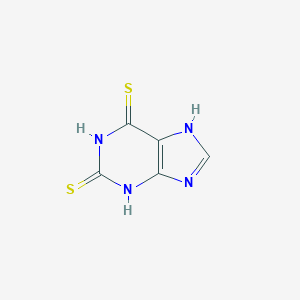
2,6-Dithiopurin
Übersicht
Beschreibung
Wissenschaftliche Forschungsanwendungen
2,6-Dimercaptopurin wurde für verschiedene wissenschaftliche Forschungsanwendungen untersucht:
Chemie: Als Ligand in der Koordinationschemie zur Detektion und Quantifizierung von Metallionen verwendet.
Biologie: Aufgrund seiner strukturellen Ähnlichkeit zu Purinbasen wurde sein Potenzial als Enzyminhibitor untersucht.
Medizin: Es wurde wegen seines potenziellen Antitumor-Potenzials und als Therapeutikum bei der Behandlung bestimmter Krankheiten untersucht.
Industrie: Einsatz bei der Entwicklung von Sensoren zur Detektion von Schwermetallen in Umwelt- und biologischen Proben.
5. Wirkmechanismus
Der Wirkmechanismus von 2,6-Dimercaptopurin beinhaltet seine Interaktion mit verschiedenen molekularen Zielen und Signalwegen:
Enzyminhibition: Die Verbindung kann Enzyme, die am Purinstoffwechsel beteiligt sind, hemmen, indem sie die Struktur natürlicher Purinsubstrate imitiert.
Inhibition der DNA- und RNA-Synthese: Es kann die Synthese von DNA und RNA stören, indem es sich in die Nukleinsäureketten einbaut, was zu einer Störung der normalen Zellprozesse führt.
Chelatbildung: Die Mercaptogruppen können Metallionen chelatieren, was die Aktivität von Metalloenzymen und anderen metall-abhängigen biologischen Prozessen beeinflussen kann.
Wirkmechanismus
Target of Action
2,6-Dithiopurine (DTP), also known as 2,6-Dimercaptopurine, is a sulfur-substituted nucleobase . It primarily targets cancer cells, particularly skin cancer cells . The compound’s primary targets are the reactive triplet states of these cells .
Mode of Action
DTP interacts with its targets through a process known as intersystem crossing . This process leads to the population of the reactive triplet state of the cancer cells . DTP populates the triplet manifold in less than 150 fs, reaching the nπ* triplet state minimum within a lifetime of 280 ± 50 fs . Subsequently, the population in the nπ* triplet state minimum internally converts to the long-lived ππ* triplet state within a lifetime of 3 ± 1 ps .
Biochemical Pathways
The biochemical pathways affected by DTP involve the electronic relaxation pathways leading to the population of the reactive triplet state of the cancer cells . This process is associated with major conformational relaxation in going from the nπ* to ππ* triplet state minimum .
Pharmacokinetics
The compound’s mode of action suggests that it is rapidly active, reaching its target state in less than 150 fs
Result of Action
The result of DTP’s action is the inhibition of cell proliferation. In studies, DTP has been shown to inhibit cell proliferation of up to 63% when combined with a low UVA dose of 5 J cm−2 . This makes DTP an effective agent in the treatment of skin cancer .
Action Environment
The action of DTP can be influenced by environmental factors such as light exposure. For instance, the effectiveness of DTP increases when combined with a low dose of UVA light . This suggests that the compound’s action, efficacy, and stability may be influenced by the light environment in which it is used.
Vorbereitungsmethoden
Synthesewege und Reaktionsbedingungen
Die Synthese von 2,6-Dimercaptopurin kann durch verschiedene Verfahren erreicht werden. Ein gängiger Ansatz beinhaltet die Reaktion von 2,6-Dichlorpurin mit Thioharnstoff. Die Reaktion findet typischerweise in wässrigem Medium unter Rückflussbedingungen statt, was zur Bildung von 2,6-Dimercaptopurin führt. Die Reaktion kann wie folgt dargestellt werden:
2,6-Dichloropurin+Thiourea→2,6-Dimercaptopurin+Hydrogen Chloride
Eine andere Methode beinhaltet die Verwendung von mit 2,6-Dimercaptopurin funktionalisierten Goldnanopartikeln zur sensitiven und selektiven kolorimetrischen Bestimmung von Cadmiumionen in verschiedenen Proben .
Industrielle Produktionsverfahren
Industrielle Produktionsverfahren für 2,6-Dimercaptopurin sind in der Literatur nicht gut dokumentiert. Die oben genannten Syntheseverfahren können für die industrielle Produktion mit geeigneter Optimierung der Reaktionsbedingungen und Reinigungsprozesse hochskaliert werden.
