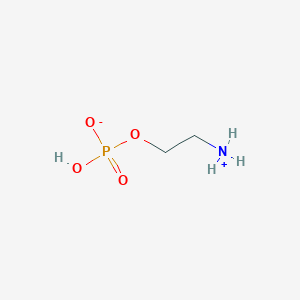
O-Phosphorylethanolamine
Übersicht
Beschreibung
Structure and Biosynthesis: O-Phosphorylethanolamine (PEA, C₂H₈NO₄P, MW 141.06) is a phosphomonoester metabolite comprising an ethanolamine group linked to a phosphate moiety . It serves as a critical precursor for phosphatidylethanolamine (PE), the second-most abundant phospholipid in eukaryotic membranes, and a product of phospholipid catabolism .
Vorbereitungsmethoden
Industrial-Scale Synthesis via Phosphorylation of Ethanolamine
The most widely documented method for PEA synthesis involves the direct phosphorylation of ethanolamine using phosphorus-containing reagents. A patented two-step process (CN101974029B) utilizes water as a solvent, ensuring environmental and economic viability .
Reaction Mechanism and Conditions
The reaction proceeds via nucleophilic attack of ethanolamine’s hydroxyl group on electrophilic phosphorus centers. Key reactants include:
-
Phosphorus pentoxide (P₂O₅)
-
Phosphoric acid (H₃PO₄)
-
Phosphorus oxychloride (POCl₃)
Reagent selection impacts product purity and reaction efficiency. For instance, P₂O₅ achieves a molar ratio of 1:2.0–6.0 relative to ethanolamine, yielding PEA hydrochloride at 94–97% efficiency . Vacuum drying at 0.098 kPa and incremental temperature ramping (80–200°C) removes residual solvents, producing a white solid with ≥99.3% purity .
Table 1: Optimization Parameters for P₂O₅-Based Synthesis
Parameter | Range | Optimal Value |
---|---|---|
Molar ratio (P₂O₅:EtNH₂) | 1:2.0 – 1:6.0 | 1:4.0 |
Reaction temperature | 60–200°C | 160°C |
Vacuum pressure | 0.098 kPa | 0.098 kPa |
Yield | 94–97% | 97% |
Solvent and Environmental Considerations
Unlike traditional methods requiring aromatic hydrocarbons or ketones, this patent employs water, reducing toxic waste . The mass ratio of reactant to water (1:1–100) ensures homogeneity without compromising reaction kinetics. Post-synthesis, the aqueous phase is removed via vacuum drying, circumventing energy-intensive distillation .
Hydrothermal Synthesis for Prebiotic Applications
Prebiotic chemistry studies have demonstrated PEA formation under simulated early-Earth conditions. A hydrothermal approach using glycerol and phosphate minerals at 100–120°C yields PEA alongside other biological phosphates .
Reaction Setup and Catalysis
In a sealed reactor, ethanolamine reacts with phosphate donors (e.g., apatite) in the presence of perlite or clay catalysts. Key factors include:
-
Temperature : 100–120°C
-
Pressure : Autogenous, driven by water vapor
-
Catalyst : Perlite enhances selectivity for PEA over byproducts .
This method mimics geochemical environments, suggesting PEA’s abiotic origin. However, yields remain unquantified in published studies, limiting industrial applicability .
Comparative Analysis of Synthesis Routes
Efficiency and Scalability
Industrial methods prioritize high yields (≥97%) and scalability, whereas hydrothermal synthesis offers academic insights into prebiotic pathways. The table below contrasts key metrics:
Table 2: Method Comparison for PEA Synthesis
Metric | Industrial (P₂O₅) | Hydrothermal |
---|---|---|
Yield | 97% | Not reported |
Purity | ≥99.3% | N/A |
Reaction time | 8–10 hours | Days |
Environmental impact | Low (water solvent) | Negligible |
Challenges and Innovations
-
Byproduct Formation : POCl₃-based reactions risk generating HCl, necessitating neutralization steps .
