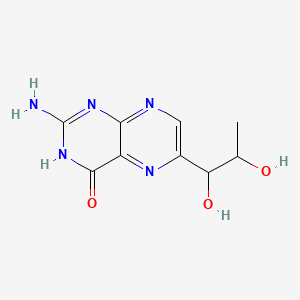
2-amino-6-(1,2-dihydroxypropyl)-3H-pteridin-4-one
Übersicht
Beschreibung
D-threo-Biopterin: ist ein Pterinderivat mit der chemischen Formel C9H11N5O3 und einem Molekulargewicht von 237,22 g/mol . .
Herstellungsmethoden
Synthesewege und Reaktionsbedingungen: Die Synthese von D-threo-Biopterin beinhaltet die Reaktion von Guanosintriphosphat mit spezifischen Enzymen, um Pteridin-Zwischenprodukte zu erzeugen . Der Prozess umfasst typischerweise die Umwandlung von Guanosintriphosphat zu Dihydroneopterin und Hydroxymethyldihydropteridin, die dann weiterverarbeitet werden, um D-threo-Biopterin zu erhalten .
Industrielle Produktionsmethoden: Industrielle Produktionsmethoden für D-threo-Biopterin sind nicht umfassend dokumentiert. Die Synthese folgt im Allgemeinen den enzymatischen Wegen, die in Laboreinstellungen verwendet werden, mit Optimierungen für die Skalierung und die Verbesserung der Ausbeute .
Vorbereitungsmethoden
Synthetic Routes and Reaction Conditions: The synthesis of D-threo-Biopterin involves the reaction of guanosine triphosphate with specific enzymes to produce pteridine intermediates . The process typically includes the conversion of guanosine triphosphate to dihydroneopterin and hydroxymethyldihydropteridine, which are then further processed to yield D-threo-Biopterin .
Industrial Production Methods: Industrial production methods for D-threo-Biopterin are not extensively documented. the synthesis generally follows the enzymatic pathways used in laboratory settings, with optimizations for scale-up and yield improvement .
Analyse Chemischer Reaktionen
Arten von Reaktionen: D-threo-Biopterin durchläuft verschiedene chemische Reaktionen, darunter:
Oxidation: Es kann oxidiert werden, um verschiedene Pterinderivate zu bilden.
Substitution: Substitutionsreaktionen können den Pteridinring modifizieren, um verschiedene Derivate zu erzeugen.
Häufige Reagenzien und Bedingungen:
Oxidation: Häufige Oxidationsmittel sind Wasserstoffperoxid und Kaliumpermanganat.
Reduktion: Reduktionsmittel wie Natriumborhydrid werden verwendet.
Substitution: Die Bedingungen variieren je nach der gewünschten Substitution, beinhalten aber häufig saure oder basische Katalysatoren.
Hauptprodukte, die gebildet werden:
Oxidation: Erzeugt oxidierte Pterinderivate.
Reduktion: Bildet Tetrahydrobiopterin.
Substitution: Liefert verschiedene substituierte Pterine.
Wissenschaftliche Forschungsanwendungen
D-threo-Biopterin hat mehrere wissenschaftliche Forschungsanwendungen:
Chemie: Wird als Reagenz in Chemilumineszenz-Assays verwendet, um die Luminol-Chemilumineszenz zu verstärken.
Wirkmechanismus
D-threo-Biopterin übt seine Wirkungen aus, indem es als Cofaktor in verschiedenen enzymatischen Reaktionen wirkt. Es ist an der Biosynthese von Neurotransmittern beteiligt, indem es als Cofaktor für Enzyme wie Phenylalaninhydroxylase, Tyrosinhydroxylase und Tryptophanhydroxylase dient . Diese Enzyme katalysieren Schlüsselreaktionen bei der Synthese von Dopamin, Serotonin, Noradrenalin und Adrenalin .
Wissenschaftliche Forschungsanwendungen
Antimicrobial Activity
Research has indicated that derivatives of pteridine compounds exhibit antimicrobial properties. Specifically, 2-amino-6-(1,2-dihydroxypropyl)-3H-pteridin-4-one has been studied for its activity against various bacterial strains. For instance, a study demonstrated its effectiveness against Staphylococcus aureus and Escherichia coli, suggesting potential applications in developing new antibiotics .
