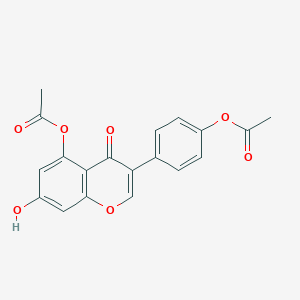
4',5-Di-O-acetyl Genistein
- Klicken Sie auf QUICK INQUIRY, um ein Angebot von unserem Expertenteam zu erhalten.
- Mit qualitativ hochwertigen Produkten zu einem WETTBEWERBSFÄHIGEN Preis können Sie sich mehr auf Ihre Forschung konzentrieren.
Übersicht
Beschreibung
4',5-Di-O-acetyl Genistein is a synthetic compound with the molecular formula C19H14O7 and a molecular weight of 354.3 g/mol. This compound belongs to the class of organic compounds known as coumarins, which are characterized by a benzopyrone structure. Coumarins are widely recognized for their diverse biological activities and applications in various fields, including medicine, agriculture, and industry.
Vorbereitungsmethoden
The synthesis of 4',5-Di-O-acetyl Genistein involves several steps. One common method includes the acetylation of 7-hydroxy-4-oxochromen-3-yl phenol with acetic anhydride in the presence of a catalyst such as pyridine. The reaction is typically carried out under reflux conditions to ensure complete acetylation. The resulting product is then purified through recrystallization or column chromatography to obtain the desired compound in high purity.
Industrial production methods for this compound may involve similar synthetic routes but on a larger scale, utilizing industrial-grade reagents and equipment to achieve higher yields and efficiency.
Analyse Chemischer Reaktionen
4',5-Di-O-acetyl Genistein undergoes various chemical reactions, including:
Oxidation: This compound can be oxidized using common oxidizing agents such as potassium permanganate or chromium trioxide, leading to the formation of corresponding quinones or carboxylic acids.
Reduction: Reduction reactions can be carried out using reducing agents like sodium borohydride or lithium aluminum hydride, resulting in the formation of alcohols or other reduced derivatives.
Substitution: Nucleophilic substitution reactions can occur at the acetoxy or hydroxy groups, leading to the formation of various substituted derivatives. Common reagents for these reactions include alkyl halides, acyl chlorides, and amines.
The major products formed from these reactions depend on the specific reagents and conditions used, but they generally include a range of oxidized, reduced, or substituted coumarin derivatives.
Wissenschaftliche Forschungsanwendungen
Chemical Properties and Mechanism of Action
Chemical Structure : The molecular formula of 4',5-Di-O-acetyl Genistein is C19H14O7 with a molecular weight of 354.3 g/mol. It belongs to the class of coumarins and exhibits a benzopyrone structure.
Mechanism of Action :
- Cell Cycle Regulation : The compound interacts with cellular components involved in the regulation of the cell cycle.
- Targeted Pathways : It inhibits tyrosine kinases, DNA topoisomerases, and telomerase, leading to effects such as apoptosis induction and anti-inflammatory responses.
- Biochemical Pathways : It affects several pathways crucial for cancer progression and inflammation, making it a candidate for therapeutic interventions.
Biological Research
This compound is investigated for its antioxidant , anti-inflammatory , and antimicrobial properties. Its ability to inhibit specific enzymes makes it valuable in studying disease mechanisms.
Medical Applications
Research indicates potential uses in treating:
- Cancer : Studies have shown significant tumor growth inhibition in xenograft models, with up to 60% reduction at doses of 20 mg/kg.
- Cardiovascular Diseases : Its modulation of biological pathways suggests benefits in heart health.
- Neurodegenerative Disorders : The compound may protect against neuronal damage, indicating its potential in neuroprotective strategies.
Industrial Applications
In the industrial sector, this compound is utilized in the formulation of:
- Dyes and Fragrances : Its unique chemical properties enhance product formulations.
- Specialty Chemicals : The compound serves as an intermediate in synthesizing complex organic molecules due to its stable acetyl groups.
