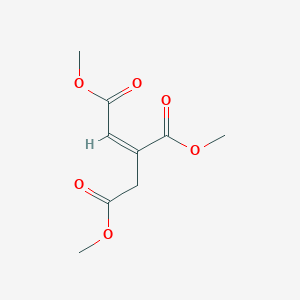
Trimethyl aconitate
Übersicht
Beschreibung
Trimethyl aconitate is an ester derivative of aconitic acid, characterized by the presence of three methoxy groups attached to the aconitic acid backbone. This compound is known for its high density of functional groups, making it a versatile reagent in organic synthesis and polymer chemistry. It is often used as a renewable raw material due to its derivation from bio-based sources.
Wirkmechanismus
Target of Action
Trimethyl aconitate, a derivative of aconitate, is a small molecule that plays a role in the tricarboxylic acid (TCA) cycle It’s parent compound, aconitate, is known to interact with the enzyme aconitase .
Mode of Action
Aconitate, from which this compound is derived, is known to be converted by aconitase in the tca cycle . Aconitase catalyzes the isomerization of citrate to isocitrate via cis-aconitate in the TCA cycle .
Biochemical Pathways
This compound is involved in the TCA cycle, a central metabolic pathway of cells . Itaconate, a byproduct of the TCA cycle, is an emerging metabolic small molecule that regulates macrophage inflammation . Itaconate is produced by the decarboxylation of cis-aconitate . The TCA cycle metabolites, including itaconate, play significant roles in controlling the immunophenotype and inflammatory response of macrophages .
Pharmacokinetics
It’s synthesized via a two-step palladium-catalysed carbonylation of propynyl alcohol .
Result of Action
Itaconate, a related compound, has been found to possess potent immunomodulatory characteristics . Itaconate regulates macrophage function through multiple mechanisms and has demonstrated promising therapeutic potential in a variety of immune and inflammatory diseases .
Action Environment
It’s known that various environmental factors can affect the action of biochemical compounds in general .
Biochemische Analyse
Biochemical Properties
Trimethyl aconitate participates in biochemical reactions within the TCA cycle . It interacts with various enzymes, proteins, and other biomolecules. For instance, it is synthesized from cis-aconitate via the enzyme aconitate decarboxylase 1 (ACOD1) . The nature of these interactions involves the conversion of cis-aconitate to itaconate, an immuno-regulatory metabolite .
Cellular Effects
This compound influences various types of cells and cellular processes. It plays a role in the regulation of macrophage immune metabolism . Itaconate, a derivative of this compound, has been shown to regulate macrophage function through multiple mechanisms .
Molecular Mechanism
The molecular mechanism of this compound involves its conversion to itaconate via the enzyme ACOD1 . This process affects metabolic pathways regulating inflammation and microbe survival . Itaconate exerts its effects at the molecular level, including binding interactions with biomolecules, enzyme inhibition or activation, and changes in gene expression .
Temporal Effects in Laboratory Settings
It is known that itaconate, a derivative of this compound, has been shown to have significant effects on macrophage function .
Dosage Effects in Animal Models
Animal models play a crucial role in advancing biomedical research, especially in the field of gene therapy .
Metabolic Pathways
This compound is involved in the TCA cycle, a central metabolic pathway of cells . It interacts with the enzyme ACOD1 in the conversion of cis-aconitate to itaconate .
Transport and Distribution
It is known that itaconate, a derivative of this compound, is produced in the mitochondria of immune cells .
Subcellular Localization
Itaconate, a derivative of this compound, is known to be produced in the mitochondria of immune cells .
Vorbereitungsmethoden
Synthetic Routes and Reaction Conditions: Trimethyl aconitate can be synthesized through various methods. One notable method involves the palladium-catalyzed triple carbonylation of propynyl alcohol. This two-step process includes an oxidative carbonylation followed by a substitutive carbonylation, yielding high amounts of E and Z aconitic trimethyl esters .
Another common preparation method involves the esterification of aconitic acid with methanol in the presence of a strong acid catalyst, such as concentrated sulfuric acid. The reaction mixture is refluxed overnight with continuous stirring to achieve the desired product .
Industrial Production Methods: In industrial settings, the production of this compound often involves the use of renewable feedstocks. The aza-Michael addition reaction between primary amines and trans-trimethyl aconitate is considered a green chemistry approach, proceeding quantitatively within minutes under ambient conditions .
