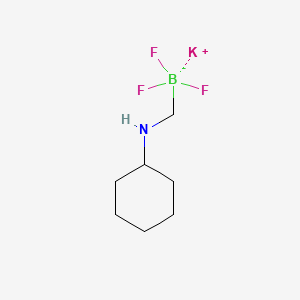
Kalium ((Cyclohexylamino)methyl)trifluoroborat
Übersicht
Beschreibung
Potassium ((cyclohexylamino)methyl)trifluoroborate is a useful research compound. Its molecular formula is C7H14BF3KN and its molecular weight is 219.1 g/mol. The purity is usually 95%.
BenchChem offers high-quality Potassium ((cyclohexylamino)methyl)trifluoroborate suitable for many research applications. Different packaging options are available to accommodate customers' requirements. Please inquire for more information about Potassium ((cyclohexylamino)methyl)trifluoroborate including the price, delivery time, and more detailed information at info@benchchem.com.
Wissenschaftliche Forschungsanwendungen
Enantioselektive Synthese
Kaliumtrifluoroboratsalze werden in der enantioselektiven Synthese von α-Aminosäureestern durch die Petasis-Borono-Mannich-Mehrkomponentenreaktion verwendet. Diese Reaktion ist von Bedeutung für die Herstellung von optisch aktiven α-Aminosäureestern, die wichtige Vorstufen für α-Aminosäurederivate sind, die in bioaktiven Naturstoffen und Arzneimitteln vorkommen .
Organische Synthese
Diese Salze ermöglichen verschiedene organische Synthesen, darunter Friedel-Crafts-Reaktionen, Mannich-Reaktionen, Michael-Additionen und [4+2]-Cycloadditionen. Die Vielseitigkeit von Kaliumtrifluoroboratsalzen ermöglicht die Herstellung hochfunktionalisierter Produkte aus einfachen Vorstufen .
Kreuzkupplungsreaktionen
Im Bereich der Kreuzkupplungschemie werden Kaliumtrifluoroboratsalze wegen ihrer Stabilität und Verträglichkeit mit starken oxidativen Bedingungen geschätzt. Sie dienen als vielseitige Kupplungspartner in einer Vielzahl von C–C-Bindungsbildungsreaktionen, die für die Konstruktion komplexer organischer Moleküle unerlässlich sind .
Epoxidierung ungesättigter Bindungen
Diese Salze sind an der Epoxidierung von C=C-Bindungen in ungesättigten Alkyl- oder Aryltrifluoroboraten beteiligt. Der Prozess verläuft mit vollständiger Umsetzung und Selektivität, was für die Synthese von Epoxiden entscheidend ist, ohne die Borfunktionalität zu beeinträchtigen .
Katalysatorentwicklung
Kaliumtrifluoroboratsalze werden bei der Entwicklung von Katalysatoren für die asymmetrische Synthese verwendet. So werden sie beispielsweise mit ®-BINOL-basierten Katalysatoren eingesetzt, um enantiomerenangereicherte α-Aminosäureester zu erzeugen und die Effizienz und Selektivität von Syntheseprozessen zu verbessern .
Funktionsgruppenverträglichkeit
Aufgrund ihrer hervorragenden Funktionsgruppenverträglichkeit werden diese Salze in Reaktionen verwendet, die eine hohe Toleranz gegenüber Luft und Feuchtigkeit erfordern. Diese Eigenschaft macht sie für Reaktionen geeignet, die empfindlich auf Umweltbedingungen reagieren .
Biochemische Analyse
Biochemical Properties
Potassium ((cyclohexylamino)methyl)trifluoroborate plays a significant role in biochemical reactions, particularly in the synthesis of boron-containing compounds. It interacts with enzymes, proteins, and other biomolecules through its trifluoroborate group, which can form stable complexes with various substrates. These interactions are crucial for facilitating reactions such as cross-coupling and borylation, which are essential in organic synthesis . The compound’s ability to form stable complexes with biomolecules makes it a valuable tool in biochemical research.
Cellular Effects
Potassium ((cyclohexylamino)methyl)trifluoroborate has been shown to influence various cellular processes. It can affect cell signaling pathways by interacting with key signaling molecules, leading to changes in gene expression and cellular metabolism. For example, the compound can modulate the activity of kinases and phosphatases, which are critical regulators of cell signaling. Additionally, it can impact cellular metabolism by altering the activity of enzymes involved in metabolic pathways . These effects highlight the compound’s potential as a modulator of cellular function.
Molecular Mechanism
The molecular mechanism of action of Potassium ((cyclohexylamino)methyl)trifluoroborate involves its ability to bind to specific biomolecules and alter their activity. The trifluoroborate group can form covalent bonds with nucleophilic sites on enzymes and proteins, leading to enzyme inhibition or activation. This binding can result in changes in gene expression by influencing transcription factors and other regulatory proteins. The compound’s ability to modulate enzyme activity and gene expression underscores its importance in biochemical research .
Temporal Effects in Laboratory Settings
In laboratory settings, the effects of Potassium ((cyclohexylamino)methyl)trifluoroborate can change over time due to its stability and degradation. The compound is stable under inert atmosphere conditions at low temperatures, but it can degrade when exposed to air or moisture. Long-term studies have shown that the compound can have sustained effects on cellular function, with changes in enzyme activity and gene expression persisting over extended periods . These temporal effects are important considerations for researchers using this compound in their experiments.
