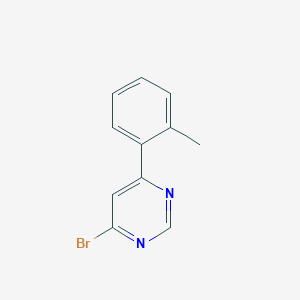
4-Bromo-6-(o-tolyl)pyrimidine
Übersicht
Beschreibung
“4-Bromo-6-(o-tolyl)pyrimidine” is a chemical compound with the molecular formula C11H9BrN2 and a molecular weight of 249.11 . It is a derivative of pyrimidine, a six-membered heterocyclic aromatic compound that is widely found in nature .
Molecular Structure Analysis
The molecular structure of “4-Bromo-6-(o-tolyl)pyrimidine” consists of a pyrimidine ring, which is a six-membered heterocyclic aromatic compound with two nitrogen atoms, substituted with a bromine atom and an o-tolyl group .Wissenschaftliche Forschungsanwendungen
Therapeutic Potential
Pyrimidine and its derivatives have been proven to have a wide range of biological potential . They have been described with various therapeutic potentials such as anticancer , antiviral , antimicrobial , anti-inflammatory , analgesic , antioxidant , and antimalarial activities.
Antimicrobial Activity
Pyrimidine derivatives have shown promising antimicrobial activity. The extensive use of antibiotics has led to the appearance of multidrug resistant microbial pathogens which necessitated the search for new chemical entities for treatment of microbial infections .
Neuroprotective Agents
Pyrimidine and its derivatives have been proven to have neuroprotective properties . They have been used in the treatment of various neurodegenerative diseases, such as Alzheimer’s disease, Huntington’s disease, Parkinson’s disease, Amyotrophic lateral sclerosis, ischemic stroke, and traumatic brain injury .
Anti-neuroinflammatory Agents
Triazole-pyrimidine hybrid compounds have shown promising anti-neuroinflammatory properties . They have been found to inhibit nitric oxide (NO) and tumor necrosis factor-α (TNF-α) production in LPS-stimulated human microglia cells .
Anticancer Agents
Pyrimidine derivatives have been used as anticancer agents . They have been found to be effective against a wide range of cancers.
Antioxidant Agents
Pyrimidine derivatives have been found to have antioxidant properties . They have been used to neutralize harmful free radicals in the body.
Zukünftige Richtungen
Pyrimidine derivatives, including “4-Bromo-6-(o-tolyl)pyrimidine”, have potential therapeutic applications. For instance, they have been studied for their neuroprotective and anti-neuroinflammatory properties . Future research could focus on further exploring these properties and developing novel therapeutic agents based on the pyrimidine scaffold .
Eigenschaften
IUPAC Name |
4-bromo-6-(2-methylphenyl)pyrimidine | |
---|---|---|
Source | PubChem | |
URL | https://pubchem.ncbi.nlm.nih.gov | |
Description | Data deposited in or computed by PubChem | |
InChI |
InChI=1S/C11H9BrN2/c1-8-4-2-3-5-9(8)10-6-11(12)14-7-13-10/h2-7H,1H3 | |
Source | PubChem | |
URL | https://pubchem.ncbi.nlm.nih.gov | |
Description | Data deposited in or computed by PubChem | |
InChI Key |
VDBVWWOWMWJGAY-UHFFFAOYSA-N | |
Source | PubChem | |
URL | https://pubchem.ncbi.nlm.nih.gov | |
Description | Data deposited in or computed by PubChem | |
Canonical SMILES |
CC1=CC=CC=C1C2=CC(=NC=N2)Br | |
Source | PubChem | |
URL | https://pubchem.ncbi.nlm.nih.gov | |
Description | Data deposited in or computed by PubChem | |
Molecular Formula |
C11H9BrN2 | |
Source | PubChem | |
URL | https://pubchem.ncbi.nlm.nih.gov | |
Description | Data deposited in or computed by PubChem | |
Molecular Weight |
249.11 g/mol | |
Source | PubChem | |
URL | https://pubchem.ncbi.nlm.nih.gov | |
Description | Data deposited in or computed by PubChem | |
Product Name |
4-Bromo-6-(o-tolyl)pyrimidine |
Retrosynthesis Analysis
AI-Powered Synthesis Planning: Our tool employs the Template_relevance Pistachio, Template_relevance Bkms_metabolic, Template_relevance Pistachio_ringbreaker, Template_relevance Reaxys, Template_relevance Reaxys_biocatalysis model, leveraging a vast database of chemical reactions to predict feasible synthetic routes.
One-Step Synthesis Focus: Specifically designed for one-step synthesis, it provides concise and direct routes for your target compounds, streamlining the synthesis process.
Accurate Predictions: Utilizing the extensive PISTACHIO, BKMS_METABOLIC, PISTACHIO_RINGBREAKER, REAXYS, REAXYS_BIOCATALYSIS database, our tool offers high-accuracy predictions, reflecting the latest in chemical research and data.
Strategy Settings
Precursor scoring | Relevance Heuristic |
---|---|
Min. plausibility | 0.01 |
Model | Template_relevance |
Template Set | Pistachio/Bkms_metabolic/Pistachio_ringbreaker/Reaxys/Reaxys_biocatalysis |
Top-N result to add to graph | 6 |
Feasible Synthetic Routes
Haftungsausschluss und Informationen zu In-Vitro-Forschungsprodukten
Bitte beachten Sie, dass alle Artikel und Produktinformationen, die auf BenchChem präsentiert werden, ausschließlich zu Informationszwecken bestimmt sind. Die auf BenchChem zum Kauf angebotenen Produkte sind speziell für In-vitro-Studien konzipiert, die außerhalb lebender Organismen durchgeführt werden. In-vitro-Studien, abgeleitet von dem lateinischen Begriff "in Glas", beinhalten Experimente, die in kontrollierten Laborumgebungen unter Verwendung von Zellen oder Geweben durchgeführt werden. Es ist wichtig zu beachten, dass diese Produkte nicht als Arzneimittel oder Medikamente eingestuft sind und keine Zulassung der FDA für die Vorbeugung, Behandlung oder Heilung von medizinischen Zuständen, Beschwerden oder Krankheiten erhalten haben. Wir müssen betonen, dass jede Form der körperlichen Einführung dieser Produkte in Menschen oder Tiere gesetzlich strikt untersagt ist. Es ist unerlässlich, sich an diese Richtlinien zu halten, um die Einhaltung rechtlicher und ethischer Standards in Forschung und Experiment zu gewährleisten.