Analyse Chemischer Reaktionen
Types of Reactions: Trimethyl aconitate undergoes various chemical reactions, including:
Aza-Michael Addition: This reaction involves the addition of nucleophilic amines to the electron-deficient alkene of this compound, forming N-alkyl-pyrrolidone structures.
Domino Reactions: These reactions consist of imine additions and intramolecular acylations, providing access to heteropolycycles in one-pot reactions.
Common Reagents and Conditions:
Aza-Michael Addition: Primary amines are used as nucleophiles, and the reaction proceeds under ambient conditions.
Domino Reactions: Imine formation and subsequent acylation are facilitated by the presence of suitable catalysts and solvents.
Major Products:
Aza-Michael Addition: Tetra-functional N-alkyl-bis-(pyrrolidone dimethylcarboxylate), which exhibits excellent thermal stability.
Domino Reactions:
Wissenschaftliche Forschungsanwendungen
Chemical Synthesis
1.1. Aza-Michael Addition Reactions
TMA has been extensively studied for its reactivity in aza-Michael addition reactions, where it acts as an acceptor for primary amines. This reaction is notable for its efficiency and specificity under mild conditions, aligning with green chemistry principles. The aza-Michael addition can yield N-substituted pyrrolidone derivatives, which have potential applications as surfactants and polymer precursors .
Table 1: Aza-Michael Addition Reaction Outcomes
Reactant | Product | Yield (%) |
---|---|---|
Benzylamine | N-benzyl pyrrolidone dimethylcarboxylate | 43-85 |
Para-xylylenediamine | N-substituted pyrrolidone dimethylcarboxylate | Moderate to High |
1.2. Knoevenagel Condensation
TMA can also undergo Knoevenagel condensation with aldehydes to form α,β-unsaturated esters. This reaction has been reported to yield products in the range of 36-93%, depending on the specific aldehyde used .
Polymer Chemistry
2.1. Cross-Linking Agent
In polymer chemistry, TMA serves as a cross-linking agent that enhances the properties of biopolymers. Its multifunctional carboxylic acid groups allow for the formation of polyesters that are biocompatible and suitable for applications such as tissue engineering .
Table 2: Applications of TMA in Polymer Chemistry
Application | Description |
---|---|
Tissue Engineering | Used to create biocompatible scaffolds for bone regeneration |
Drug Delivery Systems | Enhances solubility and bioavailability of drugs when used with chitosan-based microparticles |
Biological Applications
3.1. Metabolic Pathway Studies
TMA is a stable derivative of aconitic acid, which plays a crucial role in the tricarboxylic acid (TCA) cycle. Researchers utilize TMA to study metabolic pathways and cellular respiration due to its stability compared to free aconitic acid .
3.2. Antimicrobial Properties
Recent studies have indicated that aconitic acid derivatives, including TMA, exhibit antimicrobial properties against various pathogens, suggesting potential applications in agricultural and pharmaceutical sectors .
Case Studies
Case Study 1: Green Chemistry Applications
A study demonstrated the use of TMA in green chemistry by employing it in aza-Michael reactions under environmentally friendly conditions (low temperatures and methanol as solvent). The results showed rapid conversion and high yields, highlighting TMA's potential as a sustainable chemical .
Case Study 2: Biopolymer Development
Research involving TMA as a cross-linking agent led to the development of polyesters that mimic extracellular matrices for tissue engineering applications. These materials showed promise for supporting cell growth and tissue regeneration .
Vergleich Mit ähnlichen Verbindungen
Trimethyl aconitate can be compared with other similar compounds, such as:
Biologische Aktivität
Trimethyl aconitate, an ester derivative of aconitic acid, has garnered attention in recent years for its potential biological activities. This compound is characterized by three methoxy groups attached to the aconitic acid backbone, which contributes to its diverse applications in organic synthesis and potential therapeutic uses. This article explores the biological activity of this compound, including its mechanisms of action, biochemical pathways, and relevant case studies.
Overview of this compound
- Chemical Structure : this compound is represented by the molecular formula CHO and has a molecular weight of 230.22 g/mol.
- Physical Properties : It appears as a white solid with a melting point around -14°C .