Case Study on Cancer Treatment
- Objective : Evaluate anticancer effects in breast cancer models.
- Results : Significant apoptosis induction was observed in cancer cells with minimal effects on normal cells, suggesting selective targeting.
Case Study on Infection Control
- Objective : Assess antimicrobial efficacy against resistant bacterial strains.
- Results : Effective inhibition of growth was noted in multi-drug resistant strains, highlighting its potential as an antimicrobial agent.
Data Table Summary
Application Area | Specific Uses | Key Findings |
---|---|---|
Biological Research | Antioxidant, anti-inflammatory | Inhibits key enzymes involved in disease processes |
Medicine | Cancer therapy, cardiovascular health | Significant tumor inhibition; neuroprotective effects |
Industrial Chemistry | Dyes, fragrances | Enhances stability and solubility |
Antimicrobial Research | Infection control | Effective against multi-drug resistant bacteria |
Wirkmechanismus
The mechanism of action of 4',5-Di-O-acetyl Genistein involves its interaction with specific molecular targets and pathways. It is known to inhibit certain enzymes, such as cyclooxygenases and lipoxygenases, which play a role in inflammation and pain. Additionally, it can modulate oxidative stress pathways by scavenging free radicals and enhancing the activity of antioxidant enzymes. These actions contribute to its potential therapeutic effects in various diseases.
Vergleich Mit ähnlichen Verbindungen
4',5-Di-O-acetyl Genistein can be compared with other similar compounds, such as:
Coumarin: The parent compound of this class, known for its anticoagulant properties and use in the synthesis of various pharmaceuticals.
Warfarin: A well-known anticoagulant derived from coumarin, used to prevent blood clots.
Esculetin: A natural coumarin derivative with antioxidant and anti-inflammatory properties.
Umbelliferone: Another natural coumarin with potential therapeutic applications in skin disorders and oxidative stress-related conditions.
The uniqueness of this compound lies in its specific acetoxy and hydroxy substitutions, which confer distinct chemical and biological properties compared to other coumarin derivatives.
Biologische Aktivität
4',5-Di-O-acetyl Genistein is a derivative of genistein, a well-known isoflavone primarily found in soy products. This compound is recognized for its potential biological activities, particularly in cancer treatment and anti-inflammatory applications. This article reviews the biological activity of this compound, focusing on its mechanisms of action, pharmacological effects, and relevant case studies.
This compound is structurally modified from genistein by the addition of acetyl groups at the 4' and 5' positions. This modification enhances its solubility and bioavailability, potentially increasing its efficacy in biological systems.
The biological activity of this compound can be attributed to several mechanisms:
- Estrogen Receptor Modulation : Similar to genistein, this compound exhibits estrogenic activity by binding to estrogen receptors (ERα and ERβ). This interaction can either mimic or inhibit estrogen's effects, influencing cell proliferation and apoptosis in hormone-sensitive cancers .
- Cell Cycle Regulation : Research shows that genistein derivatives can induce cell cycle arrest. Specifically, this compound has been reported to cause G1 phase arrest in cancer cell lines, which inhibits their proliferation .
- Apoptosis Induction : The compound promotes apoptosis through various pathways, including the upregulation of pro-apoptotic proteins and downregulation of anti-apoptotic proteins like Bcl-2. This shift in protein expression leads to increased mitochondrial permeability and subsequent cell death .
- Antioxidant Activity : this compound exhibits antioxidant properties by scavenging free radicals and enhancing the expression of antioxidant enzymes, thereby reducing oxidative stress within cells .
Pharmacological Effects
The pharmacological effects of this compound have been documented in various studies:
- Anticancer Effects : In vitro studies indicate that this compound significantly inhibits the growth of breast cancer cells by inducing apoptosis and modulating signaling pathways such as MAPK and PI3K/AKT .