-
Energy Consumption : Vacuum drying constitutes 60% of energy use in industrial setups .
-
Catalytic Advances : Perlite catalysts in hydrothermal systems reduce side reactions but require optimization for higher throughput .
Validation and Analytical Techniques
Post-synthesis analysis ensures product integrity. Studies employ:
Analyse Chemischer Reaktionen
Reaktionstypen: Phosphoethanolamin durchläuft verschiedene chemische Reaktionen, darunter:
Oxidation: Phosphoethanolamin kann zu Phosphoacetaldehyd oxidiert werden.
Reduktion: Es kann zu Ethanolamin reduziert werden.
Substitution: Phosphoethanolamin kann Substitutionsreaktionen eingehen, bei denen die Aminogruppe durch andere funktionelle Gruppen ersetzt wird.
Häufige Reagenzien und Bedingungen:
Oxidation: Häufige Oxidationsmittel sind Kaliumpermanganat und Wasserstoffperoxid.
Reduktion: Reduktionsmittel wie Natriumborhydrid und Lithiumaluminiumhydrid werden verwendet.
Substitution: Verschiedene Reagenzien, einschließlich Halogenierungsmittel und Nukleophile, werden für Substitutionsreaktionen verwendet.
Hauptprodukte:
Oxidation: Phosphoacetaldehyd.
Reduktion: Ethanolamin.
Substitution: Verschiedene substituierte Phosphoethanolamin-Derivate.
Wissenschaftliche Forschungsanwendungen
Biochemical Research Applications
O-Phosphorylethanolamine serves as a precursor in the biosynthesis of phosphatidylethanolamine (PE), which is crucial for constructing cellular membranes. Its applications in biochemical research include:
- Cell Culture Supplementation : O-PEA is used in LHC-8 medium to culture transformed human liver epithelial cells (THLE-2 and THLE-3). This application supports studies on liver cell functions and diseases .
- Phosphatase Activity Detection : It plays a role in incubating phosphomonoesters with anion-exchange chromatography (AEC) fractions, facilitating the detection of phosphatase activity, which is essential for understanding various metabolic pathways .
- Chromatographic Purification : O-PEA is utilized to prepare phosphorylethanolamine-conjugated Sepharose columns for the chromatographic purification of mutant C-reactive protein (CRP). This application aids in studying protein interactions and functions .
Physiological Implications
This compound is involved in several physiological processes:
- Membrane Dynamics : It is integral to membrane fusion, cell cycle regulation, autophagy, and apoptosis. The presence of O-PEA has been linked to neuronal health, with reduced levels observed in postmortem brain samples from patients with Alzheimer's and Huntington's diseases .
- Neurodegenerative Disease Research : Studies indicate that lower concentrations of O-PEA correlate with increased neuronal death in neurodegenerative conditions, suggesting its potential role as a biomarker for disease progression .
Complexation Studies
Recent research has focused on the acid-base behavior and complexation properties of O-PEA with metal ions such as Mg²⁺. Potentiometric titrations have demonstrated the formation of multiple species under varying conditions, which is crucial for understanding its interactions within biological systems .
Table 1: Complexation Species of this compound
Species | Description | Formation Conditions |
---|---|---|
MLH₂ | Protonated form | Low pH conditions |
MLH | Neutral complex | Moderate pH |
ML | Deprotonated form | High pH |
Potential Therapeutic Applications
While this compound has been investigated for its potential as a cancer treatment, clinical trials have shown no significant benefits, leading to controversy regarding its efficacy. Despite this, ongoing research aims to explore its safety and efficacy further .
Case Study 1: Neurodegenerative Disease
In a study examining postmortem brain samples from Alzheimer's patients, researchers found significantly reduced levels of O-PEA compared to healthy controls. This reduction was associated with increased markers of neuronal death, suggesting a potential role for O-PEA in neuroprotection and as a therapeutic target for neurodegenerative diseases .
