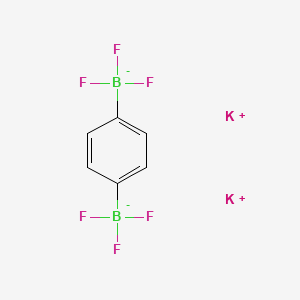
Dikalium-1,4-phenylenbis(trifluoroborat)
Übersicht
Beschreibung
Synthesis Analysis
The synthesis of Dipotassium phenylene-1,4-bistrifluoroborate involves a combination of crystallography, DFT calculations, topological and non-covalent interaction analysis . The structure of this aromatic bis(trifluoroborate) dipotassium salt is elucidated by these methods .Molecular Structure Analysis
The molecular structure of Dipotassium phenylene-1,4-bistrifluoroborate is characterized by a 3D network undergoing spontaneous self-assembly . This is due to the massive participation of weak intra- and intermolecular interactions for which fluorine atoms play a leading role .Chemical Reactions Analysis
Organotrifluoroborate salts, including Dipotassium phenylene-1,4-bistrifluoroborate, have been shown to be attractive and versatile boronic acid surrogates . They are used in many transition-metal-catalysed and also “metal-free” C–C bond-forming reactions .Physical and Chemical Properties Analysis
Dipotassium phenylene-1,4-bistrifluoroborate is known for its exceptional stability towards oxygen and common reagents . It also has higher nucleophilicity and excellent functional group tolerance .Wissenschaftliche Forschungsanwendungen
Organische Synthese
Diese Verbindung dient als wertvolles Reagenz in der organischen Synthese. Ihre Stabilität und höhere Nucleophilie machen sie zu einem hervorragenden Kandidaten für verschiedene organische Reaktionen, einschließlich solcher, die C–C-Bindungen ohne den Bedarf an Übergangsmetallen bilden .
Kristall-Engineering
Im Kristall-Engineering trägt Dikalium-1,4-phenylenbis(trifluoroborat) zur Bildung von 3D-Netzwerken durch Selbstassemblierung bei. Dies wird durch schwache intra- und intermolekulare Wechselwirkungen erleichtert, bei denen Fluoratome eine bedeutende Rolle spielen .
Asymmetrische Synthese
Die Verbindung wird in der asymmetrischen Synthese verwendet, um komplexe organische Gerüste zu konstruieren. Sie wirkt als vielseitiger Boronsäure-Ersatzstoff und beteiligt sich an stereoselektiven Additionsreaktionen .
Design von funktionalen Materialien
Ihre Rolle im systematischen Design von fortschrittlichen funktionalen Materialien ist mit ihrer Fähigkeit verbunden, supramolekulare Strukturen zu bilden. Diese Strukturen sind entscheidend für die Entwicklung neuer Materialien mit spezifischen Eigenschaften .
Supramolekulare Chemie
Die Fähigkeit von Dikalium-1,4-phenylenbis(trifluoroborat), sich spontan in einem 3D-Netzwerk selbst zu assemblieren, macht sie zu einem interessanten Thema in der supramolekularen Chemie. Das Studium dieser Netzwerke kann zu Erkenntnissen über die Entwicklung neuer supramolekularer Systeme führen .
Wirkmechanismus
Target of Action
Dipotassium phenylene-1,4-bistrifluoroborate is a type of organotrifluoroborate salt . Organotrifluoroborate salts have been shown to be attractive and versatile boronic acid surrogates . They are often used as reagents in many transition-metal-catalysed and metal-free C–C bond-forming reactions . Therefore, the primary targets of Dipotassium phenylene-1,4-bistrifluoroborate are the reactants in these chemical reactions.
Mode of Action
The compound interacts with its targets through chemical reactions. It is known for its exceptional stability towards oxygen and common reagents, higher nucleophilicity, and excellent functional group tolerance . These properties make it a valuable reagent in a variety of chemical scenarios, including palladium-catalysed cross-coupling reactions and stereoselective rhodium-catalysed 1,2- and 1,4-addition reactions .
Biochemical Pathways
The exact biochemical pathways affected by Dipotassium phenylene-1,4-bistrifluoroborate are dependent on the specific reactions it is involved in. As a versatile boronic acid surrogate, it can participate in a wide range of reactions, leading to the construction of complex organic frameworks .
Result of Action
The result of Dipotassium phenylene-1,4-bistrifluoroborate’s action is the formation of new chemical bonds and structures. It contributes to the formation of complex organic frameworks through its participation in various chemical reactions .