Anticancer Properties
The compound shows promise as an anticancer agent. In vitro studies have reported that it can inhibit the proliferation of cancer cell lines, including those from breast and colon cancers. The mechanism appears to involve the induction of apoptosis and inhibition of cell cycle progression .
Neurological Applications
There is emerging evidence that this compound may have neuroprotective effects. It has been investigated for its role in treating neurodegenerative diseases like Alzheimer's disease by inhibiting the formation of amyloid plaques .
Enzyme Inhibition
The compound acts as an inhibitor of several enzymes involved in metabolic pathways. Notably, it has been shown to inhibit pteridine reductase, which is crucial in the salvage pathway of folate metabolism in protozoan parasites like Trypanosoma brucei. This inhibition could lead to therapeutic strategies against diseases such as African sleeping sickness .
Structural Characteristics
The structural features of this compound contribute significantly to its biological activity. The presence of amino and hydroxyl groups enhances its solubility and interaction with biological targets, facilitating better absorption and efficacy in therapeutic applications .
Case Studies
Wirkmechanismus
D-threo-Biopterin exerts its effects by acting as a cofactor in various enzymatic reactions. It is involved in the biosynthesis of neurotransmitters by serving as a cofactor for enzymes such as phenylalanine hydroxylase, tyrosine hydroxylase, and tryptophan hydroxylase . These enzymes catalyze key reactions in the synthesis of dopamine, serotonin, norepinephrine, and epinephrine .
Vergleich Mit ähnlichen Verbindungen
Ähnliche Verbindungen:
D-threo-Neopterin: Ein Pterinderivat, das als Vorläufer bei der Biosynthese von Biopterin dient.
Biopterin: Ein Naturprodukt und essentieller Cofaktor bei der Neurotransmittersynthese.
Vergleich:
D-threo-Biopterin vs. D-threo-Neopterin: Während beide Pterinderivate sind, ist D-threo-Biopterin direkt an der Neurotransmittersynthese beteiligt, während D-threo-Neopterin als Vorläufer dient.
D-threo-Biopterin vs. Biopterin: D-threo-Biopterin ist ein spezifisches Isomer mit unterschiedlichen biochemischen Eigenschaften, während Biopterin eine breitere Kategorie ist, die verschiedene Isomere und Derivate umfasst.
Die einzigartige Rolle von D-threo-Biopterin als Cofaktor bei der Neurotransmittersynthese und seine spezifischen chemischen Eigenschaften machen es zu einer wertvollen Verbindung in der wissenschaftlichen Forschung und bei potenziellen medizinischen Anwendungen.
Biologische Aktivität
2-amino-6-(1,2-dihydroxypropyl)-3H-pteridin-4-one, also known as D-threo-Biopterin, is a pteridine derivative that plays a crucial role in various biological processes, particularly in the biosynthesis of neurotransmitters. This compound is a cofactor for several enzymes involved in the metabolism of amino acids and the production of neurotransmitters such as dopamine and serotonin.
- Molecular Formula : C9H11N5O3
- Molar Mass : 237.22 g/mol
- CAS Number : 36183-24-1
- Melting Point : >210°C (decomposes)
The compound exhibits significant stability under physiological conditions but can undergo oxidation under certain circumstances, leading to the formation of various metabolites.
D-threo-Biopterin functions primarily as a cofactor for:
- Phenylalanine hydroxylase : Converts phenylalanine to tyrosine.
- Tyrosine hydroxylase : Converts tyrosine to L-DOPA.
- Tryptophan hydroxylase : Converts tryptophan to serotonin.
These enzymatic reactions are essential for the synthesis of neurotransmitters, which are critical for normal brain function and mood regulation.
Neurotransmitter Synthesis
Research indicates that D-threo-Biopterin is vital for the biosynthesis of neurotransmitters. A deficiency in this compound can lead to disorders associated with neurotransmitter imbalances, such as depression and schizophrenia. Studies have shown that supplementation with biopterin can enhance dopamine and serotonin levels in animal models, suggesting its therapeutic potential in mood disorders .