Analyse Chemischer Reaktionen
Reaktionstypen
2,6-Dimercaptopurin unterliegt verschiedenen chemischen Reaktionen, darunter:
Oxidation: Die Mercaptogruppen können oxidiert werden, um Disulfide zu bilden.
Substitution: Die Mercaptogruppen können an Substitutionsreaktionen mit Elektrophilen teilnehmen.
Chelatbildung: Die Verbindung kann Chelate mit Metallionen bilden, was in der analytischen Chemie nützlich ist.
Häufige Reagenzien und Bedingungen
Oxidationsmittel: Wasserstoffperoxid, Iod.
Elektrophile: Alkylhalogenide, Acylhalogenide.
Chelatbildner: Metallionen wie Cadmium, Gold.
Hauptprodukte, die gebildet werden
Disulfide: Durch Oxidation von Mercaptogruppen gebildet.
Substituierte Derivate: Durch Substitutionsreaktionen gebildet.
Metallchelate: Durch Chelatbildung mit Metallionen gebildet.
Vergleich Mit ähnlichen Verbindungen
2,6-Dimercaptopurin kann mit anderen ähnlichen Verbindungen verglichen werden, wie z. B.:
6-Mercaptopurin: Ein bekanntes Antimetabolit, das zur Behandlung von Leukämie eingesetzt wird. Im Gegensatz zu 2,6-Dimercaptopurin hat es nur eine Mercaptogruppe an der Position 6.
2,6-Dithiopurin: Ein weiteres Derivat mit ähnlichen Eigenschaften, aber unterschiedlichen Anwendungen.
Thiopurinol: Eine Verbindung, die mit 6-Mercaptopurin verwandt ist und aufgrund ihrer chemopräventiven Eigenschaften verwendet wird.
Eigenschaften
IUPAC Name |
3,7-dihydropurine-2,6-dithione | |
---|---|---|
Source | PubChem | |
URL | https://pubchem.ncbi.nlm.nih.gov | |
Description | Data deposited in or computed by PubChem | |
InChI |
InChI=1S/C5H4N4S2/c10-4-2-3(7-1-6-2)8-5(11)9-4/h1H,(H3,6,7,8,9,10,11) | |
Source | PubChem | |
URL | https://pubchem.ncbi.nlm.nih.gov | |
Description | Data deposited in or computed by PubChem | |
InChI Key |
VQPMXSMUUILNFZ-UHFFFAOYSA-N | |
Source | PubChem | |
URL | https://pubchem.ncbi.nlm.nih.gov | |
Description | Data deposited in or computed by PubChem | |
Canonical SMILES |
C1=NC2=C(N1)C(=S)NC(=S)N2 | |
Source | PubChem | |
URL | https://pubchem.ncbi.nlm.nih.gov | |
Description | Data deposited in or computed by PubChem | |
Molecular Formula |
C5H4N4S2 | |
Source | PubChem | |
URL | https://pubchem.ncbi.nlm.nih.gov | |
Description | Data deposited in or computed by PubChem | |
DSSTOX Substance ID |
DTXSID00202744 | |
Record name | 2,6-Dithiopurine | |
Source | EPA DSSTox | |
URL | https://comptox.epa.gov/dashboard/DTXSID00202744 | |
Description | DSSTox provides a high quality public chemistry resource for supporting improved predictive toxicology. | |
Molecular Weight |
184.2 g/mol | |
Source | PubChem | |
URL | https://pubchem.ncbi.nlm.nih.gov | |
Description | Data deposited in or computed by PubChem | |
CAS No. |
5437-25-2 | |
Record name | 3,9-Dihydro-1H-purine-2,6-dithione | |
Source | CAS Common Chemistry | |
URL | https://commonchemistry.cas.org/detail?cas_rn=5437-25-2 | |
Description | CAS Common Chemistry is an open community resource for accessing chemical information. Nearly 500,000 chemical substances from CAS REGISTRY cover areas of community interest, including common and frequently regulated chemicals, and those relevant to high school and undergraduate chemistry classes. This chemical information, curated by our expert scientists, is provided in alignment with our mission as a division of the American Chemical Society. | |
Explanation | The data from CAS Common Chemistry is provided under a CC-BY-NC 4.0 license, unless otherwise stated. | |
Record name | 2,6-Dithiopurine | |
Source | ChemIDplus | |
URL | https://pubchem.ncbi.nlm.nih.gov/substance/?source=chemidplus&sourceid=0005437252 | |
Description | ChemIDplus is a free, web search system that provides access to the structure and nomenclature authority files used for the identification of chemical substances cited in National Library of Medicine (NLM) databases, including the TOXNET system. | |
Record name | Purine-2,6-dithiol | |
Source | DTP/NCI | |
URL | https://dtp.cancer.gov/dtpstandard/servlet/dwindex?searchtype=NSC&outputformat=html&searchlist=15989 | |