This compound is involved in several biochemical pathways, primarily related to the tricarboxylic acid (TCA) cycle:
- Conversion to Itaconate : this compound can be converted into itaconate via the enzyme ACOD1 (inducible nitric oxide synthase), which plays a significant role in immune responses and metabolic regulation.
- Influence on Macrophage Function : Itaconate, derived from this compound, has been shown to modulate macrophage metabolism and enhance their anti-inflammatory responses. This suggests that this compound may influence immune cell function indirectly through its metabolic products .
Antimicrobial Properties
Research indicates that this compound exhibits antimicrobial activity against various pathogens. Its derivatives have been studied for their effectiveness in inhibiting bacterial growth, particularly against strains such as Escherichia coli and Staphylococcus aureus.
Anti-inflammatory Effects
This compound has demonstrated potential anti-inflammatory properties. Studies suggest that it can downregulate pro-inflammatory cytokines and modulate immune responses, making it a candidate for therapeutic applications in inflammatory diseases.
Case Studies
-
Modification of Anti-TNF-α Aptamer :
- A study explored the conjugation of this compound with an anti-TNF-α aptamer. This modification significantly enhanced the aptamer's affinity for TNF-α, leading to improved neutralization of TNF-α activity both in vitro and in vivo. This finding highlights the potential use of this compound in developing targeted therapies for autoimmune diseases.
- Biochemical Pathway Analysis :
Research Findings
The following table summarizes key research findings related to the biological activities of this compound:
Future Directions
Ongoing research is focused on expanding the understanding of this compound's biological activities and exploring its potential applications in medicine:
- Therapeutic Development : Further studies are needed to evaluate its efficacy in clinical settings, particularly regarding its anti-inflammatory properties.
- Synthetic Applications : Given its role as a building block in organic synthesis, exploring new synthetic pathways involving this compound could lead to novel compounds with enhanced biological activities.
Eigenschaften
IUPAC Name |
trimethyl (Z)-prop-1-ene-1,2,3-tricarboxylate | |
---|---|---|
Source | PubChem | |
URL | https://pubchem.ncbi.nlm.nih.gov | |
Description | Data deposited in or computed by PubChem | |
InChI |
InChI=1S/C9H12O6/c1-13-7(10)4-6(9(12)15-3)5-8(11)14-2/h4H,5H2,1-3H3/b6-4- | |
Source | PubChem | |
URL | https://pubchem.ncbi.nlm.nih.gov | |
Description | Data deposited in or computed by PubChem | |
InChI Key |
DZAIBGWGBBQGPZ-XQRVVYSFSA-N | |
Source | PubChem | |
URL | https://pubchem.ncbi.nlm.nih.gov | |
Description | Data deposited in or computed by PubChem | |
Canonical SMILES |
COC(=O)CC(=CC(=O)OC)C(=O)OC | |
Source | PubChem | |
URL | https://pubchem.ncbi.nlm.nih.gov | |
Description | Data deposited in or computed by PubChem | |
Isomeric SMILES |
COC(=O)C/C(=C/C(=O)OC)/C(=O)OC | |
Source | PubChem | |
URL | https://pubchem.ncbi.nlm.nih.gov | |
Description | Data deposited in or computed by PubChem | |
Molecular Formula |
C9H12O6 | |
Source | PubChem | |
URL | https://pubchem.ncbi.nlm.nih.gov | |
Description | Data deposited in or computed by PubChem | |
Molecular Weight |
216.19 g/mol | |
Source | PubChem | |
URL | https://pubchem.ncbi.nlm.nih.gov | |
Description | Data deposited in or computed by PubChem | |
CAS No. |
20820-77-3 | |
Record name | Trimethyl aconitate | |
Source | DTP/NCI | |
URL | https://dtp.cancer.gov/dtpstandard/servlet/dwindex?searchtype=NSC&outputformat=html&searchlist=67343 | |
Description | The NCI Development Therapeutics Program (DTP) provides services and resources to the academic and private-sector research communities worldwide to facilitate the discovery and development of new cancer therapeutic agents. | |
Explanation | Unless otherwise indicated, all text within NCI products is free of copyright and may be reused without our permission. Credit the National Cancer Institute as the source. | |
Retrosynthesis Analysis
AI-Powered Synthesis Planning: Our tool employs the Template_relevance Pistachio, Template_relevance Bkms_metabolic, Template_relevance Pistachio_ringbreaker, Template_relevance Reaxys, Template_relevance Reaxys_biocatalysis model, leveraging a vast database of chemical reactions to predict feasible synthetic routes.