Cytotoxic Effects
In vitro studies have reported that 6-biopterin, an oxidation product of D-threo-Biopterin, exhibits cytotoxicity towards human melanocytes. This effect is attributed to oxidative stress induced by the compound, highlighting the dual nature of biopterins in cellular environments .
Antioxidant Properties
D-threo-Biopterin also possesses antioxidant properties, which may contribute to its protective effects against oxidative damage in neuronal cells. It has been suggested that this compound can scavenge free radicals and reduce oxidative stress, potentially mitigating neurodegenerative processes .
Case Study 1: Role in Neurotransmitter Regulation
A study conducted on animal models demonstrated that administration of D-threo-Biopterin significantly increased levels of dopamine and serotonin in the brain. The study indicated improvements in mood and cognitive function, supporting its potential use as a therapeutic agent for mood disorders .
Case Study 2: Cytotoxicity in Melanocytes
In vitro experiments showed that 6-biopterin induced apoptosis in human melanocytes through oxidative stress mechanisms. This finding raises concerns about the safety profile of biopterins when used in therapeutic contexts involving skin cells .
Data Tables
Property | Value |
---|---|
Molecular Formula | C9H11N5O3 |
Molar Mass | 237.22 g/mol |
Melting Point | >210°C (dec.) |
CAS Number | 36183-24-1 |
Biological Activity | Effect |
---|---|
Neurotransmitter Synthesis | Increases dopamine and serotonin levels |
Cytotoxicity | Induces apoptosis in melanocytes |
Antioxidant Activity | Scavenges free radicals |
Eigenschaften
IUPAC Name |
2-amino-6-(1,2-dihydroxypropyl)-3H-pteridin-4-one | |
---|---|---|
Source | PubChem | |
URL | https://pubchem.ncbi.nlm.nih.gov | |
Description | Data deposited in or computed by PubChem | |
InChI |
InChI=1S/C9H11N5O3/c1-3(15)6(16)4-2-11-7-5(12-4)8(17)14-9(10)13-7/h2-3,6,15-16H,1H3,(H3,10,11,13,14,17) | |
Source | PubChem | |
URL | https://pubchem.ncbi.nlm.nih.gov | |
Description | Data deposited in or computed by PubChem | |
InChI Key |
LHQIJBMDNUYRAM-UHFFFAOYSA-N | |
Source | PubChem | |
URL | https://pubchem.ncbi.nlm.nih.gov | |
Description | Data deposited in or computed by PubChem | |
Canonical SMILES |
CC(C(C1=CN=C2C(=N1)C(=O)NC(=N2)N)O)O | |
Source | PubChem | |
URL | https://pubchem.ncbi.nlm.nih.gov | |
Description | Data deposited in or computed by PubChem | |
Molecular Formula |
C9H11N5O3 | |
Source | PubChem | |
URL | https://pubchem.ncbi.nlm.nih.gov | |
Description | Data deposited in or computed by PubChem | |
Molecular Weight |
237.22 g/mol | |
Source | PubChem | |
URL | https://pubchem.ncbi.nlm.nih.gov | |
Description | Data deposited in or computed by PubChem | |
CAS No. |
36183-24-1, 22150-76-1 | |
Record name | (R,S)-(1)-2-Amino-6-(1,2-dihydroxypropyl)-1H-pteridin-4-one | |
Source | ChemIDplus | |
URL | https://pubchem.ncbi.nlm.nih.gov/substance/?source=chemidplus&sourceid=0036183241 | |
Description | ChemIDplus is a free, web search system that provides access to the structure and nomenclature authority files used for the identification of chemical substances cited in National Library of Medicine (NLM) databases, including the TOXNET system. | |
Record name | [S-(R*,S*)]-2-amino-6-(1,2-dihydroxypropyl)-1H-pteridin-4-one | |
Source | European Chemicals Agency (ECHA) | |
URL | https://echa.europa.eu/substance-information/-/substanceinfo/100.040.719 | |
Description | The European Chemicals Agency (ECHA) is an agency of the European Union which is the driving force among regulatory authorities in implementing the EU's groundbreaking chemicals legislation for the benefit of human health and the environment as well as for innovation and competitiveness. | |