Action Environment
The action of Dipotassium phenylene-1,4-bistrifluoroborate can be influenced by various environmental factors. For instance, its stability and reactivity can be affected by the presence of oxygen and other common reagents . Additionally, the compound forms a 3D network undergoing spontaneous self-assembly thanks to the massive participation of weak intra- and intermolecular interactions .
Safety and Hazards
Zukünftige Richtungen
The study of non-covalent interactions, particularly the contribution of organic fluorine in the formation of different supramolecular motifs, is an expanding area of research . This has gained much interest in crystal engineering for the systematic design of advanced functional materials and in asymmetric synthesis .
Biochemische Analyse
Biochemical Properties
Dipotassium phenylene-1,4-bistrifluoroborate plays a crucial role in biochemical reactions, particularly in transition-metal-catalyzed and metal-free carbon-carbon bond-forming reactions . It interacts with various enzymes, proteins, and other biomolecules, facilitating the formation of complex organic frameworks. The compound’s interactions are primarily driven by its high nucleophilicity and stability towards oxygen and common reagents . These properties enable it to participate in stereoselective rhodium-catalyzed addition reactions and palladium-catalyzed cross-coupling reactions .
Cellular Effects
Dipotassium phenylene-1,4-bistrifluoroborate influences various cellular processes, including cell signaling pathways, gene expression, and cellular metabolism. Its impact on cell function is mediated through its interactions with specific enzymes and proteins involved in these pathways . For instance, the compound can modulate the activity of enzymes responsible for key metabolic processes, thereby affecting cellular metabolism and energy production . Additionally, it has been observed to influence gene expression by interacting with transcription factors and other regulatory proteins .
Molecular Mechanism
The molecular mechanism of dipotassium phenylene-1,4-bistrifluoroborate involves its binding interactions with biomolecules, enzyme inhibition or activation, and changes in gene expression . The compound’s high nucleophilicity allows it to form stable complexes with various enzymes and proteins, thereby modulating their activity . It can act as an inhibitor or activator of specific enzymes, depending on the nature of the interaction . Furthermore, its interactions with transcription factors and other regulatory proteins can lead to changes in gene expression, ultimately affecting cellular function .
Temporal Effects in Laboratory Settings
In laboratory settings, the effects of dipotassium phenylene-1,4-bistrifluoroborate can change over time due to its stability and degradation properties . The compound is known for its exceptional stability towards oxygen and common reagents, which allows it to maintain its activity over extended periods . Prolonged exposure to certain conditions may lead to its degradation, resulting in a decrease in its effectiveness . Long-term studies have shown that the compound can have sustained effects on cellular function, particularly in in vitro and in vivo settings .
Dosage Effects in Animal Models
The effects of dipotassium phenylene-1,4-bistrifluoroborate vary with different dosages in animal models . At lower doses, the compound has been observed to facilitate various biochemical reactions without causing significant adverse effects . At higher doses, it may exhibit toxic or adverse effects, including enzyme inhibition and disruption of cellular processes . Threshold effects have been noted, where the compound’s activity significantly changes at specific dosage levels .
Metabolic Pathways
Dipotassium phenylene-1,4-bistrifluoroborate is involved in several metabolic pathways, interacting with various enzymes and cofactors . Its high nucleophilicity allows it to participate in key metabolic reactions, influencing metabolic flux and metabolite levels . The compound’s interactions with enzymes such as rhodium and palladium catalysts play a crucial role in its involvement in these pathways .
Transport and Distribution
Within cells and tissues, dipotassium phenylene-1,4-bistrifluoroborate is transported and distributed through interactions with specific transporters and binding proteins . These interactions facilitate its localization and accumulation in specific cellular compartments . The compound’s distribution is influenced by its stability and binding affinity to various biomolecules .
Subcellular Localization
Dipotassium phenylene-1,4-bistrifluoroborate exhibits specific subcellular localization, which affects its activity and function . The compound is directed to particular compartments or organelles through targeting signals and post-translational modifications . Its localization within the cell can influence its interactions with enzymes and proteins, thereby modulating its biochemical effects .
