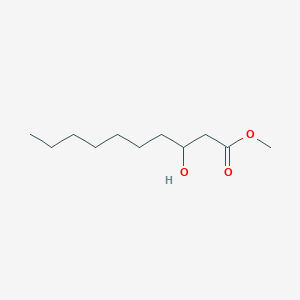
Methyl 3-hydroxydecanoate
Übersicht
Beschreibung
Methyl 3-hydroxydecanoate is a chemical compound that belongs to the class of organic compounds known as fatty acid methyl esters. These are derived from fatty acids, which are important components of lipids and play a crucial role in various biological processes. This compound, in particular, is a hydroxy fatty acid ester that has been studied for its potential applications in various fields, including the synthesis of bioplastics, pharmaceuticals, and as a component in fragrances and flavors.
Synthesis Analysis
The synthesis of this compound can be achieved through various methods. One approach involves the hydrolytic degradation of polyhydroxyalkanoate copolymers synthesized by bacteria such as Pseudomonas putida. The polymer is isolated, hydrolyzed by acid methanolysis, and the resulting methylester mixture is distilled to obtain this compound with high purity . Another method for synthesizing hydroxy fatty acid esters involves the use of engineered strains of Candida tropicalis for fermentation processes .
Molecular Structure Analysis
The molecular structure of this compound consists of a hydrocarbon chain with ten carbon atoms, a methyl ester group at one end, and a hydroxyl group at the third carbon atom. This structure imparts unique physical and chemical properties to the compound, which can be further analyzed through techniques such as gas chromatography-mass spectrometry (GC-MS) .
Chemical Reactions Analysis
This compound can undergo various chemical reactions due to the presence of both ester and hydroxyl functional groups. It can be saponified to yield the corresponding 3-hydroxyalkanoic acid, which can be used for further chemical transformations . The hydroxyl group also allows for reactions such as esterification, oxidation, and the formation of derivatives that can be used in the synthesis of complex molecules .
Physical and Chemical Properties Analysis
The physical and chemical properties of this compound are influenced by its molecular weight and the presence of functional groups. The compound exhibits specific melting and boiling points, solubility characteristics, and reactivity profiles that are relevant to its applications. For instance, the mechanical and thermal properties of polymers derived from hydroxy fatty acid esters can be compared to those of conventional plastics, such as high-density polyethylene, to evaluate their potential as biodegradable alternatives .
Wissenschaftliche Forschungsanwendungen
Chemical Analysis Techniques
Methyl 3-hydroxydecanoate and related hydroxy fatty acids have been studied for their role in various chemical analysis techniques. For instance, 3-hydroxytetradecanoic and 3-hydroxyhexadecanoic acids have been used as chemical markers in gas chromatography-mass spectrometry for the determination of lipopolysaccharides. These methods are beneficial for the characterization of lipopolysaccharides in different environments (Mielniczuk et al., 1992).
Synthesis Techniques
The compound has also been involved in the synthesis of various chemical structures. For instance, a study described a versatile route for the synthesis of (R)-3-hydroxyalkanoic acids, including (R)-3-hydroxydecanoic acid. This synthesis involved microwave-assisted catalytic transfer hydrogenation and microwave-assisted hydrolysis of N-methoxy-N-methyl (Weinreb) amide, enhancing the practicality of this protecting group for carboxylic acids (Jaipuri et al., 2004).
Biocompatibility and Tissue Engineering
In the context of biocompatibility and tissue engineering, studies have investigated the cellular responses to polyhydroxyalkanoates (PHA) degradation products, including oligo-hydroxyalkanoates. These studies are crucial for understanding the biocompatibility of PHA polymers in tissue-engineering applications. For instance, medium-chain-length oligo(3-hydroxyalkanoates), which contain 3-hydroxydecanoate, have been proposed as preferred materials for PHA-based tissue-engineering biomaterials due to their reduced cytotoxicity (Sun et al., 2007).
Safety and Hazards
In case of accidental release, avoid dust formation, breathing mist, gas or vapours, and contact with skin and eye . Use personal protective equipment, wear chemical impermeable gloves, ensure adequate ventilation, remove all sources of ignition, and evacuate personnel to safe areas . Keep people away from and upwind of spill/leak . In case of ingestion of large amounts, consult a doctor/physician .
Zukünftige Richtungen
Wirkmechanismus
Target of Action
Methyl 3-hydroxydecanoate is a biochemical reagent that can be used as a biological material or organic compound for life science related research . .
Mode of Action
As a biochemical reagent, it may interact with various biological targets, but the specifics of these interactions are currently unknown .
Pharmacokinetics
Therefore, its impact on bioavailability is currently unknown .
Action Environment
Like many biochemical reagents, its activity may be influenced by factors such as temperature, ph, and the presence of other chemicals .
