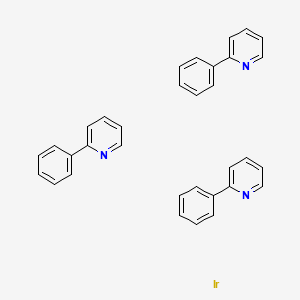
Tris(2-phenylpyridin)iridium
Übersicht
Beschreibung
Tris(2-phenylpyridine)iridium, abbreviated as [Ir(ppy)3], is an organoiridium complex with the formula Ir(C6H4-C5H4N)3 . It’s a yellow-green solid and a derivative of Ir3+ bound to three monoanionic 2-pyridinylphenyl ligands . It is electroluminescent, emitting green light .
Synthesis Analysis
The complex is prepared by cyclometalation reactions of 2-phenylpyridine and iridium trichloride, as represented by this idealized equation: IrCl3 + 3 C6H5-C5H4N → Ir(C6H4-C5H4N)3 + 3 HCl . A study also synthesized deuterated Ir(ppy)3-D24, which showed improved device stability and lifetime .Molecular Structure Analysis
The complex is observed with the facial stereochemistry, which is chiral . A computational study of Ir(ppy)3 has been presented .Chemical Reactions Analysis
The complex and many analogues have been investigated for application in photoredox catalysis . Its excited state has a reduction potential of −2.14 V, nearly 1 V more negative than the reduction potential of excited [Ru(bipy)3]2+ .Physical and Chemical Properties Analysis
Ir(ppy)3 is a yellow-green solid . It is electroluminescent, emitting green light . It has a molar mass of 654.793 g·mol−1 .Wissenschaftliche Forschungsanwendungen
Organische Leuchtdioden (OLEDs)
Ir(ppy)3 wird häufig bei der Synthese von elektrophosphoreszierenden Materialien für organische Leuchtdioden (OLEDs) . Es erzeugt eine grüne Lichtemission und eine hohe Phosphoreszenzquantenausbeute nahe eins .
Elektrolumineszente Dendrimere
Ir(ppy)3 wird bei der Herstellung von elektrolumineszenten Dendrimeren verwendet. Dies sind große, sternförmige Moleküle, die in OLEDs eingesetzt werden. Die Diffusionslänge des Triplett-Exzitons in diesen Dendrimeren liegt je nach Größe des Dendrons im Bereich von 2–10 nm .
Deuterium-Effekt in der Elektrophosphoreszenz
Die Synthese von vollständig deuteriertem Tris(2-phenylpyridin)iridium wurde wegen seiner hochstabilen Elektrophosphoreszenz untersucht . Ein darauf basierendes Gerät hat eine Stromdichte, die zwanzigmal höher ist als und eine Lebensdauer, die sechsmal länger ist als bei Geräten, die auf Ir(ppy)3 basieren . Dies ist auf die stabilere C–D-Bindung zurückzuführen, die als „Deuterium-Effekt“ bekannt ist .
Triplett-Exziton-Diffusion
Ir(ppy)3 wird zur Untersuchung der Triplett-Exziton-Diffusion verwendet. Dies ist ein Prozess, bei dem angeregte Zustände (Triplett-Exzitonen) sich von einer Stelle zur anderen innerhalb eines Materials bewegen. Die Abhängigkeitsbeziehung des Hüpfraten des nächsten Nachbarn vom Abstand zeigt, dass der Energietransfer vom Austauschmechanismus dominiert wird .
Hohe Quantenausbeuten
Ir(ppy)3 ist bekannt für seine hohen Quantenausbeuten . Die Quantenausbeute ist ein Maß für die Effizienz einer photonenproduzierenden Reaktion. Wenn eine Reaktion eine große Anzahl von Photonen erzeugt, hat sie eine hohe Quantenausbeute.
Thermische Stabilität
Ir(ppy)3 ist bekannt für seine thermische Stabilität . Dies macht es zu einem wertvollen Material in Anwendungen, bei denen hohe Temperaturen auftreten können.
Wirkmechanismus
Target of Action
Tris(2-phenylpyridine)iridium is an organoiridium complex . The primary targets of this compound are organic light-emitting diodes (OLEDs) where it is used as a precursor molecule for the synthesis of electro-phosphorescent materials .
Mode of Action
The compound interacts with its targets by emitting green light, a property known as electroluminescence . This is achieved through two possible mechanisms: direct charge trapping by the compound or energy transfer from another compound to Tris(2-phenylpyridine)iridium followed by carrier recombination .
