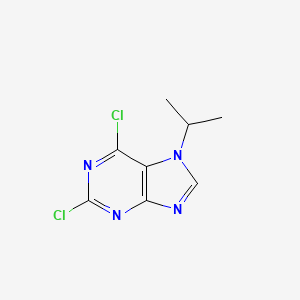
2,6-dichloro-7-isopropyl-7H-purine
Übersicht
Beschreibung
“2,6-dichloro-7-isopropyl-7H-purine” is a molecule with the formula C8H8Cl2N4 . The molecule is essentially planar with imidazole and pyrimidine rings . The maximum deviations of these rings are 0.0030 (15) and 0.0111 (15) Å, respectively . The rings make a dihedral angle of 1.32 (8)° .
Molecular Structure Analysis
In the crystal structure of the molecule, the fused-ring systems are stacked approximately parallel to the bc plane . The centroid-centroid distance between inversion-related pyrimidine rings is 3.5189 (9) Å .Physical And Chemical Properties Analysis
The molecule has an average mass of 231.082 Da and a monoisotopic mass of 230.012604 Da .Wissenschaftliche Forschungsanwendungen
Corrosion Inhibition
Research on purines, including derivatives similar to 2,6-dichloro-7-isopropyl-7H-purine, has identified them as potential corrosion inhibitors for metals. A study investigating various purines and their derivatives demonstrated their effectiveness in preventing corrosion of mild steel in acidic solutions. These compounds, including 2,6-dichloro derivatives, operate through both cathodic and anodic inhibition mechanisms, suggesting a broad potential for applications in protecting metal surfaces in industrial settings (Yan et al., 2008).
Biological Activity and Drug Synthesis
Synthesis studies have explored the modification of 2,6-dichloro-purine derivatives to discover new biological activities. For instance, modification of 2,6-dichloro-purine to produce new compounds has led to discoveries in inhibiting phosphodiesterase isozymes, indicating potential therapeutic applications in diseases where phosphodiesterase modulation is beneficial (Kozai & Maruyama, 1999).
Glycosylation for Nucleoside Analogues
Glycosylation studies of chloropurines, including 2,6-dichloropurine, have contributed to the development of novel nucleoside analogues. These studies have been crucial in the synthesis of compounds that can serve as intermediates for further chemical transformations or as potential therapeutic agents (Tranová & Stýskala, 2021).
Antitumor Activity
Research into the antiproliferative effects of 2,6-dichloro-purine derivatives on cancer cell lines has revealed significant potential for these compounds in cancer treatment. Specific derivatives have shown potent cytotoxic effects against various human solid tumor cell lines, highlighting the importance of these compounds in the development of new anticancer drugs (Morales et al., 2014).
Safety and Hazards
Eigenschaften
IUPAC Name |
2,6-dichloro-7-propan-2-ylpurine | |
---|---|---|
Source | PubChem | |
URL | https://pubchem.ncbi.nlm.nih.gov | |
Description | Data deposited in or computed by PubChem | |
InChI |
InChI=1S/C8H8Cl2N4/c1-4(2)14-3-11-7-5(14)6(9)12-8(10)13-7/h3-4H,1-2H3 | |
Source | PubChem | |
URL | https://pubchem.ncbi.nlm.nih.gov | |
Description | Data deposited in or computed by PubChem | |
InChI Key |
BMKLSAIGMUTOQX-UHFFFAOYSA-N | |
Source | PubChem | |
URL | https://pubchem.ncbi.nlm.nih.gov | |
Description | Data deposited in or computed by PubChem | |
Canonical SMILES |
CC(C)N1C=NC2=C1C(=NC(=N2)Cl)Cl | |
Source | PubChem | |
URL | https://pubchem.ncbi.nlm.nih.gov | |
Description | Data deposited in or computed by PubChem | |
Molecular Formula |
C8H8Cl2N4 | |
Source | PubChem | |
URL | https://pubchem.ncbi.nlm.nih.gov | |
Description | Data deposited in or computed by PubChem | |
Molecular Weight |
231.08 g/mol | |
Source | PubChem | |
URL | https://pubchem.ncbi.nlm.nih.gov | |
Description | Data deposited in or computed by PubChem | |
Retrosynthesis Analysis
AI-Powered Synthesis Planning: Our tool employs the Template_relevance Pistachio, Template_relevance Bkms_metabolic, Template_relevance Pistachio_ringbreaker, Template_relevance Reaxys, Template_relevance Reaxys_biocatalysis model, leveraging a vast database of chemical reactions to predict feasible synthetic routes.
One-Step Synthesis Focus: Specifically designed for one-step synthesis, it provides concise and direct routes for your target compounds, streamlining the synthesis process.
Accurate Predictions: Utilizing the extensive PISTACHIO, BKMS_METABOLIC, PISTACHIO_RINGBREAKER, REAXYS, REAXYS_BIOCATALYSIS database, our tool offers high-accuracy predictions, reflecting the latest in chemical research and data.
Strategy Settings
Precursor scoring | Relevance Heuristic |
---|---|
Min. plausibility | 0.01 |
Model | Template_relevance |
Template Set | Pistachio/Bkms_metabolic/Pistachio_ringbreaker/Reaxys/Reaxys_biocatalysis |
Top-N result to add to graph | 6 |
Feasible Synthetic Routes
Q1: What is the spatial arrangement of the rings in 2,6-dichloro-7-isopropyl-7H-purine?
A1: The molecule consists of fused imidazole and pyrimidine rings. While both rings are essentially planar individually, they are not perfectly coplanar. The dihedral angle between them is 1.32° [].
Q2: How are the molecules arranged in the crystal lattice?
A2: In the crystal, the this compound molecules are stacked in a parallel arrangement along the bc plane. The distance between the centroids of inversion-related pyrimidine rings is 3.5189 Å [].
Haftungsausschluss und Informationen zu In-Vitro-Forschungsprodukten
Bitte beachten Sie, dass alle Artikel und Produktinformationen, die auf BenchChem präsentiert werden, ausschließlich zu Informationszwecken bestimmt sind. Die auf BenchChem zum Kauf angebotenen Produkte sind speziell für In-vitro-Studien konzipiert, die außerhalb lebender Organismen durchgeführt werden. In-vitro-Studien, abgeleitet von dem lateinischen Begriff "in Glas", beinhalten Experimente, die in kontrollierten Laborumgebungen unter Verwendung von Zellen oder Geweben durchgeführt werden. Es ist wichtig zu beachten, dass diese Produkte nicht als Arzneimittel oder Medikamente eingestuft sind und keine Zulassung der FDA für die Vorbeugung, Behandlung oder Heilung von medizinischen Zuständen, Beschwerden oder Krankheiten erhalten haben. Wir müssen betonen, dass jede Form der körperlichen Einführung dieser Produkte in Menschen oder Tiere gesetzlich strikt untersagt ist. Es ist unerlässlich, sich an diese Richtlinien zu halten, um die Einhaltung rechtlicher und ethischer Standards in Forschung und Experiment zu gewährleisten.