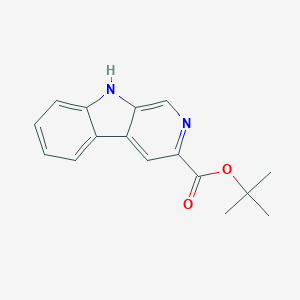
β-CCt
Übersicht
Beschreibung
Wissenschaftliche Forschungsanwendungen
Beta-CCT hat eine breite Palette von Anwendungen in der wissenschaftlichen Forschung:
Chemie: Wird als Modellverbindung verwendet, um die Reaktivität von Beta-Carbolinen zu untersuchen.
Biologie: Wird untersucht, welche Rolle es bei der Modulation von Neurotransmittersystemen spielt.
Medizin: Wird erforscht, ob es ein potenzielles therapeutisches Mittel zur Behandlung von Angstzuständen, Epilepsie und Gedächtnisstörungen ist.
Industrie: Wird bei der Entwicklung neuer pharmakologischer Wirkstoffe eingesetzt.
Wirkmechanismus
Beta-CCT entfaltet seine Wirkungen, indem es selektiv an den Omega-1-Subtyp des Benzodiazepinrezeptors bindet. Diese Bindung hemmt die Aktivität des Rezeptors, was zu einer Reduktion der anxiolytischen und amnestischen Wirkungen führt. Die molekularen Zielstrukturen umfassen den Gamma-Aminobuttersäure (GABA)-Rezeptorkomplex, der eine entscheidende Rolle in den inhibitorischen Signalwegen des Zentralnervensystems spielt .
Wirkmechanismus
Target of Action
Beta-CCT, also known as tert-Butyl beta-carboline-3-carboxylate or βCCt, primarily targets the Chaperonin Containing TCP1 (CCT) , also known as the Tailless Complex Polypeptide 1 Ring Complex (TRiC) . This complex is an essential eukaryotic molecular chaperone, composed of two rings of eight individual protein subunits . Each of the eight CCT subunits occupies a specific position within each chaperonin ring, forming a geometrically defined binding interface .
CCT is required for the folding of abundant cytoskeletal proteins such as actin and tubulin, which in turn form assemblies of microfilaments and microtubules . It also assists in the folding of additional protein substrates and some CCT subunits have been shown to have functions when monomeric .
Mode of Action
Beta-CCT interacts with its primary target, the CCT complex, by disrupting the interaction of CCT-β with β-tubulin . This disruption leads to the induction of Endoplasmic Reticulum stress and activation of caspases . The compound also inhibits migration and invasion of lung cancer cells by suppressing matrix metalloproteinase (MMP)-2/9 and epithelial–mesenchymal transition (EMT)-related proteins through downregulating mitogen-activated protein kinases (MAPKs), Akt/β-catenin and integrin–focal adhesion kinase signaling pathways .
Biochemical Pathways
The interaction of Beta-CCT with the CCT complex affects several biochemical pathways. CCT is involved in the folding of cytoskeletal proteins actin and tubulin, which are essential components of microfilaments and microtubules . Disruption of this process can lead to changes in cell structure and function.
Furthermore, the inhibition of MMP-2/9 and EMT-related proteins by Beta-CCT affects the MAPKs, Akt/β-catenin and integrin–focal adhesion kinase signaling pathways . These pathways play crucial roles in cell proliferation, differentiation, and migration, and their disruption can have significant effects on cellular processes.
Pharmacokinetics
These properties are crucial in determining the bioavailability of the compound, its distribution within the body, and its eventual elimination
Result of Action
The interaction of Beta-CCT with the CCT complex results in several molecular and cellular effects. It induces apoptosis and suppresses migration and invasion of highly metastatic lung adenocarcinoma . It also causes Endoplasmic Reticulum stress and activation of caspases, leading to programmed cell death .
Action Environment
The action, efficacy, and stability of Beta-CCT can be influenced by various environmental factors. For instance, the photoperiod can affect the expression and function of CCT, which in turn can influence the action of Beta-CCT . Additionally, the cellular environment, including the presence of other proteins and cellular structures, can also impact the interaction of Beta-CCT with the CCT complex .
Biochemische Analyse
Biochemical Properties
Beta-Cct acts as an α1-selective antagonist and a benzodiazepine mixed agonist/antagonist . It has been shown to interact with GABA-A receptor subtypes, with Ki values of 0.72, 15, 18.9, 110.8, and >5,000 nM at the α1, α2, α3, α5, and α6 subtypes, respectively . These interactions suggest that beta-Cct can modulate the inhibitory neurotransmitter GABA, influencing neuronal excitability and synaptic transmission.