Biochemical Pathways
The compound’s action affects the photoredox catalysis pathway . Its excited state has a reduction potential of -2.14 V, nearly 1 V more negative than the reduction potential of excited [Ru (bipy) 3] 2+ . This property makes it a strong photoreducing agent .
Result of Action
The result of Tris(2-phenylpyridine)iridium’s action is the emission of green light . This makes it valuable in the creation of OLEDs, contributing to their high efficiency .
Action Environment
The action, efficacy, and stability of Tris(2-phenylpyridine)iridium can be influenced by environmental factors. For instance, the compound’s electroluminescence can be affected by the presence of other compounds in the OLED . Additionally, the compound’s stability and lifetime in devices can be enhanced through deuteration, a process that replaces hydrogen atoms in the compound with deuterium .
Safety and Hazards
Zukünftige Richtungen
Ir(ppy)3 is the most frequently used precursor molecule for the synthesis of electro-phosphorescent materials, which are then used in organic light emitting diodes (OLEDs) . It provides green-color emission and high phosphorescence quantum yield close to unity . The complex has also been used as an efficient photocatalyst for a variety of organic processes .
Biochemische Analyse
Biochemical Properties
Tris(2-phenylpyridine)iridium plays a significant role in biochemical reactions, particularly in photoredox catalysis. It interacts with various enzymes and proteins, facilitating electron transfer processes. The compound’s excited state has a reduction potential of -2.14 V, making it a strong photoreducing agent . This property allows it to participate in redox reactions, where it can donate electrons to other molecules, thereby influencing various biochemical pathways.
Cellular Effects
Tris(2-phenylpyridine)iridium affects various types of cells and cellular processes. It has been shown to influence cell signaling pathways, gene expression, and cellular metabolism. The compound’s ability to emit light upon excitation makes it useful in imaging and tracking cellular processes. Additionally, its interaction with cellular components can lead to changes in cell function, including alterations in metabolic activity and gene expression patterns .
Molecular Mechanism
The mechanism of action of Tris(2-phenylpyridine)iridium involves its ability to bind to biomolecules and participate in electron transfer reactions. The compound’s strong photoreducing properties enable it to donate electrons to other molecules, thereby influencing their activity. This can result in the activation or inhibition of enzymes, changes in gene expression, and modulation of cellular signaling pathways . The binding interactions with biomolecules are crucial for its function in biochemical reactions.
Temporal Effects in Laboratory Settings
In laboratory settings, the effects of Tris(2-phenylpyridine)iridium can change over time. The compound is relatively stable, but its activity can be influenced by factors such as light exposure and temperature. Over time, degradation products may form, which can affect its long-term efficacy in biochemical reactions. Studies have shown that the compound maintains its stability and activity for extended periods under controlled conditions .
Dosage Effects in Animal Models
The effects of Tris(2-phenylpyridine)iridium vary with different dosages in animal models. At low doses, the compound can effectively participate in biochemical reactions without causing significant toxicity. At higher doses, it may exhibit toxic effects, including oxidative stress and cellular damage. Threshold effects have been observed, where the compound’s activity significantly changes at specific concentration levels .
Metabolic Pathways
Tris(2-phenylpyridine)iridium is involved in various metabolic pathways, primarily through its role in redox reactions. It interacts with enzymes and cofactors that facilitate electron transfer processes, influencing metabolic flux and metabolite levels. The compound’s ability to donate electrons makes it a key player in oxidative and reductive biochemical pathways .
Transport and Distribution
Within cells and tissues, Tris(2-phenylpyridine)iridium is transported and distributed through interactions with transporters and binding proteins. These interactions influence its localization and accumulation in specific cellular compartments. The compound’s distribution is crucial for its activity, as it needs to reach target sites to participate in biochemical reactions .
Subcellular Localization
Tris(2-phenylpyridine)iridium exhibits specific subcellular localization, which affects its activity and function. The compound may be directed to particular compartments or organelles through targeting signals or post-translational modifications. Its localization is essential for its role in biochemical reactions, as it needs to be in proximity to its target biomolecules .