- Anti-inflammatory Properties : The compound has shown promise in reducing inflammation markers such as IL-6 and TNF-α in various cell models. For instance, it effectively suppressed nitric oxide production in macrophages, indicating its potential use in inflammatory diseases .
- Neuroprotective Effects : Preliminary studies suggest that this compound may also provide neuroprotective benefits by enhancing autophagy and reducing neuroinflammation, making it a candidate for treating neurodegenerative disorders .
Case Studies
Several key studies highlight the efficacy of this compound:
- Breast Cancer Study : A study involving MCF-7 breast cancer cells demonstrated that treatment with this compound resulted in a significant reduction in cell viability and induced apoptosis through mitochondrial pathways .
- Inflammation Model : In an experimental model using RAW 264.7 macrophages, treatment with varying concentrations of this compound resulted in a dose-dependent decrease in pro-inflammatory cytokines (IL-1β and TNF-α) production .
- Neuroprotection Study : Research on neuroblastoma cells indicated that this compound enhanced cell survival under oxidative stress conditions by modulating autophagy-related pathways .
Comparative Table of Biological Activities
Eigenschaften
IUPAC Name |
[4-(5-acetyloxy-7-hydroxy-4-oxochromen-3-yl)phenyl] acetate |
Source
|
---|---|---|
Source | PubChem | |
URL | https://pubchem.ncbi.nlm.nih.gov | |
Description | Data deposited in or computed by PubChem | |
InChI |
InChI=1S/C19H14O7/c1-10(20)25-14-5-3-12(4-6-14)15-9-24-16-7-13(22)8-17(26-11(2)21)18(16)19(15)23/h3-9,22H,1-2H3 |
Source
|
Source | PubChem | |
URL | https://pubchem.ncbi.nlm.nih.gov | |
Description | Data deposited in or computed by PubChem | |
InChI Key |
SWRSZMILWBHUEZ-UHFFFAOYSA-N |
Source
|
Source | PubChem | |
URL | https://pubchem.ncbi.nlm.nih.gov | |
Description | Data deposited in or computed by PubChem | |
Canonical SMILES |
CC(=O)OC1=CC=C(C=C1)C2=COC3=C(C2=O)C(=CC(=C3)O)OC(=O)C |
Source
|
Source | PubChem | |
URL | https://pubchem.ncbi.nlm.nih.gov | |
Description | Data deposited in or computed by PubChem | |
Molecular Formula |
C19H14O7 |
Source
|
Source | PubChem | |
URL | https://pubchem.ncbi.nlm.nih.gov | |
Description | Data deposited in or computed by PubChem | |
Molecular Weight |
354.3 g/mol |
Source
|
Source | PubChem | |
URL | https://pubchem.ncbi.nlm.nih.gov | |
Description | Data deposited in or computed by PubChem | |
Haftungsausschluss und Informationen zu In-Vitro-Forschungsprodukten
Bitte beachten Sie, dass alle Artikel und Produktinformationen, die auf BenchChem präsentiert werden, ausschließlich zu Informationszwecken bestimmt sind. Die auf BenchChem zum Kauf angebotenen Produkte sind speziell für In-vitro-Studien konzipiert, die außerhalb lebender Organismen durchgeführt werden. In-vitro-Studien, abgeleitet von dem lateinischen Begriff "in Glas", beinhalten Experimente, die in kontrollierten Laborumgebungen unter Verwendung von Zellen oder Geweben durchgeführt werden. Es ist wichtig zu beachten, dass diese Produkte nicht als Arzneimittel oder Medikamente eingestuft sind und keine Zulassung der FDA für die Vorbeugung, Behandlung oder Heilung von medizinischen Zuständen, Beschwerden oder Krankheiten erhalten haben. Wir müssen betonen, dass jede Form der körperlichen Einführung dieser Produkte in Menschen oder Tiere gesetzlich strikt untersagt ist. Es ist unerlässlich, sich an diese Richtlinien zu halten, um die Einhaltung rechtlicher und ethischer Standards in Forschung und Experiment zu gewährleisten.