deposited in or computed by PubChem | |
CAS No. |
73097-00-4 | |
Record name | Methyl stearidonate | |
Source | EPA DSSTox | |
URL | https://comptox.epa.gov/dashboard/DTXSID801015995 | |
Description | DSSTox provides a high quality public chemistry resource for supporting improved predictive toxicology. | |
Retrosynthesis Analysis
AI-Powered Synthesis Planning: Our tool employs the Template_relevance Pistachio, Template_relevance Bkms_metabolic, Template_relevance Pistachio_ringbreaker, Template_relevance Reaxys, Template_relevance Reaxys_biocatalysis model, leveraging a vast database of chemical reactions to predict feasible synthetic routes.
One-Step Synthesis Focus: Specifically designed for one-step synthesis, it provides concise and direct routes for your target compounds, streamlining the synthesis process.
Accurate Predictions: Utilizing the extensive PISTACHIO, BKMS_METABOLIC, PISTACHIO_RINGBREAKER, REAXYS, REAXYS_BIOCATALYSIS database, our tool offers high-accuracy predictions, reflecting the latest in chemical research and data.
Strategy Settings
Precursor scoring | Relevance Heuristic |
---|---|
Min. plausibility | 0.01 |
Model | Template_relevance |
Template Set | Pistachio/Bkms_metabolic/Pistachio_ringbreaker/Reaxys/Reaxys_biocatalysis |
Top-N result to add to graph | 6 |
Feasible Synthetic Routes
Haftungsausschluss und Informationen zu In-Vitro-Forschungsprodukten
Bitte beachten Sie, dass alle Artikel und Produktinformationen, die auf BenchChem präsentiert werden, ausschließlich zu Informationszwecken bestimmt sind. Die auf BenchChem zum Kauf angebotenen Produkte sind speziell für In-vitro-Studien konzipiert, die außerhalb lebender Organismen durchgeführt werden. In-vitro-Studien, abgeleitet von dem lateinischen Begriff "in Glas", beinhalten Experimente, die in kontrollierten Laborumgebungen unter Verwendung von Zellen oder Geweben durchgeführt werden. Es ist wichtig zu beachten, dass diese Produkte nicht als Arzneimittel oder Medikamente eingestuft sind und keine Zulassung der FDA für die Vorbeugung, Behandlung oder Heilung von medizinischen Zuständen, Beschwerden oder Krankheiten erhalten haben. Wir müssen betonen, dass jede Form der körperlichen Einführung dieser Produkte in Menschen oder Tiere gesetzlich strikt untersagt ist. Es ist unerlässlich, sich an diese Richtlinien zu halten, um die Einhaltung rechtlicher und ethischer Standards in Forschung und Experiment zu gewährleisten.