Case Study 2: Protein Interaction Studies
In experiments involving mutant CRP purification using O-PEA-conjugated columns, researchers successfully isolated proteins that interacted specifically with CRP. This study highlights the utility of O-PEA in understanding protein dynamics and interactions within cellular processes .
Wirkmechanismus
Phosphoethanolamine exerts its effects through its involvement in the synthesis of phospholipids. It acts as a precursor for the formation of glycerophospholipids and sphingophospholipids, which are essential for maintaining cell membrane integrity and fluidity . The compound interacts with various enzymes and pathways involved in lipid metabolism, influencing cellular processes such as membrane fusion, signaling, and transport .
Vergleich Mit ähnlichen Verbindungen
Comparison with Structurally and Functionally Related Compounds
Phosphatidylethanolamine (PE)
Parameter | PEA | PE |
---|---|---|
Structure | Phosphomonoester (ethanolamine + phosphate) | Diacylglycerol linked to PEA |
Role | Metabolic intermediate | Structural membrane lipid |
Function | Precursor/product of PE synthesis | Membrane curvature, autophagy, apoptosis |
Disease Link | Reduced in neurodegeneration | Altered in cancer and metabolic disorders |
Key Insight : While PEA is a soluble metabolite, PE integrates into membranes, highlighting the interdependence of their pools in lipid homeostasis .
O-Phosphorylcholine (PPC)
Parameter | PEA | PPC |
---|---|---|
Structure | Ethanolamine headgroup | Choline headgroup |
Mg²⁺ Interaction | Forms 4-membered chelate with phosphate | Binds Mg²⁺ via phosphate and choline |
Biological Role | Membrane biosynthesis, neuroprotection | Component of sphingomyelin, platelet-activating factor |
Research Findings :
- PEA and PPC exhibit distinct acid-base behavior and Mg²⁺ binding affinities, influencing their roles in membrane stability and signaling .
- PPC-based biomaterials are used clinically, while PEA’s applications focus on neurodegenerative disease research .
Phosphatidylserine (PS)
Parameter | PEA | PS |
---|---|---|
Head Group | Ethanolamine | Serine |
Localization | Cytoplasmic leaflet (as PE) | Inner membrane leaflet |
Function | Membrane precursor | Apoptosis signaling, blood clotting |
Metabolomic Data :
- In transcriptomic studies, PS showed negative correlations with immune-related genes, whereas PEA correlated with both up- and downregulated pathways .
Ethanolamine
Parameter | PEA | Ethanolamine |
---|---|---|
Phosphorylation | Yes | No |
Metabolic Role | Phospholipid synthesis intermediate | Direct precursor of PEA and choline |
Stress Response | Accumulates under Cd stress in plants | Elevated in overfed animal models |
Key Insight: Ethanolamine and PEA levels are tightly coupled; ethanolamine kinase catalyzes their interconversion, critical for membrane repair under stress .
Phosphorylated Amino Acids (e.g., O-Phosphorylserine)
Parameter | PEA | O-Phosphorylserine |
---|---|---|
Backbone | Ethanolamine | Serine |
Binding Specificity | Binds RKIP in crystal structures | No significant binding to RKIP |
Research Findings :
- PEA’s ethanolamine group confers unique binding to proteins like Raf Kinase Inhibitory Protein (RKIP), unlike phosphorylated amino acids .
Data Tables
Table 1: Structural and Functional Comparison
Compound | Molecular Formula | Key Functional Groups | Primary Role |
---|---|---|---|
PEA | C₂H₈NO₄P | Phosphate, ethanolamine | Phospholipid metabolism |
PPC | C₅H₁₄NO₄P | Phosphate, choline | Membrane signaling |
Ethanolamine | C₂H₇NO | Amino alcohol | PEA precursor |
PS | C₉H₁₈NO₈P | Serine, phosphate | Apoptosis, clotting |
Table 2: Disease-Associated Changes
Compound | Disease Context | Change Observed | Reference |
---|---|---|---|
PEA | Alzheimer’s disease | ↓ in brain tissue | |
PS | Cancer | Altered membrane exposure | |
Ethanolamine | Overfeeding models | ↑ in liver and intestinal content |
Research Highlights
- Metal Interactions : PEA and PPC form distinct Mg²⁺ complexes, influencing their stability in biological fluids .