Description | The NCI Development Therapeutics Program (DTP) provides services and resources to the academic and private-sector research communities worldwide to facilitate the discovery and development of new cancer therapeutic agents. | |
Explanation | Unless otherwise indicated, all text within NCI products is free of copyright and may be reused without our permission. Credit the National Cancer Institute as the source. | |
Record name | 2,6-Dithiopurine | |
Source | EPA DSSTox | |
URL | https://comptox.epa.gov/dashboard/DTXSID00202744 | |
Description | DSSTox provides a high quality public chemistry resource for supporting improved predictive toxicology. | |
Record name | 3,7-dihydro-1H-purine-2,6-dithione | |
Source | European Chemicals Agency (ECHA) | |
URL | https://echa.europa.eu/substance-information/-/substanceinfo/100.024.189 | |
Description | The European Chemicals Agency (ECHA) is an agency of the European Union which is the driving force among regulatory authorities in implementing the EU's groundbreaking chemicals legislation for the benefit of human health and the environment as well as for innovation and competitiveness. | |
Explanation | Use of the information, documents and data from the ECHA website is subject to the terms and conditions of this Legal Notice, and subject to other binding limitations provided for under applicable law, the information, documents and data made available on the ECHA website may be reproduced, distributed and/or used, totally or in part, for non-commercial purposes provided that ECHA is acknowledged as the source: "Source: European Chemicals Agency, http://echa.europa.eu/". Such acknowledgement must be included in each copy of the material. ECHA permits and encourages organisations and individuals to create links to the ECHA website under the following cumulative conditions: Links can only be made to webpages that provide a link to the Legal Notice page. | |
Synthesis routes and methods I
Procedure details
Synthesis routes and methods II
Procedure details
Retrosynthesis Analysis
AI-Powered Synthesis Planning: Our tool employs the Template_relevance Pistachio, Template_relevance Bkms_metabolic, Template_relevance Pistachio_ringbreaker, Template_relevance Reaxys, Template_relevance Reaxys_biocatalysis model, leveraging a vast database of chemical reactions to predict feasible synthetic routes.
One-Step Synthesis Focus: Specifically designed for one-step synthesis, it provides concise and direct routes for your target compounds, streamlining the synthesis process.
Accurate Predictions: Utilizing the extensive PISTACHIO, BKMS_METABOLIC, PISTACHIO_RINGBREAKER, REAXYS, REAXYS_BIOCATALYSIS database, our tool offers high-accuracy predictions, reflecting the latest in chemical research and data.
Strategy Settings
Precursor scoring | Relevance Heuristic |
---|---|
Min. plausibility | 0.01 |
Model | Template_relevance |
Template Set | Pistachio/Bkms_metabolic/Pistachio_ringbreaker/Reaxys/Reaxys_biocatalysis |
Top-N result to add to graph | 6 |
Feasible Synthetic Routes
Haftungsausschluss und Informationen zu In-Vitro-Forschungsprodukten
Bitte beachten Sie, dass alle Artikel und Produktinformationen, die auf BenchChem präsentiert werden, ausschließlich zu Informationszwecken bestimmt sind. Die auf BenchChem zum Kauf angebotenen Produkte sind speziell für In-vitro-Studien konzipiert, die außerhalb lebender Organismen durchgeführt werden. In-vitro-Studien, abgeleitet von dem lateinischen Begriff "in Glas", beinhalten Experimente, die in kontrollierten Laborumgebungen unter Verwendung von Zellen oder Geweben durchgeführt werden. Es ist wichtig zu beachten, dass diese Produkte nicht als Arzneimittel oder Medikamente eingestuft sind und keine Zulassung der FDA für die Vorbeugung, Behandlung oder Heilung von medizinischen Zuständen, Beschwerden oder Krankheiten erhalten haben. Wir müssen betonen, dass jede Form der körperlichen Einführung dieser Produkte in Menschen oder Tiere gesetzlich strikt untersagt ist. Es ist unerlässlich, sich an diese Richtlinien zu halten, um die Einhaltung rechtlicher und ethischer Standards in Forschung und Experiment zu gewährleisten.