Eigenschaften
{ "Design of the Synthesis Pathway": "The synthesis of Tris(2-phenylpyridine)iridium can be achieved through a multi-step process involving the reaction of various starting materials.", "Starting Materials": [ "2-Phenylpyridine", "Iridium(III) chloride hydrate", "Sodium hydroxide", "Methanol", "Acetone" ], "Reaction": [ "Step 1: Dissolve 2-Phenylpyridine in methanol and add sodium hydroxide to the solution.", "Step 2: Add iridium(III) chloride hydrate to the solution and stir for several hours.", "Step 3: Filter the resulting mixture and wash the solid with methanol.", "Step 4: Dissolve the solid in acetone and filter the solution.", "Step 5: Evaporate the acetone to obtain Tris(2-phenylpyridine)iridium as a solid." ] } | |
CAS-Nummer |
94928-86-6 |
Molekularformel |
C33H24IrN3 |
Molekulargewicht |
654.8 g/mol |
IUPAC-Name |
iridium(3+);2-phenylpyridine |
InChI |
InChI=1S/3C11H8N.Ir/c3*1-2-6-10(7-3-1)11-8-4-5-9-12-11;/h3*1-6,8-9H;/q3*-1;+3 |
InChI-Schlüssel |
NSABRUJKERBGOU-UHFFFAOYSA-N |
SMILES |
C1=CC=C(C=C1)C2=CC=CC=N2.C1=CC=C(C=C1)C2=CC=CC=N2.C1=CC=C(C=C1)C2=CC=CC=N2.[Ir] |
Kanonische SMILES |
C1=CC=C([C-]=C1)C2=CC=CC=N2.C1=CC=C([C-]=C1)C2=CC=CC=N2.C1=CC=C([C-]=C1)C2=CC=CC=N2.[Ir+3] |
693794-98-8 | |
Piktogramme |
Irritant |
Synonyme |
tris(2-phenylpyridine)iridium(III) |
Herkunft des Produkts |
United States |
Retrosynthesis Analysis
AI-Powered Synthesis Planning: Our tool employs the Template_relevance Pistachio, Template_relevance Bkms_metabolic, Template_relevance Pistachio_ringbreaker, Template_relevance Reaxys, Template_relevance Reaxys_biocatalysis model, leveraging a vast database of chemical reactions to predict feasible synthetic routes.
One-Step Synthesis Focus: Specifically designed for one-step synthesis, it provides concise and direct routes for your target compounds, streamlining the synthesis process.
Accurate Predictions: Utilizing the extensive PISTACHIO, BKMS_METABOLIC, PISTACHIO_RINGBREAKER, REAXYS, REAXYS_BIOCATALYSIS database, our tool offers high-accuracy predictions, reflecting the latest in chemical research and data.
Strategy Settings
Precursor scoring | Relevance Heuristic |
---|---|
Min. plausibility | 0.01 |
Model | Template_relevance |
Template Set | Pistachio/Bkms_metabolic/Pistachio_ringbreaker/Reaxys/Reaxys_biocatalysis |
Top-N result to add to graph | 6 |
Feasible Synthetic Routes
Q1: What is the molecular formula and weight of Ir(ppy)3?
A1: Ir(ppy)3 has a molecular formula of C33H24IrN3 and a molecular weight of 654.77 g/mol.
Q2: How does the structure of Ir(ppy)3 contribute to its phosphorescence?
A: Ir(ppy)3 exhibits strong phosphorescence due to the heavy atom effect of iridium, which enhances spin-orbit coupling (SOC). This allows for efficient intersystem crossing from singlet to triplet excited states. The triplet state, with its longer lifetime, is responsible for the observed phosphorescence. []
Q3: What are the characteristic spectroscopic features of Ir(ppy)3?
A: Ir(ppy)3 displays strong absorption in the UV region and intense green phosphorescence with a peak around 520 nm. [, ] Theoretical calculations using time-dependent density functional theory (TD-DFT) have been employed to accurately predict its UV absorption spectrum and understand the nature of electronic transitions involved. []
Q4: What makes Ir(ppy)3 suitable for OLED applications?
A: Ir(ppy)3 exhibits high luminescence quantum yields, approaching 100%, due to a triplet harvesting mechanism. [] This allows for efficient conversion of both singlet and triplet excitons into light, leading to highly efficient OLEDs. []
Q5: How stable is Ir(ppy)3 in OLED devices under prolonged operation?