- Enzyme Substrates : PEA is hydrolyzed by alkaline phosphatase, linking its metabolism to disorders like hypophosphatasia .
- Immune Modulation : PEA-modified lipid A enhances TLR4 agonist activity, suggesting a role in bacterial endotoxin signaling .
Biologische Aktivität
O-Phosphorylethanolamine (PEA) is an ethanolamine derivative that plays a crucial role in the formation of various phospholipids, which are essential components of biological membranes. This article explores the biological activity of PEA, focusing on its chemical properties, interaction with metal ions, potential therapeutic applications, and relevant case studies.
This compound is characterized as a polyprotic acid with two pKa values at 5.61 and 10.39, indicating its ability to donate protons under physiological conditions. The speciation studies conducted on PEA revealed the formation of three distinct species in solution: MLH₂, MLH, and ML, depending on the pH and ionic strength of the environment . These species interact with divalent metal ions such as Mg²⁺, which are critical for various biological functions. The complexation properties were examined through potentiometric titrations in NaCl solutions at varying temperatures (15°C to 37°C) and ionic strengths (0.15 mol L⁻¹ to 1 mol L⁻¹) .
Biological Functions
Membrane Composition : PEA is integral to the structure of phospholipids found in cellular membranes. It is a precursor for both glycerophospholipids and sphingomyelin, contributing to the functional diversity of lipid bilayers . The presence of PEA enhances membrane fluidity and stability, which is vital for cellular processes such as signaling and transport.
Antimicrobial Activity : Recent studies have highlighted the role of PEA in enhancing the antibacterial properties of certain lipopolysaccharides (LPS) by modifying lipid A structures in Gram-negative bacteria. This modification increases the susceptibility of bacteria to host immune responses . For instance, the removal of PEA groups from lipid A significantly reduced bacterial adhesion to epithelial cells, indicating its potential as a target for antimicrobial therapies .
Therapeutic Applications
PEA has been investigated for its potential therapeutic effects, particularly in cancer treatment. However, clinical trials have yielded inconclusive results regarding its efficacy. Research indicated that while PEA was promoted as a cancer treatment in Brazil, it lacked substantial evidence supporting its benefits . Despite this, ongoing studies aim to explore its mechanisms further and evaluate its safety profile.
Case Studies and Research Findings
- Kinetic Characterization : A study characterized the human O-phosphoethanolamine phospho-lyase enzyme, revealing unique kinetic properties that may influence metabolic pathways involving PEA . This enzyme's activity suggests that PEA could play a role in amino acid metabolism and neurotransmitter synthesis.
- Clinical Trials : Clinical trials investigating phosphorylethanolamine as a cancer treatment were halted due to insufficient evidence of benefit. However, legal controversies surrounding its use continue in Brazil, where it was administered without regulatory approval .
- Interaction with Metal Ions : The interaction between PEA and metal ions like Mg²⁺ was studied extensively. These interactions are crucial for maintaining cellular functions and could influence drug delivery systems targeting specific tissues .
Summary Table
Aspect | Details |
---|---|
Chemical Nature | Polyprotic acid; pKa values: 5.61 and 10.39 |
Biological Role | Precursor for phospholipids; enhances membrane stability |
Antimicrobial Function | Modifies lipid A in Gram-negative bacteria; increases susceptibility |
Therapeutic Use | Investigated for cancer treatment; inconclusive clinical trial results |
Metal Ion Interaction | Forms complexes with Mg²⁺; important for biological functions |
Q & A
Basic Research Questions
Q. How can O-Phosphorylethanolamine be quantified in biological samples, and what analytical methods are most reliable?
Quantification typically involves liquid chromatography-mass spectrometry (LC-MS) or nuclear magnetic resonance (NMR) due to its small molecular size and polar nature. For example, metabolomic studies on chronic stress models in rats used LC-MS to detect this compound in hippocampal tissue, correlating its levels with lipidomic perturbations . Standard protocols include derivatization for enhanced sensitivity in LC-MS or using deuterated solvents for NMR signal stabilization.