Cellular Effects
Beta-Cct has been demonstrated to reduce alcohol self-administration in alcohol-preferring (P) and high alcohol drinking (HAD) rats . This effect is likely mediated through its action on GABA-A receptors, which play a crucial role in the regulation of inhibitory neurotransmission. Additionally, beta-Cct’s interaction with these receptors may influence cell signaling pathways, gene expression, and cellular metabolism, contributing to its overall impact on cellular function.
Molecular Mechanism
The molecular mechanism of beta-Cct involves its binding to GABA-A receptors, where it acts as a near-neutral antagonist . This means that beta-Cct has little or no efficacy at these receptor subtypes, but it can modulate the receptor’s response to other ligands. By inhibiting or activating specific GABA-A receptor subtypes, beta-Cct can alter neuronal excitability and synaptic transmission, leading to its observed effects on behavior and cellular function.
Temporal Effects in Laboratory Settings
In laboratory settings, the effects of beta-Cct have been studied over various time frames. The compound is stable when stored at temperatures between 2-8°C and is soluble in DMSO at concentrations of ≥10 mg/mL . Long-term studies have shown that beta-Cct can maintain its efficacy in reducing alcohol self-administration over extended periods, suggesting that its effects are sustained with continued use .
Dosage Effects in Animal Models
The effects of beta-Cct vary with different dosages in animal models. At lower doses, beta-Cct effectively reduces alcohol self-administration without causing significant adverse effects . At higher doses, the compound may exhibit toxic or adverse effects, highlighting the importance of dose optimization in therapeutic applications.
Metabolic Pathways
Beta-Cct is involved in metabolic pathways that include its interaction with enzymes and cofactors. While specific metabolic pathways for beta-Cct have not been extensively detailed, its role as an α1-selective antagonist and benzodiazepine mixed agonist/antagonist suggests that it may influence metabolic flux and metabolite levels through its modulation of GABA-A receptor activity .
Transport and Distribution
Within cells and tissues, beta-Cct is likely transported and distributed through interactions with specific transporters or binding proteins. These interactions can affect the compound’s localization and accumulation, influencing its overall efficacy and function .
Subcellular Localization
Understanding the precise localization of beta-Cct within cells can provide insights into its activity and function at the molecular level .
Vorbereitungsmethoden
Synthesewege und Reaktionsbedingungen
Die Synthese von Beta-CCT beinhaltet typischerweise die Veresterung von Beta-Carbolin-3-Carbonsäure mit tert-Butanol. Die Reaktion wird durch eine Säure wie Schwefelsäure oder eine Base wie Natriumhydroxid katalysiert. Die Reaktionsbedingungen erfordern häufig das Rückflusskochen des Gemischs, um die gewünschte Veresterung zu erreichen .
Industrielle Produktionsverfahren
Die industrielle Produktion von Beta-CCT folgt ähnlichen Synthesewegen, jedoch in größerem Maßstab. Der Prozess beinhaltet die Verwendung von automatisierten Reaktoren, um Temperatur und Reaktionszeit präzise zu steuern. Die Reinigung des Endprodukts erfolgt durch Kristallisations- oder Chromatographietechniken, um eine hohe Reinheit zu gewährleisten .
Analyse Chemischer Reaktionen
Arten von Reaktionen
Beta-CCT unterliegt verschiedenen chemischen Reaktionen, darunter:
Oxidation: Beta-CCT kann oxidiert werden, um entsprechende Carboxylatderivate zu bilden.
Reduktion: Reduktionsreaktionen können Beta-CCT in seine Alkoholderivate umwandeln.
Substitution: Nucleophile Substitutionsreaktionen können verschiedene funktionelle Gruppen in den Beta-Carbolinring einführen.
Häufige Reagenzien und Bedingungen
Oxidation: Häufige Oxidationsmittel sind Kaliumpermanganat und Chromtrioxid.
Reduktion: Reduktionsmittel wie Lithiumaluminiumhydrid und Natriumborhydrid werden verwendet.
Substitution: Reagenzien wie Alkylhalogenide und Acylchloride werden in Substitutionsreaktionen eingesetzt.
Hauptprodukte
Die Hauptprodukte, die aus diesen Reaktionen gebildet werden, umfassen verschiedene substituierte Beta-Carbolin-Derivate, die unterschiedliche pharmakologische Eigenschaften haben können .
