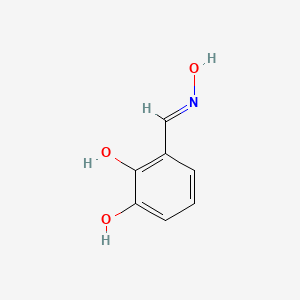
2,3-Dihydroxybenzaldehyde oxime
Übersicht
Beschreibung
2,3-Dihydroxybenzaldehyde oxime is an organic compound with the molecular formula C7H7NO3. It is a derivative of benzaldehyde, where the aldehyde group is converted to an oxime group, and two hydroxyl groups are attached to the benzene ring at the 2 and 3 positions.
Wirkmechanismus
Target of Action
It is known that the compound is used in the synthesis of hydrazones , which are known to interact with various biological targets.
Mode of Action
The mode of action of 2,3-Dihydroxybenzaldehyde oxime involves its reaction with suitable aldehydes to form hydrazones . This reaction is essentially irreversible as the adduct dehydrates .
Biochemical Pathways
It is known that the compound is involved in the synthesis of hydrazones , which can interact with various biochemical pathways depending on their structure and the nature of their targets.
Result of Action
It is known that the compound is involved in the synthesis of hydrazones , which can have various effects depending on their structure and the nature of their targets.
Biochemische Analyse
Biochemical Properties
2,3-Dihydroxybenzaldehyde oxime plays a significant role in biochemical reactions, particularly in the formation of oximes and hydrazones. It interacts with enzymes such as cytochrome P450, which catalyzes the hydroxylation of aromatic compounds. Additionally, this compound can form complexes with metal ions, influencing their biochemical activity. These interactions are crucial for understanding the compound’s role in redox reactions and its potential as a chemosensitizing agent .
Cellular Effects
This compound affects various types of cells and cellular processes. It has been shown to disrupt cellular antioxidation systems, leading to oxidative stress in fungal cells. This disruption can inhibit fungal growth and enhance the efficacy of conventional antifungal agents
Molecular Mechanism
The molecular mechanism of this compound involves its interaction with biomolecules through the formation of oximes. The compound reacts with aldehydes and ketones to form stable oxime derivatives, which can then undergo further biochemical transformations. This reaction is catalyzed by enzymes such as hydroxylamine oxidase, which facilitates the conversion of hydroxylamine to oxime . Additionally, this compound can inhibit or activate specific enzymes, leading to changes in gene expression and cellular function.
Temporal Effects in Laboratory Settings
In laboratory settings, the effects of this compound can change over time. The compound’s stability and degradation are influenced by factors such as pH, temperature, and the presence of other reactive species. Long-term studies have shown that this compound can maintain its activity for extended periods under controlled conditions . Its degradation products may also have biological activity, necessitating careful monitoring in experimental setups.
Dosage Effects in Animal Models
The effects of this compound vary with different dosages in animal models. At low doses, the compound may exhibit beneficial effects, such as enhancing the efficacy of antifungal agents. At high doses, this compound can induce toxic effects, including oxidative stress and cellular damage . Threshold effects have been observed, indicating that careful dosage optimization is essential for achieving desired outcomes without adverse effects.
Metabolic Pathways
This compound is involved in various metabolic pathways, including the biosynthesis of phenolic compounds. It interacts with enzymes such as 2,3-dihydro-2,3-dihydroxybenzoate synthase and 2,3-dihydro-2,3-dihydroxybenzoate dehydrogenase, which are involved in the production of dihydroxybenzoates . These interactions can affect metabolic flux and the levels of metabolites, influencing overall cellular metabolism.
Transport and Distribution
Within cells and tissues, this compound is transported and distributed through interactions with transporters and binding proteins. These interactions can affect the compound’s localization and accumulation, influencing its biological activity . Understanding the transport mechanisms is crucial for optimizing the compound’s delivery and efficacy in therapeutic applications.
Subcellular Localization
The subcellular localization of this compound is determined by targeting signals and post-translational modifications. The compound may be directed to specific compartments or organelles, where it exerts its biochemical effects. For example, this compound may localize to the mitochondria, influencing mitochondrial function and cellular energy metabolism
Vorbereitungsmethoden
Synthetic Routes and Reaction Conditions: 2,3-Dihydroxybenzaldehyde oxime can be synthesized through the reaction of 2,3-dihydroxybenzaldehyde with hydroxylamine hydrochloride. The reaction typically occurs in an aqueous or alcoholic medium, often under reflux conditions. The general reaction is as follows:
2,3-Dihydroxybenzaldehyde+Hydroxylamine Hydrochloride→2,3-Dihydroxybenzaldehyde Oxime+Water+Hydrochloric Acid
The reaction is facilitated by the presence of a base, such as sodium acetate, which neutralizes the hydrochloric acid formed during the reaction .