Q. What experimental approaches are used to study this compound’s role in cell membrane integrity?
Lipidomic profiling combined with fluorescence anisotropy or atomic force microscopy (AFM) can assess membrane fluidity and structural changes. Studies on plant stress responses under cadmium exposure revealed elevated this compound levels, suggesting its role in stabilizing membranes via phosphatidylethanolamine (PE) synthesis . Molecular dynamics simulations further complement these experiments by modeling interactions with phospholipid bilayers .
Q. What are the standard protocols for preparing this compound solutions in cell culture studies?
A 5 mM stock solution is prepared by dissolving 0.705 g of this compound (MW = 141.06) in 100 mL of DMEM, followed by sterile filtration (0.2 μm) and storage at −20 °C. This protocol ensures stability for up to one year and avoids degradation during repeated freeze-thaw cycles .
Advanced Research Questions
Q. How do molecular dynamics simulations elucidate the conformational flexibility of this compound in solvation environments?
Simulations using software like GROMACS or AMBER parameterize solvation effects by modeling hydrogen bonding and dielectric properties. For instance, Woolf and Roux (1994) demonstrated that this compound’s phosphoryl group exhibits dynamic interactions with water molecules, influencing its conformational equilibrium. These studies employ free energy calculations and replica exchange molecular dynamics to sample rare states .
Q. How can metabolomic and lipidomic approaches be integrated to study this compound’s role in stress response pathways?
Multi-omics integration involves correlating this compound levels with lipid species (e.g., phosphatidylcholines, sphingolipids) and pathway enrichment analysis. In chronic social defeat stress models, its association with sphingolipid signaling highlighted cross-talk between phospholipid metabolism and apoptosis regulation. Tools like MetaboAnalyst and LipidSearch facilitate statistical integration .
Q. What enzymatic pathways regulate this compound metabolism, and how can their activity be assayed?
this compound phospho-lyase, a vitamin B6-dependent enzyme, catalyzes its cleavage into ethanolamine and inorganic phosphate. Activity assays involve incubating tissue homogenates with radiolabeled this compound and measuring 14CO2 release. Kinetic parameters (Km, Vmax) are determined via Lineweaver-Burk plots, with inhibitors like hydroxylamine used to validate specificity .
Q. What is the significance of this compound in the purification of calcium-binding proteins like C-reactive protein (CRP)?
Immobilized this compound on agarose resin binds CRP via calcium-dependent interactions. Affinity chromatography protocols use 20 mM Tris-HCl (pH 7.4) with 2 mM CaCl2 for binding and EDTA-containing buffers for elution. This method achieves >90% purity and avoids non-specific binding observed with phosphorylcholine matrices .
Q. How does this compound functionalization enhance the biocompatibility of nanomaterials?
Surface functionalization of detonation nanodiamonds (DNDs) involves covalent coupling via carbodiimide chemistry, confirmed by FTIR spectroscopy. This modification improves colloidal stability in physiological buffers and enhances binding to titanium alloy surfaces for biomedical implants. Biocompatibility is assessed via cytotoxicity assays and protein adsorption studies .
Q. Methodological Notes
- Data Contradictions : While some studies report elevated this compound under stress , others note depletion in neurodegenerative disease models . These discrepancies may arise from tissue-specific metabolic fluxes or methodological variability in sample preparation.
- Unresolved Questions : The mechanistic link between this compound depletion and neuronal death in Alzheimer’s disease remains unclear. Proposed hypotheses include disrupted PE synthesis or impaired autophagy, requiring knock-in/knockout models for validation .