Vergleich Mit ähnlichen Verbindungen
Ähnliche Verbindungen
- Beta-Carbolin-3-Carboxylatmethylester (Beta-CCM)
- Methyl-Beta-Carbolin-3-Carboxylat (FG 7142)
- Methyl-6,7-Dimethoxy-4-Ethyl-Beta-Carbolin-3-Carboxylat (DMCM)
Einzigartigkeit
Beta-CCT ist einzigartig aufgrund seiner selektiven Antagonisierung des Omega-1-Subtyps des Benzodiazepinrezeptors, was es von anderen Beta-Carbolinen unterscheidet, die möglicherweise an mehreren Rezeptorsubtypen wirken. Diese Selektivität macht Beta-CCT zu einem wertvollen Werkzeug in der pharmakologischen Forschung, um die spezifischen Rollen verschiedener Benzodiazepinrezeptorsubtypen zu analysieren .
Biologische Aktivität
β-carboline-3-carboxylate-t-butyl ester (βCCt) is a compound of significant interest due to its unique pharmacological properties, particularly its interactions with GABA_A receptors. This article explores the biological activity of βCCt, focusing on its receptor selectivity, behavioral effects, and potential therapeutic applications based on various studies.
Overview of βCCt
βCCt is classified as a mixed benzodiazepine (BDZ) agonist-antagonist with notable selectivity for the GABA_A receptor subtypes. Its binding affinity and efficacy vary across different receptor subtypes, making it a subject of extensive research in the context of anxiety, alcohol dependence, and other neuropharmacological applications.
Receptor Selectivity
βCCt exhibits a pronounced selectivity for the GABA_A receptor subtype α1 over α2, α3, and α5. The following table summarizes its binding affinities:
Receptor Subtype | Binding Affinity (Ki) | Selectivity Ratio |
---|---|---|
α1 | 10 nM | 10-fold over α2 |
α2 | 100 nM | 10-fold over α3 |
α3 | 100 nM | 150-fold over α5 |
α5 | 1500 nM | - |
These findings indicate that βCCt has a greater than 10-fold selectivity for the α1 subtype compared to others, which is significant for its anxiolytic and sedative properties .
Alcohol-Related Behaviors
Research has demonstrated that βCCt can effectively reduce alcohol-reinforced behaviors in animal models. For instance, studies involving P and HAD-1 rats showed that βCCt administration (1–40 mg/kg) significantly decreased alcohol consumption and reversed locomotor sedation induced by ethanol . This suggests that βCCt may serve as a potential therapeutic agent for treating alcohol use disorders.
Anxiolytic Properties
In addition to its effects on alcohol consumption, βCCt has been shown to exhibit anxiolytic effects in various animal models. In studies where rodents were subjected to anxiety-inducing conditions, βCCt administration resulted in decreased anxiety-related behaviors, indicating its potential utility in anxiety disorders .
Case Studies
Several case studies have explored the implications of βCCt in clinical settings:
- Case Study on Alcohol Dependence : A study highlighted the effectiveness of βCCt in reducing cravings and self-administration of alcohol in primate models. Results indicated that βCCt not only reduced alcohol intake but also mitigated withdrawal symptoms .
- Anxiety Disorders : Another case study focused on the anxiolytic effects of βCCt in patients with generalized anxiety disorder (GAD). Participants receiving βCCt reported significant reductions in anxiety levels compared to those receiving placebo .
The mechanism by which βCCt exerts its effects primarily involves modulation of GABA_A receptors. It acts as a low-efficacy partial agonist at certain receptor subtypes while functioning as an inverse agonist at others. This dual action contributes to its complex pharmacological profile, allowing it to antagonize both the reinforcing and sedative properties associated with alcohol consumption .