Industrial Production Methods: In industrial settings, the synthesis of this compound may involve solvent-free methods to minimize waste and environmental impact. One such method involves grinding the reactants together in the presence of a catalyst like bismuth oxide (Bi2O3), which promotes the formation of the oxime without the need for solvents .
Analyse Chemischer Reaktionen
Types of Reactions: 2,3-Dihydroxybenzaldehyde oxime undergoes various chemical reactions, including:
Oxidation: The oxime group can be oxidized to form nitrile compounds.
Reduction: The oxime group can be reduced to form amines.
Substitution: The hydroxyl groups can participate in substitution reactions to form ethers or esters.
Common Reagents and Conditions:
Oxidation: Common oxidizing agents include potassium permanganate (KMnO4) and chromium trioxide (CrO3).
Reduction: Reducing agents such as sodium borohydride (NaBH4) or lithium aluminum hydride (LiAlH4) are used.
Substitution: Reagents like alkyl halides or acyl chlorides are used in the presence of a base to facilitate substitution reactions.
Major Products:
Oxidation: Formation of 2,3-dihydroxybenzonitrile.
Reduction: Formation of 2,3-dihydroxybenzylamine.
Substitution: Formation of 2,3-dihydroxybenzyl ethers or esters.
Wissenschaftliche Forschungsanwendungen
2,3-Dihydroxybenzaldehyde oxime has several applications in scientific research:
Medicinal Chemistry: It is used as an intermediate in the synthesis of pharmaceuticals, particularly those targeting neurological conditions.
Materials Science: The compound is used in the development of novel materials with specific electronic or optical properties.
Industry: It is used in the synthesis of dyes, pigments, and other specialty chemicals.
Vergleich Mit ähnlichen Verbindungen
2,4-Dihydroxybenzaldehyde: Similar structure but with hydroxyl groups at the 2 and 4 positions.
2,5-Dihydroxybenzaldehyde: Hydroxyl groups at the 2 and 5 positions.
3,4-Dihydroxybenzaldehyde: Hydroxyl groups at the 3 and 4 positions.
Uniqueness: 2,3-Dihydroxybenzaldehyde oxime is unique due to the specific positioning of the hydroxyl groups and the oxime group, which confer distinct chemical reactivity and biological activity. This unique structure allows for specific interactions in both chemical and biological systems, making it a valuable compound in various applications.
Eigenschaften
IUPAC Name |
3-[(E)-hydroxyiminomethyl]benzene-1,2-diol | |
---|---|---|
Source | PubChem | |
URL | https://pubchem.ncbi.nlm.nih.gov | |
Description | Data deposited in or computed by PubChem | |
InChI |
InChI=1S/C7H7NO3/c9-6-3-1-2-5(4-8-11)7(6)10/h1-4,9-11H/b8-4+ | |
Source | PubChem | |
URL | https://pubchem.ncbi.nlm.nih.gov | |
Description | Data deposited in or computed by PubChem | |
InChI Key |
UAICVXLIXRIZBA-XBXARRHUSA-N | |
Source | PubChem | |
URL | https://pubchem.ncbi.nlm.nih.gov | |
Description | Data deposited in or computed by PubChem | |
Canonical SMILES |
C1=CC(=C(C(=C1)O)O)C=NO | |
Source | PubChem | |
URL | https://pubchem.ncbi.nlm.nih.gov | |
Description | Data deposited in or computed by PubChem | |
Isomeric SMILES |
C1=CC(=C(C(=C1)O)O)/C=N/O | |
Source | PubChem | |
URL | https://pubchem.ncbi.nlm.nih.gov | |
Description | Data deposited in or computed by PubChem | |
Molecular Formula |
C7H7NO3 | |
Source | PubChem | |
URL | https://pubchem.ncbi.nlm.nih.gov | |
Description | Data deposited in or computed by PubChem | |
Molecular Weight |
153.14 g/mol | |
Source | PubChem | |
URL | https://pubchem.ncbi.nlm.nih.gov | |
Description | Data deposited in or computed by PubChem | |
Retrosynthesis Analysis
AI-Powered Synthesis Planning: Our tool employs the Template_relevance Pistachio, Template_relevance Bkms_metabolic, Template_relevance Pistachio_ringbreaker, Template_relevance Reaxys, Template_relevance Reaxys_biocatalysis model, leveraging a vast database of chemical reactions to predict feasible synthetic routes.