Eigenschaften
IUPAC Name |
2-aminoethyl dihydrogen phosphate | |
---|---|---|
Source | PubChem | |
URL | https://pubchem.ncbi.nlm.nih.gov | |
Description | Data deposited in or computed by PubChem | |
InChI |
InChI=1S/C2H8NO4P/c3-1-2-7-8(4,5)6/h1-3H2,(H2,4,5,6) | |
Source | PubChem | |
URL | https://pubchem.ncbi.nlm.nih.gov | |
Description | Data deposited in or computed by PubChem | |
InChI Key |
SUHOOTKUPISOBE-UHFFFAOYSA-N | |
Source | PubChem | |
URL | https://pubchem.ncbi.nlm.nih.gov | |
Description | Data deposited in or computed by PubChem | |
Canonical SMILES |
C(COP(=O)(O)O)N | |
Source | PubChem | |
URL | https://pubchem.ncbi.nlm.nih.gov | |
Description | Data deposited in or computed by PubChem | |
Molecular Formula |
C2H8NO4P | |
Source | PubChem | |
URL | https://pubchem.ncbi.nlm.nih.gov | |
Description | Data deposited in or computed by PubChem | |
DSSTOX Substance ID |
DTXSID5061453 | |
Record name | 2-Aminoethyl dihydrogen phosphate | |
Source | EPA DSSTox | |
URL | https://comptox.epa.gov/dashboard/DTXSID5061453 | |
Description | DSSTox provides a high quality public chemistry resource for supporting improved predictive toxicology. | |
Molecular Weight |
141.06 g/mol | |
Source | PubChem | |
URL | https://pubchem.ncbi.nlm.nih.gov | |
Description | Data deposited in or computed by PubChem | |
Physical Description |
Solid | |
Record name | O-Phosphoethanolamine | |
Source | Human Metabolome Database (HMDB) | |
URL | http://www.hmdb.ca/metabolites/HMDB0000224 | |
Description | The Human Metabolome Database (HMDB) is a freely available electronic database containing detailed information about small molecule metabolites found in the human body. | |
Explanation | HMDB is offered to the public as a freely available resource. Use and re-distribution of the data, in whole or in part, for commercial purposes requires explicit permission of the authors and explicit acknowledgment of the source material (HMDB) and the original publication (see the HMDB citing page). We ask that users who download significant portions of the database cite the HMDB paper in any resulting publications. | |
Solubility |
72 mg/mL | |
Record name | O-Phosphoethanolamine | |
Source | Human Metabolome Database (HMDB) | |
URL | http://www.hmdb.ca/metabolites/HMDB0000224 | |
Description | The Human Metabolome Database (HMDB) is a freely available electronic database containing detailed information about small molecule metabolites found in the human body. | |
Explanation | HMDB is offered to the public as a freely available resource. Use and re-distribution of the data, in whole or in part, for commercial purposes requires explicit permission of the authors and explicit acknowledgment of the source material (HMDB) and the original publication (see the HMDB citing page). We ask that users who download significant portions of the database cite the HMDB paper in any resulting publications. | |
CAS No. |
1071-23-4 | |
Record name | Phosphoethanolamine | |
Source | CAS Common Chemistry | |
URL | https://commonchemistry.cas.org/detail?cas_rn=1071-23-4 | |
Description | CAS Common Chemistry is an open community resource for accessing chemical information. Nearly 500,000 chemical substances from CAS REGISTRY cover areas of community interest, including common and frequently regulated chemicals, and those relevant to high school and undergraduate chemistry classes. This chemical information, curated by our expert scientists, is provided in alignment with our mission as a division of the American Chemical Society. | |
Explanation | The data from CAS Common Chemistry is provided under a CC-BY-NC 4.0 license, unless otherwise stated. | |
Record name | Phosphorylethanolamine | |
Source | ChemIDplus | |
URL | https://pubchem.ncbi.nlm.nih.gov/substance/?source=chemidplus&sourceid=0001071234 | |
Description | ChemIDplus is a free, web search system that provides access to the structure and nomenclature authority files used for the identification of chemical substances cited in National Library of Medicine (NLM) databases, including the TOXNET system. | |