Eigenschaften
IUPAC Name |
dipotassium;trifluoro-(4-trifluoroboranuidylphenyl)boranuide | |
---|---|---|
Source | PubChem | |
URL | https://pubchem.ncbi.nlm.nih.gov | |
Description | Data deposited in or computed by PubChem | |
InChI |
InChI=1S/C6H4B2F6.2K/c9-7(10,11)5-1-2-6(4-3-5)8(12,13)14;;/h1-4H;;/q-2;2*+1 | |
Source | PubChem | |
URL | https://pubchem.ncbi.nlm.nih.gov | |
Description | Data deposited in or computed by PubChem | |
InChI Key |
SKFWLYVYQKXSTD-UHFFFAOYSA-N | |
Source | PubChem | |
URL | https://pubchem.ncbi.nlm.nih.gov | |
Description | Data deposited in or computed by PubChem | |
Canonical SMILES |
[B-](C1=CC=C(C=C1)[B-](F)(F)F)(F)(F)F.[K+].[K+] | |
Source | PubChem | |
URL | https://pubchem.ncbi.nlm.nih.gov | |
Description | Data deposited in or computed by PubChem | |
Molecular Formula |
C6H4B2F6K2 | |
Source | PubChem | |
URL | https://pubchem.ncbi.nlm.nih.gov | |
Description | Data deposited in or computed by PubChem | |
DSSTOX Substance ID |
DTXSID10712369 | |
Record name | dipotassium;trifluoro-(4-trifluoroboranuidylphenyl)boranuide | |
Source | EPA DSSTox | |
URL | https://comptox.epa.gov/dashboard/DTXSID10712369 | |
Description | DSSTox provides a high quality public chemistry resource for supporting improved predictive toxicology. | |
Molecular Weight |
289.91 g/mol | |
Source | PubChem | |
URL | https://pubchem.ncbi.nlm.nih.gov | |
Description | Data deposited in or computed by PubChem | |
CAS No. |
1150655-08-5 | |
Record name | dipotassium;trifluoro-(4-trifluoroboranuidylphenyl)boranuide | |
Source | EPA DSSTox | |
URL | https://comptox.epa.gov/dashboard/DTXSID10712369 | |
Description | DSSTox provides a high quality public chemistry resource for supporting improved predictive toxicology. | |
Retrosynthesis Analysis
AI-Powered Synthesis Planning: Our tool employs the Template_relevance Pistachio, Template_relevance Bkms_metabolic, Template_relevance Pistachio_ringbreaker, Template_relevance Reaxys, Template_relevance Reaxys_biocatalysis model, leveraging a vast database of chemical reactions to predict feasible synthetic routes.
One-Step Synthesis Focus: Specifically designed for one-step synthesis, it provides concise and direct routes for your target compounds, streamlining the synthesis process.
Accurate Predictions: Utilizing the extensive PISTACHIO, BKMS_METABOLIC, PISTACHIO_RINGBREAKER, REAXYS, REAXYS_BIOCATALYSIS database, our tool offers high-accuracy predictions, reflecting the latest in chemical research and data.
Strategy Settings
Precursor scoring | Relevance Heuristic |
---|---|
Min. plausibility | 0.01 |
Model | Template_relevance |
Template Set | Pistachio/Bkms_metabolic/Pistachio_ringbreaker/Reaxys/Reaxys_biocatalysis |
Top-N result to add to graph | 6 |
Feasible Synthetic Routes
Haftungsausschluss und Informationen zu In-Vitro-Forschungsprodukten
Bitte beachten Sie, dass alle Artikel und Produktinformationen, die auf BenchChem präsentiert werden, ausschließlich zu Informationszwecken bestimmt sind. Die auf BenchChem zum Kauf angebotenen Produkte sind speziell für In-vitro-Studien konzipiert, die außerhalb lebender Organismen durchgeführt werden. In-vitro-Studien, abgeleitet von dem lateinischen Begriff "in Glas", beinhalten Experimente, die in kontrollierten Laborumgebungen unter Verwendung von Zellen oder Geweben durchgeführt werden. Es ist wichtig zu beachten, dass diese Produkte nicht als Arzneimittel oder Medikamente eingestuft sind und keine Zulassung der FDA für die Vorbeugung, Behandlung oder Heilung von medizinischen Zuständen, Beschwerden oder Krankheiten erhalten haben. Wir müssen betonen, dass jede Form der körperlichen Einführung dieser Produkte in Menschen oder Tiere gesetzlich strikt untersagt ist. Es ist unerlässlich, sich an diese Richtlinien zu halten, um die Einhaltung rechtlicher und ethischer Standards in Forschung und Experiment zu gewährleisten.