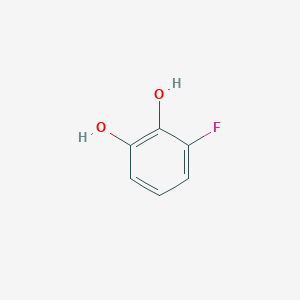
3-Fluorocatechol
Übersicht
Beschreibung
3-Fluorocatechol, also known as 3-fluoro-1,2-benzoquinone, is a chemical compound belonging to the family of quinones. It is a colorless, crystalline solid that is soluble in organic solvents and has a melting point of 122°C. This compound is a versatile compound with numerous applications in scientific research. It is used in the synthesis of various compounds, as well as in the study of biochemical and physiological effects.
Wissenschaftliche Forschungsanwendungen
Biodegradation and Environmental Applications
Biodegradation of Fluorinated Aromatic Compounds : Research indicates that certain bacteria, like Rhizobium sp. and Burkholderia fungorum, can degrade fluorinated aromatic compounds, including 3-fluorocatechol, as part of their metabolic processes. These findings suggest potential applications in bioremediation of environmental contaminants (Zhao et al., 2019); (Strunk & Engesser, 2013).
Ectomycorrhizal Fungi and Mono-Fluorophenols Degradation : Studies show that ectomycorrhizal fungi like Pisolithus tinctorius can degrade mono-fluorophenols, including this compound, in co-metabolism with glucose, highlighting their role in soil ecosystems and potential in environmental biotechnology (Franco et al., 2014).
Biochemical Research and Industrial Applications
NMR Studies for Understanding Biodegradation : Fluorine NMR (19F NMR) is utilized to study the biodegradation pathways of fluorinated compounds like 3-fluorobenzoate, leading to the formation of intermediates such as this compound. This method provides insights into the biochemical pathways in microorganisms (Boersma et al., 2004).
Synthesis and Characterization of Fluorinated Compounds : Synthesis methods for fluorinated catechols, including this compound, are important in pharmaceutical and agrochemical research, offering pathways for creating novel compounds with potential applications in these industries (Yong, 2006).
Biotechnological and Analytical Applications
Enzymatic Transformation in Bacterial Systems : Escherichia coli expressing specific enzymes can transform halogenated phenols into halocatechols, including 4-fluorocatechol, indicating potential biotechnological applications in producing specific halogenated compounds (Coulombel et al., 2011).
Fluorescence and Chromogenic Probes in Biological Research : Compounds like 3-hydroxyphenylacetylene, related to catechol chemistry, serve as probes for bacterial enzymes, assisting in the study of bacterial degradation pathways, which could extend to research involving this compound (Kauffman et al., 2003).
Safety and Hazards
Wirkmechanismus
Target of Action
3-Fluorocatechol, also known as 1,2-Dihydroxy-3-fluorobenzene , is a fluorinated catechol . The primary targets of this compound are enzymes involved in the degradation of halobenzenes, such as catechol 1,2-dioxygenase .
Mode of Action
The interaction of this compound with its targets involves two common steps :
These interactions result in changes to the structure of the compound, facilitating its further metabolism .
Biochemical Pathways
The degradation of this compound proceeds via the formation of 2-fluoromuconic acid . This is a significant finding as 2-fluoromuconic acid was previously considered a dead-end metabolite in fluoroaromatic degradation .
Pharmacokinetics
The strength of the carbon-fluorine bond in fluorinated compounds like this compound generally confers stability, suggesting that they may be recalcitrant in the environment .
Result of Action
The complete metabolism of this compound via 2-fluoromuconate represents a significant advancement in our understanding of fluoroaromatic degradation . This process allows for the effective breakdown of this compound, preventing the accumulation of potentially harmful metabolites .
Action Environment
Environmental factors can influence the action, efficacy, and stability of this compound. For instance, the presence of specific microorganisms capable of metabolizing this compound is crucial for its degradation . Additionally, the physical and chemical properties of the environment, such as pH and temperature, may also impact the compound’s stability and the efficiency of its metabolism .
Biochemische Analyse
Biochemical Properties
3-Fluorocatechol interacts with various enzymes and proteins in biochemical reactions . It is a central metabolite in the degradation pathway of fluorobenzene, proceeding via this compound and productive use of 2-fluoromuconate . The interactions of this compound with these biomolecules are crucial for its role in these biochemical reactions .
Cellular Effects
The effects of this compound on cells and cellular processes are complex and multifaceted. It influences cell function by interacting with various cell signaling pathways, gene expression, and cellular metabolism
Molecular Mechanism
At the molecular level, this compound exerts its effects through binding interactions with biomolecules, enzyme inhibition or activation, and changes in gene expression . The exact mechanism of action of this compound is complex and involves multiple steps, including the formation of this compound and then 2-fluoro-cis-cis-muconate .
Temporal Effects in Laboratory Settings
In laboratory settings, the effects of this compound change over time. It has been observed that high concentrations of this compound can have negative effects on the activity of catechol 1,2-dioxygenase
Dosage Effects in Animal Models
The effects of this compound vary with different dosages in animal models . While specific studies on this compound are limited, it is known that fluorinated compounds can have threshold effects and can cause toxic or adverse effects at high doses .
Metabolic Pathways
This compound is involved in several metabolic pathways. It is a central metabolite in the degradation pathway of fluorobenzene, proceeding via this compound and productive use of 2-fluoromuconate . It interacts with various enzymes and cofactors in these pathways .
Subcellular Localization
Understanding the subcellular localization of this compound could provide insights into its targeting signals or post-translational modifications that direct it to specific compartments or organelles .