Record name | Phosphorylcolamine | |
Source | DrugBank | |
URL | https://www.drugbank.ca/drugs/DB01738 | |
Description | The DrugBank database is a unique bioinformatics and cheminformatics resource that combines detailed drug (i.e. chemical, pharmacological and pharmaceutical) data with comprehensive drug target (i.e. sequence, structure, and pathway) information. | |
Explanation | Creative Common's Attribution-NonCommercial 4.0 International License (http://creativecommons.org/licenses/by-nc/4.0/legalcode) | |
Record name | O-Phosphoethanolamine | |
Source | DTP/NCI | |
URL | https://dtp.cancer.gov/dtpstandard/servlet/dwindex?searchtype=NSC&outputformat=html&searchlist=254167 | |
Description | The NCI Development Therapeutics Program (DTP) provides services and resources to the academic and private-sector research communities worldwide to facilitate the discovery and development of new cancer therapeutic agents. | |
Explanation | Unless otherwise indicated, all text within NCI products is free of copyright and may be reused without our permission. Credit the National Cancer Institute as the source. | |
Record name | Ethanol, 2-amino-, 1-(dihydrogen phosphate) | |
Source | EPA Chemicals under the TSCA | |
URL | https://www.epa.gov/chemicals-under-tsca | |
Description | EPA Chemicals under the Toxic Substances Control Act (TSCA) collection contains information on chemicals and their regulations under TSCA, including non-confidential content from the TSCA Chemical Substance Inventory and Chemical Data Reporting. | |
Record name | 2-Aminoethyl dihydrogen phosphate | |
Source | EPA DSSTox | |
URL | https://comptox.epa.gov/dashboard/DTXSID5061453 | |
Description | DSSTox provides a high quality public chemistry resource for supporting improved predictive toxicology. | |
Record name | 2-aminoethyl dihydrogen phosphate | |
Source | European Chemicals Agency (ECHA) | |
URL | https://echa.europa.eu/substance-information/-/substanceinfo/100.012.717 | |
Description | The European Chemicals Agency (ECHA) is an agency of the European Union which is the driving force among regulatory authorities in implementing the EU's groundbreaking chemicals legislation for the benefit of human health and the environment as well as for innovation and competitiveness. | |
Explanation | Use of the information, documents and data from the ECHA website is subject to the terms and conditions of this Legal Notice, and subject to other binding limitations provided for under applicable law, the information, documents and data made available on the ECHA website may be reproduced, distributed and/or used, totally or in part, for non-commercial purposes provided that ECHA is acknowledged as the source: "Source: European Chemicals Agency, http://echa.europa.eu/". Such acknowledgement must be included in each copy of the material. ECHA permits and encourages organisations and individuals to create links to the ECHA website under the following cumulative conditions: Links can only be made to webpages that provide a link to the Legal Notice page. | |
Record name | PHOSPHORYLCOLAMINE | |
Source | FDA Global Substance Registration System (GSRS) | |
URL | https://gsrs.ncats.nih.gov/ginas/app/beta/substances/78A2BX7AEU | |
Description | The FDA Global Substance Registration System (GSRS) enables the efficient and accurate exchange of information on what substances are in regulated products. Instead of relying on names, which vary across regulatory domains, countries, and regions, the GSRS knowledge base makes it possible for substances to be defined by standardized, scientific descriptions. | |
Explanation | Unless otherwise noted, the contents of the FDA website (www.fda.gov), both text and graphics, are not copyrighted. They are in the public domain and may be republished, reprinted and otherwise used freely by anyone without the need to obtain permission from FDA. Credit to the U.S. Food and Drug Administration as the source is appreciated but not required. | |
Record name | O-Phosphoethanolamine | |
Source | Human Metabolome Database (HMDB) | |
URL | http://www.hmdb.ca/metabolites/HMDB0000224 | |
