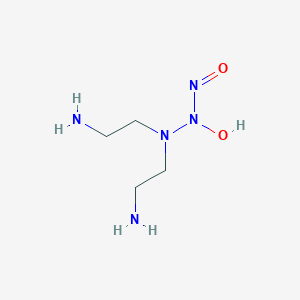
DETA NONOate
- Klicken Sie auf QUICK INQUIRY, um ein Angebot von unserem Expertenteam zu erhalten.
- Mit qualitativ hochwertigen Produkten zu einem WETTBEWERBSFÄHIGEN Preis können Sie sich mehr auf Ihre Forschung konzentrieren.
Übersicht
Beschreibung
DETA NONOat, auch bekannt als Diethylentriamin NONOat, ist eine Stickstoffoxid-Donorverbindung. Es wird in der wissenschaftlichen Forschung häufig eingesetzt, da es Stickstoffoxid kontrolliert freisetzen kann. Stickstoffoxid ist ein wichtiges Signalmolekül, das an verschiedenen physiologischen und pathologischen Prozessen beteiligt ist, darunter Vasodilatation, Neurotransmission und Immunantwort .
Vorbereitungsmethoden
Synthetische Wege und Reaktionsbedingungen: DETA NONOat wird durch Reaktion von Diethylentriamin mit Stickstoffoxid unter kontrollierten Bedingungen synthetisiert. Die Reaktion beinhaltet typischerweise das Einleiten von Stickstoffoxidgas in eine Lösung von Diethylentriamin in einem geeigneten Lösungsmittel wie Ethanol oder Wasser bei niedrigen Temperaturen. Das resultierende Produkt wird dann durch Kristallisation oder andere geeignete Verfahren gereinigt .
Industrielle Produktionsmethoden: Die industrielle Produktion von DETA NONOat folgt ähnlichen Prinzipien, jedoch in größerem Maßstab. Das Verfahren beinhaltet die Verwendung von Diethylentriamin in Industriequalität und Stickstoffoxidgas. Die Reaktion wird in großen Reaktoren mit präziser Kontrolle von Temperatur und Druck durchgeführt, um eine gleichbleibende Produktqualität zu gewährleisten. Das Endprodukt wird gereinigt und zum Vertrieb verpackt .
Analyse Chemischer Reaktionen
Reaktionstypen: DETA NONOat zerfällt hauptsächlich unter Freisetzung von Stickstoffoxid. Dieser Zerfall ist pH-abhängig und folgt einem kinetischen Prozess erster Ordnung. Bei physiologischem pH-Wert (7,4) beträgt die Halbwertszeit von DETA NONOat etwa 20 Stunden bei 37 °C .
Häufige Reagenzien und Bedingungen: Für den Zerfall von DETA NONOat werden keine spezifischen Reagenzien benötigt. die Geschwindigkeit der Stickstoffoxidfreisetzung kann jedoch durch Faktoren wie Temperatur, pH-Wert und das Vorhandensein anderer reaktiver Spezies beeinflusst werden .
Geformte Hauptprodukte: Das bei der Zersetzung von DETA NONOat entstehende Hauptprodukt ist Stickstoffoxid. Zusätzlich entsteht bei der Zersetzung Diethylentriamin als Nebenprodukt .
Wissenschaftliche Forschungsanwendungen
DETA NONOat hat ein breites Anwendungsspektrum in der wissenschaftlichen Forschung:
Medizin: Es wird in präklinischen Studien verwendet, um das therapeutische Potenzial von Stickstoffoxid bei Erkrankungen wie Herz-Kreislauf-Erkrankungen, Krebs und neurodegenerativen Erkrankungen zu untersuchen
5. Wirkmechanismus
DETA NONOat entfaltet seine Wirkung durch die Freisetzung von Stickstoffoxid, das dann mit verschiedenen molekularen Zielstrukturen und Signalwegen interagiert. Stickstoffoxid aktiviert das Enzym Guanylatcyclase, was zur Produktion von cyclischem Guanosinmonophosphat (cGMP) führt. Dieses Signalmolekül vermittelt verschiedene physiologische Reaktionen, darunter Vasodilatation, Hemmung der Thrombozytenaggregation und Modulation der Neurotransmission .
Ähnliche Verbindungen:
- PAPA NONOat (Propylamin-Propylamin NONOat)
- JS-K (O-(2,4-Dinitrophenyl)-1-[(4-Ethoxycarbonyl)piperazin-1-yl]diazen-1-ium-1,2-diolat)
Vergleich: DETA NONOat ist aufgrund seiner relativ langen Halbwertszeit und kontrollierten Freisetzung von Stickstoffoxid einzigartig. Dies macht es besonders nützlich für Studien, die eine anhaltende Stickstoffoxidabgabe erfordern. Im Gegensatz dazu haben andere Stickstoffoxid-Donoren wie PAPA NONOat und JS-K eine andere Freisetzungskinetik und sind möglicherweise besser für Anwendungen geeignet, die eine schnelle oder kurzfristige Stickstoffoxidfreisetzung erfordern .
Wirkmechanismus
DETA NONOate exerts its effects by releasing nitric oxide, which then interacts with various molecular targets and pathways. Nitric oxide activates the enzyme guanylate cyclase, leading to the production of cyclic guanosine monophosphate (cGMP). This signaling molecule mediates various physiological responses, including vasodilation, inhibition of platelet aggregation, and modulation of neurotransmission .