One-Step Synthesis Focus: Specifically designed for one-step synthesis, it provides concise and direct routes for your target compounds, streamlining the synthesis process.
Accurate Predictions: Utilizing the extensive PISTACHIO, BKMS_METABOLIC, PISTACHIO_RINGBREAKER, REAXYS, REAXYS_BIOCATALYSIS database, our tool offers high-accuracy predictions, reflecting the latest in chemical research and data.
Strategy Settings
Precursor scoring | Relevance Heuristic |
---|---|
Min. plausibility | 0.01 |
Model | Template_relevance |
Template Set | Pistachio/Bkms_metabolic/Pistachio_ringbreaker/Reaxys/Reaxys_biocatalysis |
Top-N result to add to graph | 6 |
Feasible Synthetic Routes
Q1: What makes trimethyl aconitate a promising building block in polymer synthesis?
A: this compound possesses a high density of functional groups, making it attractive for polymerization reactions. For instance, it readily undergoes aza-Michael addition with diamines, forming tetra-functional N-alkyl-bis-(pyrrolidone dimethylcarboxylate) monomers. These monomers exhibit excellent thermal stability, proving valuable for melt-polycondensation reactions, ultimately yielding polymers with tunable properties. Furthermore, its copolymerization with butadiene, styrene, acrylonitrile, and vinyl chloride has been studied. These studies explored its reactivity ratios and the resulting copolymer properties, demonstrating its versatility in material science.
Q2: How does this compound contribute to greener synthetic approaches in organic chemistry?
A: this compound can be derived from renewable sources, aligning with green chemistry principles. It participates in efficient domino reactions, such as those involving imine additions and intramolecular acylations, offering a concise route to heteropolycycles in one-pot reactions. This highlights its potential to streamline synthesis while minimizing environmental impact.
Q3: Can this compound be electrochemically modified, and what are the implications?
A: Yes, this compound undergoes stereoselective cathodic cyclodimerization, producing a cyclic hexamethyl ester via inter- and intramolecular Michael addition. This electrochemical transformation exemplifies how renewable feedstocks can be converted into valuable compounds using sustainable methods.
Q4: Has this compound been explored for biological applications?
A: While not extensively studied for direct biological activity, a recent study explored a novel application of this compound in modifying an existing anti-TNF-α aptamer. Researchers conjugated three units of the aptamer to a this compound core, creating a trimeric structure. This modification significantly enhanced the aptamer's affinity for TNF-α, leading to improved neutralization of TNF-α activity both in vitro and in vivo.
Q5: What analytical techniques are commonly used to characterize this compound and its derivatives?
A: Gas chromatography has been employed to analyze the pyrazoline derivative of this compound. This technique allows for the separation and quantification of the derivative, providing insights into its purity and potential applications. In the context of the anti-TNF-α aptamer modification, gel electrophoresis played a crucial role in confirming the formation of the trimeric aptamer structure linked by this compound.
Haftungsausschluss und Informationen zu In-Vitro-Forschungsprodukten
Bitte beachten Sie, dass alle Artikel und Produktinformationen, die auf BenchChem präsentiert werden, ausschließlich zu Informationszwecken bestimmt sind. Die auf BenchChem zum Kauf angebotenen Produkte sind speziell für In-vitro-Studien konzipiert, die außerhalb lebender Organismen durchgeführt werden. In-vitro-Studien, abgeleitet von dem lateinischen Begriff "in Glas", beinhalten Experimente, die in kontrollierten Laborumgebungen unter Verwendung von Zellen oder Geweben durchgeführt werden. Es ist wichtig zu beachten, dass diese Produkte nicht als Arzneimittel oder Medikamente eingestuft sind und keine Zulassung der FDA für die Vorbeugung, Behandlung oder Heilung von medizinischen Zuständen, Beschwerden oder Krankheiten erhalten haben. Wir müssen betonen, dass jede Form der körperlichen Einführung dieser Produkte in Menschen oder Tiere gesetzlich strikt untersagt ist. Es ist unerlässlich, sich an diese Richtlinien zu halten, um die Einhaltung rechtlicher und ethischer Standards in Forschung und Experiment zu gewährleisten.