Description | The Human Metabolome Database (HMDB) is a freely available electronic database containing detailed information about small molecule metabolites found in the human body. | |
Explanation | HMDB is offered to the public as a freely available resource. Use and re-distribution of the data, in whole or in part, for commercial purposes requires explicit permission of the authors and explicit acknowledgment of the source material (HMDB) and the original publication (see the HMDB citing page). We ask that users who download significant portions of the database cite the HMDB paper in any resulting publications. | |
Melting Point |
241-243 °C, 241 - 243 °C | |
Record name | Phosphorylcolamine | |
Source | DrugBank | |
URL | https://www.drugbank.ca/drugs/DB01738 | |
Description | The DrugBank database is a unique bioinformatics and cheminformatics resource that combines detailed drug (i.e. chemical, pharmacological and pharmaceutical) data with comprehensive drug target (i.e. sequence, structure, and pathway) information. | |
Explanation | Creative Common's Attribution-NonCommercial 4.0 International License (http://creativecommons.org/licenses/by-nc/4.0/legalcode) | |
Record name | O-Phosphoethanolamine | |
Source | Human Metabolome Database (HMDB) | |
URL | http://www.hmdb.ca/metabolites/HMDB0000224 | |
Description | The Human Metabolome Database (HMDB) is a freely available electronic database containing detailed information about small molecule metabolites found in the human body. | |
Explanation | HMDB is offered to the public as a freely available resource. Use and re-distribution of the data, in whole or in part, for commercial purposes requires explicit permission of the authors and explicit acknowledgment of the source material (HMDB) and the original publication (see the HMDB citing page). We ask that users who download significant portions of the database cite the HMDB paper in any resulting publications. | |
Retrosynthesis Analysis
AI-Powered Synthesis Planning: Our tool employs the Template_relevance Pistachio, Template_relevance Bkms_metabolic, Template_relevance Pistachio_ringbreaker, Template_relevance Reaxys, Template_relevance Reaxys_biocatalysis model, leveraging a vast database of chemical reactions to predict feasible synthetic routes.
One-Step Synthesis Focus: Specifically designed for one-step synthesis, it provides concise and direct routes for your target compounds, streamlining the synthesis process.
Accurate Predictions: Utilizing the extensive PISTACHIO, BKMS_METABOLIC, PISTACHIO_RINGBREAKER, REAXYS, REAXYS_BIOCATALYSIS database, our tool offers high-accuracy predictions, reflecting the latest in chemical research and data.
Strategy Settings
Precursor scoring | Relevance Heuristic |
---|---|
Min. plausibility | 0.01 |
Model | Template_relevance |
Template Set | Pistachio/Bkms_metabolic/Pistachio_ringbreaker/Reaxys/Reaxys_biocatalysis |
Top-N result to add to graph | 6 |
Feasible Synthetic Routes
Haftungsausschluss und Informationen zu In-Vitro-Forschungsprodukten
Bitte beachten Sie, dass alle Artikel und Produktinformationen, die auf BenchChem präsentiert werden, ausschließlich zu Informationszwecken bestimmt sind. Die auf BenchChem zum Kauf angebotenen Produkte sind speziell für In-vitro-Studien konzipiert, die außerhalb lebender Organismen durchgeführt werden. In-vitro-Studien, abgeleitet von dem lateinischen Begriff "in Glas", beinhalten Experimente, die in kontrollierten Laborumgebungen unter Verwendung von Zellen oder Geweben durchgeführt werden. Es ist wichtig zu beachten, dass diese Produkte nicht als Arzneimittel oder Medikamente eingestuft sind und keine Zulassung der FDA für die Vorbeugung, Behandlung oder Heilung von medizinischen Zuständen, Beschwerden oder Krankheiten erhalten haben. Wir müssen betonen, dass jede Form der körperlichen Einführung dieser Produkte in Menschen oder Tiere gesetzlich strikt untersagt ist. Es ist unerlässlich, sich an diese Richtlinien zu halten, um die Einhaltung rechtlicher und ethischer Standards in Forschung und Experiment zu gewährleisten.