Eigenschaften
IUPAC Name |
methyl 3-hydroxydecanoate | |
---|---|---|
Source | PubChem | |
URL | https://pubchem.ncbi.nlm.nih.gov | |
Description | Data deposited in or computed by PubChem | |
InChI |
InChI=1S/C11H22O3/c1-3-4-5-6-7-8-10(12)9-11(13)14-2/h10,12H,3-9H2,1-2H3 | |
Source | PubChem | |
URL | https://pubchem.ncbi.nlm.nih.gov | |
Description | Data deposited in or computed by PubChem | |
InChI Key |
UBVACUZVNTVHTE-UHFFFAOYSA-N | |
Source | PubChem | |
URL | https://pubchem.ncbi.nlm.nih.gov | |
Description | Data deposited in or computed by PubChem | |
Canonical SMILES |
CCCCCCCC(CC(=O)OC)O | |
Source | PubChem | |
URL | https://pubchem.ncbi.nlm.nih.gov | |
Description | Data deposited in or computed by PubChem | |
Molecular Formula |
C11H22O3 | |
Source | PubChem | |
URL | https://pubchem.ncbi.nlm.nih.gov | |
Description | Data deposited in or computed by PubChem | |
DSSTOX Substance ID |
DTXSID90334720 | |
Record name | Methyl 3-hydroxydecanoate | |
Source | EPA DSSTox | |
URL | https://comptox.epa.gov/dashboard/DTXSID90334720 | |
Description | DSSTox provides a high quality public chemistry resource for supporting improved predictive toxicology. | |
Molecular Weight |
202.29 g/mol | |
Source | PubChem | |
URL | https://pubchem.ncbi.nlm.nih.gov | |
Description | Data deposited in or computed by PubChem | |
CAS RN |
62675-82-5 | |
Record name | Methyl 3-hydroxydecanoate | |
Source | EPA DSSTox | |
URL | https://comptox.epa.gov/dashboard/DTXSID90334720 | |
Description | DSSTox provides a high quality public chemistry resource for supporting improved predictive toxicology. | |
Synthesis routes and methods
Procedure details
Retrosynthesis Analysis
AI-Powered Synthesis Planning: Our tool employs the Template_relevance Pistachio, Template_relevance Bkms_metabolic, Template_relevance Pistachio_ringbreaker, Template_relevance Reaxys, Template_relevance Reaxys_biocatalysis model, leveraging a vast database of chemical reactions to predict feasible synthetic routes.
One-Step Synthesis Focus: Specifically designed for one-step synthesis, it provides concise and direct routes for your target compounds, streamlining the synthesis process.
Accurate Predictions: Utilizing the extensive PISTACHIO, BKMS_METABOLIC, PISTACHIO_RINGBREAKER, REAXYS, REAXYS_BIOCATALYSIS database, our tool offers high-accuracy predictions, reflecting the latest in chemical research and data.
Strategy Settings
Precursor scoring | Relevance Heuristic |
---|---|
Min. plausibility | 0.01 |
Model | Template_relevance |
Template Set | Pistachio/Bkms_metabolic/Pistachio_ringbreaker/Reaxys/Reaxys_biocatalysis |
Top-N result to add to graph | 6 |
Feasible Synthetic Routes
Q & A
Q1: Why is Methyl 3-hydroxydecanoate of interest in carbohydrate synthesis?
A1: this compound serves as a valuable starting material for synthesizing complex molecules, particularly in the context of carbohydrate chemistry. For instance, it plays a crucial role in the synthesis of Rhamnolipids, specifically Rhamnolipid B. Rhamnolipids are glycolipids known for their biological activities, including antibacterial and antiviral properties. [, ]
Q2: Can you explain the role of stereochemistry in the synthesis of Rhamnolipid B using this compound?
A2: The stereochemistry of this compound is crucial when synthesizing Rhamnolipid B. Research has shown that the (R)-enantiomer of this compound leads to the formation of diastereoisomeric rhamnosides when reacted with L-rhamnose peracetate. [] Furthermore, in the final step of Rhamnolipid B synthesis, the stereochemistry of this compound derivative determines the configuration of the final product. Specifically, using a derivative of (R)-Methyl 3-hydroxydecanoate results in the attachment of the β-hydroxydecanoic acid moiety in an α-configuration to the dirhamnose sugar. [] This highlights the importance of stereochemical control in the synthesis of bioactive glycolipids like Rhamnolipid B.
Q3: What are the advantages of using minimally competent Lewis acids like Indium(III) bromide and Bismuth(III) triflate in rhamnoside synthesis with this compound?
A3: Minimally competent Lewis acids, such as Indium(III) bromide (InBr3) and Bismuth(III) triflate (Bi(OTf)3), offer distinct advantages over stronger Lewis acids in synthesizing rhamnosides using this compound. While stronger acids like BF3⋅Et2O and Sc(OTf)3 can promote glycosylation, they often necessitate excess reagents and stringent reaction control to minimize impurities. In contrast, InBr3 and Bi(OTf)3 demonstrate high catalytic efficiency even at low concentrations (<10%), produce purer rhamnoside products, and tolerate a wider range of reaction conditions, including higher temperatures. [] This makes them desirable alternatives for this specific glycosylation reaction.
Haftungsausschluss und Informationen zu In-Vitro-Forschungsprodukten
Bitte beachten Sie, dass alle Artikel und Produktinformationen, die auf BenchChem präsentiert werden, ausschließlich zu Informationszwecken bestimmt sind. Die auf BenchChem zum Kauf angebotenen Produkte sind speziell für In-vitro-Studien konzipiert, die außerhalb lebender Organismen durchgeführt werden. In-vitro-Studien, abgeleitet von dem lateinischen Begriff "in Glas", beinhalten Experimente, die in kontrollierten Laborumgebungen unter Verwendung von Zellen oder Geweben durchgeführt werden. Es ist wichtig zu beachten, dass diese Produkte nicht als Arzneimittel oder Medikamente eingestuft sind und keine Zulassung der FDA für die Vorbeugung, Behandlung oder Heilung von medizinischen Zuständen, Beschwerden oder Krankheiten erhalten haben. Wir müssen betonen, dass jede Form der körperlichen Einführung dieser Produkte in Menschen oder Tiere gesetzlich strikt untersagt ist. Es ist unerlässlich, sich an diese Richtlinien zu halten, um die Einhaltung rechtlicher und ethischer Standards in Forschung und Experiment zu gewährleisten.