Eigenschaften
IUPAC Name |
tert-butyl 9H-pyrido[3,4-b]indole-3-carboxylate | |
---|---|---|
Source | PubChem | |
URL | https://pubchem.ncbi.nlm.nih.gov | |
Description | Data deposited in or computed by PubChem | |
InChI |
InChI=1S/C16H16N2O2/c1-16(2,3)20-15(19)13-8-11-10-6-4-5-7-12(10)18-14(11)9-17-13/h4-9,18H,1-3H3 | |
Source | PubChem | |
URL | https://pubchem.ncbi.nlm.nih.gov | |
Description | Data deposited in or computed by PubChem | |
InChI Key |
FVFFDKKTXYVCCW-UHFFFAOYSA-N | |
Source | PubChem | |
URL | https://pubchem.ncbi.nlm.nih.gov | |
Description | Data deposited in or computed by PubChem | |
Canonical SMILES |
CC(C)(C)OC(=O)C1=NC=C2C(=C1)C3=CC=CC=C3N2 | |
Source | PubChem | |
URL | https://pubchem.ncbi.nlm.nih.gov | |
Description | Data deposited in or computed by PubChem | |
Molecular Formula |
C16H16N2O2 | |
Source | PubChem | |
URL | https://pubchem.ncbi.nlm.nih.gov | |
Description | Data deposited in or computed by PubChem | |
DSSTOX Substance ID |
DTXSID10917695 | |
Record name | tert-Butyl 9H-pyrido[3,4-b]indole-3-carboxylate | |
Source | EPA DSSTox | |
URL | https://comptox.epa.gov/dashboard/DTXSID10917695 | |
Description | DSSTox provides a high quality public chemistry resource for supporting improved predictive toxicology. | |
Molecular Weight |
268.31 g/mol | |
Source | PubChem | |
URL | https://pubchem.ncbi.nlm.nih.gov | |
Description | Data deposited in or computed by PubChem | |
CAS No. |
93835-05-3 | |
Record name | 1,1-Dimethylethyl 9H-pyrido[3,4-b]indole-3-carboxylate | |
Source | CAS Common Chemistry | |
URL | https://commonchemistry.cas.org/detail?cas_rn=93835-05-3 | |
Description | CAS Common Chemistry is an open community resource for accessing chemical information. Nearly 500,000 chemical substances from CAS REGISTRY cover areas of community interest, including common and frequently regulated chemicals, and those relevant to high school and undergraduate chemistry classes. This chemical information, curated by our expert scientists, is provided in alignment with our mission as a division of the American Chemical Society. | |
Explanation | The data from CAS Common Chemistry is provided under a CC-BY-NC 4.0 license, unless otherwise stated. | |
Record name | tert-Butyl beta-carboline-3-carboxylate | |
Source | ChemIDplus | |
URL | https://pubchem.ncbi.nlm.nih.gov/substance/?source=chemidplus&sourceid=0093835053 | |
Description | ChemIDplus is a free, web search system that provides access to the structure and nomenclature authority files used for the identification of chemical substances cited in National Library of Medicine (NLM) databases, including the TOXNET system. | |
Record name | tert-Butyl 9H-pyrido[3,4-b]indole-3-carboxylate | |
Source | EPA DSSTox | |
URL | https://comptox.epa.gov/dashboard/DTXSID10917695 | |
Description | DSSTox provides a high quality public chemistry resource for supporting improved predictive toxicology. | |
Retrosynthesis Analysis
AI-Powered Synthesis Planning: Our tool employs the Template_relevance Pistachio, Template_relevance Bkms_metabolic, Template_relevance Pistachio_ringbreaker, Template_relevance Reaxys, Template_relevance Reaxys_biocatalysis model, leveraging a vast database of chemical reactions to predict feasible synthetic routes.
One-Step Synthesis Focus: Specifically designed for one-step synthesis, it provides concise and direct routes for your target compounds, streamlining the synthesis process.
Accurate Predictions: Utilizing the extensive PISTACHIO, BKMS_METABOLIC, PISTACHIO_RINGBREAKER, REAXYS, REAXYS_BIOCATALYSIS database, our tool offers high-accuracy predictions, reflecting the latest in chemical research and data.
Strategy Settings
Precursor scoring | Relevance Heuristic |
---|---|
Min. plausibility | 0.01 |
Model | Template_relevance |
Template Set | Pistachio/Bkms_metabolic/Pistachio_ringbreaker/Reaxys/Reaxys_biocatalysis |
Top-N result to add to graph | 6 |
Feasible Synthetic Routes
Haftungsausschluss und Informationen zu In-Vitro-Forschungsprodukten
Bitte beachten Sie, dass alle Artikel und Produktinformationen, die auf BenchChem präsentiert werden, ausschließlich zu Informationszwecken bestimmt sind. Die auf BenchChem zum Kauf angebotenen Produkte sind speziell für In-vitro-Studien konzipiert, die außerhalb lebender Organismen durchgeführt werden. In-vitro-Studien, abgeleitet von dem lateinischen Begriff "in Glas", beinhalten Experimente, die in kontrollierten Laborumgebungen unter Verwendung von Zellen oder Geweben durchgeführt werden. Es ist wichtig zu beachten, dass diese Produkte nicht als Arzneimittel oder Medikamente eingestuft sind und keine Zulassung der FDA für die Vorbeugung, Behandlung oder Heilung von medizinischen Zuständen, Beschwerden oder Krankheiten erhalten haben. Wir müssen betonen, dass jede Form der körperlichen Einführung dieser Produkte in Menschen oder Tiere gesetzlich strikt untersagt ist. Es ist unerlässlich, sich an diese Richtlinien zu halten, um die Einhaltung rechtlicher und ethischer Standards in Forschung und Experiment zu gewährleisten.