Dosage Effects in Animal Models
The effects of Potassium ((cyclohexylamino)methyl)trifluoroborate vary with different dosages in animal models. At low doses, the compound can modulate enzyme activity and gene expression without causing significant toxicity. At high doses, it can lead to adverse effects such as enzyme inhibition and cellular toxicity. Threshold effects have been observed, where the compound’s impact on cellular function increases sharply beyond a certain dosage . Understanding these dosage effects is crucial for determining the safe and effective use of the compound in research.
Transport and Distribution
Within cells and tissues, Potassium ((cyclohexylamino)methyl)trifluoroborate is transported and distributed through interactions with transporters and binding proteins. These interactions can affect the compound’s localization and accumulation in specific cellular compartments. For example, the compound can be transported into cells via specific transporters and then bind to intracellular proteins, influencing its distribution and activity . Understanding these transport and distribution mechanisms is important for optimizing the compound’s use in research.
Subcellular Localization
The subcellular localization of Potassium ((cyclohexylamino)methyl)trifluoroborate is influenced by targeting signals and post-translational modifications that direct it to specific compartments or organelles. The compound can localize to the cytoplasm, nucleus, or other organelles, depending on its interactions with cellular proteins. These localization patterns can affect the compound’s activity and function, making it important to study its subcellular distribution in detail .
Eigenschaften
IUPAC Name |
potassium;(cyclohexylamino)methyl-trifluoroboranuide | |
---|---|---|
Source | PubChem | |
URL | https://pubchem.ncbi.nlm.nih.gov | |
Description | Data deposited in or computed by PubChem | |
InChI |
InChI=1S/C7H14BF3N.K/c9-8(10,11)6-12-7-4-2-1-3-5-7;/h7,12H,1-6H2;/q-1;+1 | |
Source | PubChem | |
URL | https://pubchem.ncbi.nlm.nih.gov | |
Description | Data deposited in or computed by PubChem | |
InChI Key |
GYUWPHIATXXXJT-UHFFFAOYSA-N | |
Source | PubChem | |
URL | https://pubchem.ncbi.nlm.nih.gov | |
Description | Data deposited in or computed by PubChem | |
Canonical SMILES |
[B-](CNC1CCCCC1)(F)(F)F.[K+] | |
Source | PubChem | |
URL | https://pubchem.ncbi.nlm.nih.gov | |
Description | Data deposited in or computed by PubChem | |
Molecular Formula |
C7H14BF3KN | |
Source | PubChem | |
URL | https://pubchem.ncbi.nlm.nih.gov | |
Description | Data deposited in or computed by PubChem | |
DSSTOX Substance ID |
DTXSID80670568 | |
Record name | Potassium [(cyclohexylamino)methyl](trifluoro)borate(1-) | |
Source | EPA DSSTox | |
URL | https://comptox.epa.gov/dashboard/DTXSID80670568 | |
Description | DSSTox provides a high quality public chemistry resource for supporting improved predictive toxicology. | |
Molecular Weight |
219.10 g/mol | |
Source | PubChem | |
URL | https://pubchem.ncbi.nlm.nih.gov | |
Description | Data deposited in or computed by PubChem | |
CAS No. |
888711-52-2 | |
Record name | Potassium [(cyclohexylamino)methyl](trifluoro)borate(1-) | |
Source | EPA DSSTox | |
URL | https://comptox.epa.gov/dashboard/DTXSID80670568 | |
Description | DSSTox provides a high quality public chemistry resource for supporting improved predictive toxicology. | |
Retrosynthesis Analysis
AI-Powered Synthesis Planning: Our tool employs the Template_relevance Pistachio, Template_relevance Bkms_metabolic, Template_relevance Pistachio_ringbreaker, Template_relevance Reaxys, Template_relevance Reaxys_biocatalysis model, leveraging a vast database of chemical reactions to predict feasible synthetic routes.
One-Step Synthesis Focus: Specifically designed for one-step synthesis, it provides concise and direct routes for your target compounds, streamlining the synthesis process.
Accurate Predictions: Utilizing the extensive PISTACHIO, BKMS_METABOLIC, PISTACHIO_RINGBREAKER, REAXYS, REAXYS_BIOCATALYSIS database, our tool offers high-accuracy predictions, reflecting the latest in chemical research and data.
Strategy Settings
Precursor scoring | Relevance Heuristic |
---|---|
Min. plausibility | 0.01 |
Model | Template_relevance |
Template Set | Pistachio/Bkms_metabolic/Pistachio_ringbreaker/Reaxys/Reaxys_biocatalysis |
Top-N result to add to graph | 6 |
Feasible Synthetic Routes
Haftungsausschluss und Informationen zu In-Vitro-Forschungsprodukten
Bitte beachten Sie, dass alle Artikel und Produktinformationen, die auf BenchChem präsentiert werden, ausschließlich zu Informationszwecken bestimmt sind. Die auf BenchChem zum Kauf angebotenen Produkte sind speziell für In-vitro-Studien konzipiert, die außerhalb lebender Organismen durchgeführt werden. In-vitro-Studien, abgeleitet von dem lateinischen Begriff "in Glas", beinhalten Experimente, die in kontrollierten Laborumgebungen unter Verwendung von Zellen oder Geweben durchgeführt werden. Es ist wichtig zu beachten, dass diese Produkte nicht als Arzneimittel oder Medikamente eingestuft sind und keine Zulassung der FDA für die Vorbeugung, Behandlung oder Heilung von medizinischen Zuständen, Beschwerden oder Krankheiten erhalten haben. Wir müssen betonen, dass jede Form der körperlichen Einführung dieser Produkte in Menschen oder Tiere gesetzlich strikt untersagt ist. Es ist unerlässlich, sich an diese Richtlinien zu halten, um die Einhaltung rechtlicher und ethischer Standards in Forschung und Experiment zu gewährleisten.