Eigenschaften
IUPAC Name |
3-fluorobenzene-1,2-diol | |
---|---|---|
Source | PubChem | |
URL | https://pubchem.ncbi.nlm.nih.gov | |
Description | Data deposited in or computed by PubChem | |
InChI |
InChI=1S/C6H5FO2/c7-4-2-1-3-5(8)6(4)9/h1-3,8-9H | |
Source | PubChem | |
URL | https://pubchem.ncbi.nlm.nih.gov | |
Description | Data deposited in or computed by PubChem | |
InChI Key |
DXOSJQLIRGXWCF-UHFFFAOYSA-N | |
Source | PubChem | |
URL | https://pubchem.ncbi.nlm.nih.gov | |
Description | Data deposited in or computed by PubChem | |
Canonical SMILES |
C1=CC(=C(C(=C1)F)O)O | |
Source | PubChem | |
URL | https://pubchem.ncbi.nlm.nih.gov | |
Description | Data deposited in or computed by PubChem | |
Molecular Formula |
C6H5FO2 | |
Source | PubChem | |
URL | https://pubchem.ncbi.nlm.nih.gov | |
Description | Data deposited in or computed by PubChem | |
DSSTOX Substance ID |
DTXSID50189839 | |
Record name | 1,2-Benzenediol, 3-fluoro- | |
Source | EPA DSSTox | |
URL | https://comptox.epa.gov/dashboard/DTXSID50189839 | |
Description | DSSTox provides a high quality public chemistry resource for supporting improved predictive toxicology. | |
Molecular Weight |
128.10 g/mol | |
Source | PubChem | |
URL | https://pubchem.ncbi.nlm.nih.gov | |
Description | Data deposited in or computed by PubChem | |
CAS RN |
363-52-0 | |
Record name | 3-Fluoro-1,2-benzenediol | |
Source | CAS Common Chemistry | |
URL | https://commonchemistry.cas.org/detail?cas_rn=363-52-0 | |
Description | CAS Common Chemistry is an open community resource for accessing chemical information. Nearly 500,000 chemical substances from CAS REGISTRY cover areas of community interest, including common and frequently regulated chemicals, and those relevant to high school and undergraduate chemistry classes. This chemical information, curated by our expert scientists, is provided in alignment with our mission as a division of the American Chemical Society. | |
Explanation | The data from CAS Common Chemistry is provided under a CC-BY-NC 4.0 license, unless otherwise stated. | |
Record name | 1,2-Benzenediol, 3-fluoro- | |
Source | ChemIDplus | |
URL | https://pubchem.ncbi.nlm.nih.gov/substance/?source=chemidplus&sourceid=0000363520 | |
Description | ChemIDplus is a free, web search system that provides access to the structure and nomenclature authority files used for the identification of chemical substances cited in National Library of Medicine (NLM) databases, including the TOXNET system. | |
Record name | 1,2-Benzenediol, 3-fluoro- | |
Source | EPA DSSTox | |
URL | https://comptox.epa.gov/dashboard/DTXSID50189839 | |
Description | DSSTox provides a high quality public chemistry resource for supporting improved predictive toxicology. | |
Synthesis routes and methods I
Procedure details
Synthesis routes and methods II
Procedure details
Retrosynthesis Analysis
AI-Powered Synthesis Planning: Our tool employs the Template_relevance Pistachio, Template_relevance Bkms_metabolic, Template_relevance Pistachio_ringbreaker, Template_relevance Reaxys, Template_relevance Reaxys_biocatalysis model, leveraging a vast database of chemical reactions to predict feasible synthetic routes.
One-Step Synthesis Focus: Specifically designed for one-step synthesis, it provides concise and direct routes for your target compounds, streamlining the synthesis process.
Accurate Predictions: Utilizing the extensive PISTACHIO, BKMS_METABOLIC, PISTACHIO_RINGBREAKER, REAXYS, REAXYS_BIOCATALYSIS database, our tool offers high-accuracy predictions, reflecting the latest in chemical research and data.
Strategy Settings
Precursor scoring | Relevance Heuristic |
---|---|
Min. plausibility | 0.01 |
Model | Template_relevance |
Template Set | Pistachio/Bkms_metabolic/Pistachio_ringbreaker/Reaxys/Reaxys_biocatalysis |
Top-N result to add to graph | 6 |
Feasible Synthetic Routes
Haftungsausschluss und Informationen zu In-Vitro-Forschungsprodukten
Bitte beachten Sie, dass alle Artikel und Produktinformationen, die auf BenchChem präsentiert werden, ausschließlich zu Informationszwecken bestimmt sind. Die auf BenchChem zum Kauf angebotenen Produkte sind speziell für In-vitro-Studien konzipiert, die außerhalb lebender Organismen durchgeführt werden. In-vitro-Studien, abgeleitet von dem lateinischen Begriff "in Glas", beinhalten Experimente, die in kontrollierten Laborumgebungen unter Verwendung von Zellen oder Geweben durchgeführt werden. Es ist wichtig zu beachten, dass diese Produkte nicht als Arzneimittel oder Medikamente eingestuft sind und keine Zulassung der FDA für die Vorbeugung, Behandlung oder Heilung von medizinischen Zuständen, Beschwerden oder Krankheiten erhalten haben. Wir müssen betonen, dass jede Form der körperlichen Einführung dieser Produkte in Menschen oder Tiere gesetzlich strikt untersagt ist. Es ist unerlässlich, sich an diese Richtlinien zu halten, um die Einhaltung rechtlicher und ethischer Standards in Forschung und Experiment zu gewährleisten.