A: While Ir(ppy)3 is generally considered stable, prolonged electrical driving of OLEDs can lead to degradation and a rise in driving voltage. Studies suggest that this is primarily due to the accumulation of holes at the emission layer/hole blocking layer interface. [] Strategic device engineering, such as eliminating guest molecules near the interface, can enhance voltage stability. []
Q6: Can Ir(ppy)3 be used in wet-processed OLED fabrication?
A: Yes, researchers have successfully incorporated Ir(ppy)3 into wet-processed OLEDs. This fabrication method utilizes soluble host materials like 2-[4-(carbazol-9-yl)butyloxy]-9-[4-(carbazol-9-yl)butyl]carbazole, allowing for large-scale, cost-effective production. []
Q7: How efficient is Ir(ppy)3 in electrochemiluminescence (ECL) systems?
A: Ir(ppy)3 demonstrates remarkably high ECL efficiencies, reaching up to 0.67 in acetonitrile-dioxane solutions, which is close to its photoluminescence quantum yield of 0.75. []
Q8: What is the role of tri-n-propylamine (TPrA) in Ir(ppy)3-based ECL systems?
A: TPrA acts as a coreactant in Ir(ppy)3-based ECL systems. It undergoes anodic oxidation, generating radical species that react with electrochemically generated Ir(ppy)3 ions, leading to the formation of excited-state Ir(ppy)3 and subsequent light emission. [, ]
Q9: Can Ir(ppy)3 be used in ECL-based biosensors?
A: Yes, Ir(ppy)3's excellent ECL properties make it suitable for biosensing applications. For example, encapsulating Ir(ppy)3 within apoferritin (apoFt) creates an aggregation-induced ECL bioconjugate. This bioconjugate can be further functionalized with antibodies for sensitive detection of biomarkers like cytokeratin 19 fragment 21-1 (CYFRA 21-1). []
Q10: How can computational chemistry contribute to understanding Ir(ppy)3 and its derivatives?
A: Computational methods, such as density functional theory (DFT) and time-dependent DFT (TD-DFT), provide valuable insights into the electronic structure, excited state properties, and spectroscopic characteristics of Ir(ppy)3. [] This knowledge is crucial for understanding its photophysical behavior and designing new derivatives with tailored properties.
Q11: How do structural modifications affect the emission color of Ir(ppy)3 derivatives?
A: Modifying the ligand structure of Ir(ppy)3 significantly impacts its emission color. For instance, introducing electron-withdrawing fluorine atoms into the bipyridine ligands of Ir(ppy)3 leads to a blue shift in emission. [] Conversely, incorporating electron-donating groups or extending the π-conjugation system generally results in a red shift. [, ]
Q12: Can Ir(ppy)3 be used as a photocatalyst?
A: Yes, Ir(ppy)3 and its derivatives have shown promising activity as photocatalysts. For example, incorporating Ir(ppy)3 into conjugated microporous polymers (CPOPs) yielded materials with high surface areas and excellent catalytic activity for the heterogeneous photocatalytic aza-Henry reaction. []
Q13: Are there other applications of Ir(ppy)3 beyond OLEDs and ECL?
A: Yes, Ir(ppy)3 has found applications in areas such as photopolymerization initiation [], surface modification of colloidal particles for potential use in optoelectronic devices [], and development of multifunctional molecular capsules for dual catalysis. []
Haftungsausschluss und Informationen zu In-Vitro-Forschungsprodukten
Bitte beachten Sie, dass alle Artikel und Produktinformationen, die auf BenchChem präsentiert werden, ausschließlich zu Informationszwecken bestimmt sind. Die auf BenchChem zum Kauf angebotenen Produkte sind speziell für In-vitro-Studien konzipiert, die außerhalb lebender Organismen durchgeführt werden. In-vitro-Studien, abgeleitet von dem lateinischen Begriff "in Glas", beinhalten Experimente, die in kontrollierten Laborumgebungen unter Verwendung von Zellen oder Geweben durchgeführt werden. Es ist wichtig zu beachten, dass diese Produkte nicht als Arzneimittel oder Medikamente eingestuft sind und keine Zulassung der FDA für die Vorbeugung, Behandlung oder Heilung von medizinischen Zuständen, Beschwerden oder Krankheiten erhalten haben. Wir müssen betonen, dass jede Form der körperlichen Einführung dieser Produkte in Menschen oder Tiere gesetzlich strikt untersagt ist. Es ist unerlässlich, sich an diese Richtlinien zu halten, um die Einhaltung rechtlicher und ethischer Standards in Forschung und Experiment zu gewährleisten.