Explanation | Use of the information, documents and data from the ECHA website is subject to the terms and conditions of this Legal Notice, and subject to other binding limitations provided for under applicable law, the information, documents and data made available on the ECHA website may be reproduced, distributed and/or used, totally or in part, for non-commercial purposes provided that ECHA is acknowledged as the source: "Source: European Chemicals Agency, http://echa.europa.eu/". Such acknowledgement must be included in each copy of the material. ECHA permits and encourages organisations and individuals to create links to the ECHA website under the following cumulative conditions: Links can only be made to webpages that provide a link to the Legal Notice page. | |
Record name | (R,S)-(±)-2-amino-6-(1,2-dihydroxypropyl)-1H-pteridin-4-one | |
Source | European Chemicals Agency (ECHA) | |
URL | https://echa.europa.eu/substance-information/-/substanceinfo/100.048.078 | |
Description | The European Chemicals Agency (ECHA) is an agency of the European Union which is the driving force among regulatory authorities in implementing the EU's groundbreaking chemicals legislation for the benefit of human health and the environment as well as for innovation and competitiveness. | |
Explanation | Use of the information, documents and data from the ECHA website is subject to the terms and conditions of this Legal Notice, and subject to other binding limitations provided for under applicable law, the information, documents and data made available on the ECHA website may be reproduced, distributed and/or used, totally or in part, for non-commercial purposes provided that ECHA is acknowledged as the source: "Source: European Chemicals Agency, http://echa.europa.eu/". Such acknowledgement must be included in each copy of the material. ECHA permits and encourages organisations and individuals to create links to the ECHA website under the following cumulative conditions: Links can only be made to webpages that provide a link to the Legal Notice page. | |
Retrosynthesis Analysis
AI-Powered Synthesis Planning: Our tool employs the Template_relevance Pistachio, Template_relevance Bkms_metabolic, Template_relevance Pistachio_ringbreaker, Template_relevance Reaxys, Template_relevance Reaxys_biocatalysis model, leveraging a vast database of chemical reactions to predict feasible synthetic routes.
One-Step Synthesis Focus: Specifically designed for one-step synthesis, it provides concise and direct routes for your target compounds, streamlining the synthesis process.
Accurate Predictions: Utilizing the extensive PISTACHIO, BKMS_METABOLIC, PISTACHIO_RINGBREAKER, REAXYS, REAXYS_BIOCATALYSIS database, our tool offers high-accuracy predictions, reflecting the latest in chemical research and data.
Strategy Settings
Precursor scoring | Relevance Heuristic |
---|---|
Min. plausibility | 0.01 |
Model | Template_relevance |
Template Set | Pistachio/Bkms_metabolic/Pistachio_ringbreaker/Reaxys/Reaxys_biocatalysis |
Top-N result to add to graph | 6 |
Feasible Synthetic Routes
Haftungsausschluss und Informationen zu In-Vitro-Forschungsprodukten
Bitte beachten Sie, dass alle Artikel und Produktinformationen, die auf BenchChem präsentiert werden, ausschließlich zu Informationszwecken bestimmt sind. Die auf BenchChem zum Kauf angebotenen Produkte sind speziell für In-vitro-Studien konzipiert, die außerhalb lebender Organismen durchgeführt werden. In-vitro-Studien, abgeleitet von dem lateinischen Begriff "in Glas", beinhalten Experimente, die in kontrollierten Laborumgebungen unter Verwendung von Zellen oder Geweben durchgeführt werden. Es ist wichtig zu beachten, dass diese Produkte nicht als Arzneimittel oder Medikamente eingestuft sind und keine Zulassung der FDA für die Vorbeugung, Behandlung oder Heilung von medizinischen Zuständen, Beschwerden oder Krankheiten erhalten haben. Wir müssen betonen, dass jede Form der körperlichen Einführung dieser Produkte in Menschen oder Tiere gesetzlich strikt untersagt ist. Es ist unerlässlich, sich an diese Richtlinien zu halten, um die Einhaltung rechtlicher und ethischer Standards in Forschung und Experiment zu gewährleisten.