Vergleich Mit ähnlichen Verbindungen
- PAPA NONOate (propylamine propylamine NONOate)
- JS-K (O-(2,4-dinitrophenyl)-1-[(4-ethoxycarbonyl)piperazin-1-yl]diazen-1-ium-1,2-diolate)
Comparison: DETA NONOate is unique due to its relatively long half-life and controlled release of nitric oxide. This makes it particularly useful for studies requiring sustained nitric oxide delivery. In contrast, other nitric oxide donors like PAPA NONOate and JS-K have different release kinetics and may be more suitable for applications requiring rapid or short-term nitric oxide release .
Biologische Aktivität
DETA NONOate, a diazeniumdiolate compound, is recognized for its ability to release nitric oxide (NO) in a controlled manner. This property has made it a significant subject of research in various biological contexts, particularly in vascular biology, neurobiology, and cancer research. This article explores the biological activity of this compound, highlighting its mechanisms of action, therapeutic potentials, and relevant case studies.
This compound acts primarily as a NO donor, generating NO upon decomposition. The biological effects of NO are mediated through several pathways:
- Vasodilation : this compound induces relaxation of vascular smooth muscle cells (VSMCs) by increasing intracellular cGMP levels, leading to vasodilation. This effect is crucial in managing conditions like pulmonary hypertension and atherosclerosis .
- Oxidative Stress Modulation : It has been shown to inhibit the production of reactive oxygen species (ROS), which are implicated in various cardiovascular diseases. This compound can block the activity of NADPH oxidase, thereby reducing oxidative stress .
- Cell Cycle Regulation : In cancer cells, this compound has been observed to induce cytostasis by down-regulating cyclin D1, leading to cell cycle arrest at the G1 phase. This mechanism highlights its potential as an anti-cancer agent .
Biological Activities
The diverse biological activities of this compound can be summarized as follows:
1. Vascular Protective Activity
A study demonstrated that this compound significantly inhibited superoxide production in VSMCs, indicating its protective role against oxidative damage in vascular tissues. This suggests its potential use in therapies for atherosclerosis and other cardiovascular diseases .
2. Cancer Research
In human tumor cell lines, this compound was shown to cause cell cycle arrest by down-regulating cyclin D1 expression. This effect was concentration-dependent and highlights the compound's potential as a therapeutic agent in cancer treatment .
3. Neurobiology
Research indicated that this compound could activate cation-selective channels in cerebellar granule neurons, leading to inward currents that are independent of NO release. This finding points to unique properties of this compound that could be leveraged for neuroprotective strategies .
Eigenschaften
CAS-Nummer |
146724-94-9 |
---|---|
Molekularformel |
C4H13N5O2 |
Molekulargewicht |
163.18 g/mol |
IUPAC-Name |
(E)-[bis(2-aminoethyl)amino]-hydroxyimino-oxidoazanium |
InChI |
InChI=1S/C4H13N5O2/c5-1-3-8(4-2-6)9(11)7-10/h10H,1-6H2/b9-7+ |
InChI-Schlüssel |
YNRCBOXEDICOIX-VQHVLOKHSA-N |
SMILES |
C(CN(CCN)[N+](=NO)[O-])N |
Isomerische SMILES |
C(CN(CCN)/[N+](=N\O)/[O-])N |
Kanonische SMILES |
C(CN(CCN)[N+](=NO)[O-])N |
Aussehen |
Assay:≥98%A crystalline solid |
Synonyme |
Diethylenetriamine NONOate; NOC-18 |
Herkunft des Produkts |
United States |
Retrosynthesis Analysis
AI-Powered Synthesis Planning: Our tool employs the Template_relevance Pistachio, Template_relevance Bkms_metabolic, Template_relevance Pistachio_ringbreaker, Template_relevance Reaxys, Template_relevance Reaxys_biocatalysis model, leveraging a vast database of chemical reactions to predict feasible synthetic routes.
One-Step Synthesis Focus: Specifically designed for one-step synthesis, it provides concise and direct routes for your target compounds, streamlining the synthesis process.
Accurate Predictions: Utilizing the extensive PISTACHIO, BKMS_METABOLIC, PISTACHIO_RINGBREAKER, REAXYS, REAXYS_BIOCATALYSIS database, our tool offers high-accuracy predictions, reflecting the latest in chemical research and data.
Strategy Settings
Precursor scoring | Relevance Heuristic |
---|---|
Min. plausibility | 0.01 |
Model | Template_relevance |
Template Set | Pistachio/Bkms_metabolic/Pistachio_ringbreaker/Reaxys/Reaxys_biocatalysis |
Top-N result to add to graph | 6 |
Feasible Synthetic Routes
Haftungsausschluss und Informationen zu In-Vitro-Forschungsprodukten
Bitte beachten Sie, dass alle Artikel und Produktinformationen, die auf BenchChem präsentiert werden, ausschließlich zu Informationszwecken bestimmt sind. Die auf BenchChem zum Kauf angebotenen Produkte sind speziell für In-vitro-Studien konzipiert, die außerhalb lebender Organismen durchgeführt werden. In-vitro-Studien, abgeleitet von dem lateinischen Begriff "in Glas", beinhalten Experimente, die in kontrollierten Laborumgebungen unter Verwendung von Zellen oder Geweben durchgeführt werden. Es ist wichtig zu beachten, dass diese Produkte nicht als Arzneimittel oder Medikamente eingestuft sind und keine Zulassung der FDA für die Vorbeugung, Behandlung oder Heilung von medizinischen Zuständen, Beschwerden oder Krankheiten erhalten haben. Wir müssen betonen, dass jede Form der körperlichen Einführung dieser Produkte in Menschen oder Tiere gesetzlich strikt untersagt ist. Es ist unerlässlich, sich an diese Richtlinien zu halten, um die Einhaltung rechtlicher und ethischer Standards in Forschung und Experiment zu gewährleisten.