One-Step Synthesis Focus: Specifically designed for one-step synthesis, it provides concise and direct routes for your target compounds, streamlining the synthesis process.
Accurate Predictions: Utilizing the extensive PISTACHIO, BKMS_METABOLIC, PISTACHIO_RINGBREAKER, REAXYS, REAXYS_BIOCATALYSIS database, our tool offers high-accuracy predictions, reflecting the latest in chemical research and data.
Strategy Settings
Precursor scoring | Relevance Heuristic |
---|---|
Min. plausibility | 0.01 |
Model | Template_relevance |
Template Set | Pistachio/Bkms_metabolic/Pistachio_ringbreaker/Reaxys/Reaxys_biocatalysis |
Top-N result to add to graph | 6 |
Feasible Synthetic Routes
Q1: How does 2,3-Dihydroxybenzaldehyde oxime interact with metal ions, and what are the structural consequences?
A1: this compound acts as a strong chelating ligand, particularly towards Group IV metals like titanium, zirconium, and hafnium. It achieves this through its catechol and oxime groups, both capable of binding to metal ions. This interaction leads to the formation of unique polynuclear oxo-clusters with intriguing structural motifs. For instance, H3dihybo facilitates the formation of hexanuclear clusters with a [MIV6(μ3-Ο)2(μ-Ο)3] core, where M represents the metal ion. Interestingly, in the case of zirconium and hafnium, these clusters adopt a rare trigonal-prismatic arrangement of the metal atoms, distinct from the typical octahedral arrangement observed in previously reported Zr6 clusters [, ].
Q2: What makes this compound particularly interesting for photocatalytic applications?
A2: The combination of the catechol and oxime groups in H3dihybo significantly impacts the electronic properties of the resulting metal oxide clusters. This ligand effectively reduces the band gap of metal oxides like TiO2 and ZrO2. For example, titanium and zirconium compounds complexed with H3dihybo exhibited band gaps of 1.48 eV and 2.34 eV, respectively []. This reduction in band gap compared to the pure metal oxides is crucial for enhancing visible light absorption, thereby increasing their potential for applications in photocatalysis, such as water splitting and degradation of pollutants [, ].
Q3: Can computational chemistry provide insights into the properties of metal complexes formed with this compound?
A3: Yes, Density Functional Theory (DFT) calculations have been instrumental in understanding the properties of metal complexes containing H3dihybo. These calculations revealed a fascinating phenomenon: the triangular M3 (M = Ti, Zr) metallic ring cores within these clusters exhibit pronounced metalloaromaticity []. This means that the metal rings possess electron delocalization, similar to aromatic organic compounds like benzene. The extent of metalloaromaticity varies depending on the metal center, with zirconium complexes showing a higher degree compared to titanium complexes []. This finding opens up possibilities for exploring new materials with unique electronic and magnetic properties.
Haftungsausschluss und Informationen zu In-Vitro-Forschungsprodukten
Bitte beachten Sie, dass alle Artikel und Produktinformationen, die auf BenchChem präsentiert werden, ausschließlich zu Informationszwecken bestimmt sind. Die auf BenchChem zum Kauf angebotenen Produkte sind speziell für In-vitro-Studien konzipiert, die außerhalb lebender Organismen durchgeführt werden. In-vitro-Studien, abgeleitet von dem lateinischen Begriff "in Glas", beinhalten Experimente, die in kontrollierten Laborumgebungen unter Verwendung von Zellen oder Geweben durchgeführt werden. Es ist wichtig zu beachten, dass diese Produkte nicht als Arzneimittel oder Medikamente eingestuft sind und keine Zulassung der FDA für die Vorbeugung, Behandlung oder Heilung von medizinischen Zuständen, Beschwerden oder Krankheiten erhalten haben. Wir müssen betonen, dass jede Form der körperlichen Einführung dieser Produkte in Menschen oder Tiere gesetzlich strikt untersagt ist. Es ist unerlässlich, sich an diese Richtlinien zu halten, um die Einhaltung rechtlicher und ethischer Standards in Forschung und Experiment zu gewährleisten.