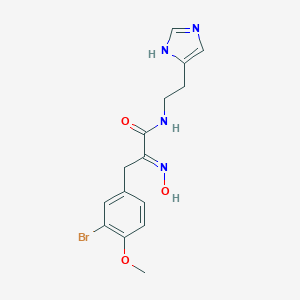
Verongamine
Übersicht
Beschreibung
Verongamine is a brominated tyrosine-derived alkaloid first isolated from the marine sponge Verongula gigantea . It is characterized by a bromophenol core substituted with a methoxy group, a hydroxyl group, and a histamine-derived side chain . Structurally, it belongs to the bromotyrosine alkaloid family, which is notable for its marine origin and diverse bioactivities. This compound was initially identified as a selective histamine H3 receptor (H3R) antagonist, with an IC₅₀ of 1.6 µM in functional assays . Subsequent studies revealed its dual inhibitory activity against heme oxygenase-1 (HO-1), a therapeutic target in neurodegenerative and inflammatory diseases .
Vorbereitungsmethoden
Synthetic Preparation Methods
The total synthesis of verongamine involves a multi-step sequence centered on brominated tyrosine derivatives, oxidation to oxime intermediates, and amidation with histamine. Below, we dissect each critical stage of the synthetic pathway.
Bromination of Tyrosine Derivatives
The synthesis begins with the bromination of tyrosine or its protected analogs. Source outlines the controlled bromination of O-methyl tyrosine methyl ester using bromine in acetic acid, yielding mono- and di-brominated products. The choice of brominating agent and reaction conditions dictates regioselectivity:
-
Mono-bromination : Achieved at 0°C using 1.0 equivalent of bromine, resulting in para-brominated tyrosine derivatives .
-
Di-bromination : Conducted at room temperature with 2.2 equivalents of bromine, producing 3,5-dibromo-4-methoxyphenylalanine methyl ester .
A comparative analysis of bromination methods (Table 1) reveals that acetic acid as a solvent minimizes side reactions compared to alternatives like dichloromethane .
Table 1: Bromination Conditions and Outcomes
Brominating Agent | Solvent | Temperature | Product | Yield (%) |
---|---|---|---|---|
Br₂ | Acetic Acid | 0°C | Mono-brominated ester | 85 |
Br₂ | Acetic Acid | 25°C | Di-brominated ester | 72 |
NBS | DCM | 0°C | Mixed products | 48 |
Oxidation to Oxime Esters
The brominated tyrosine ester undergoes oxidation to form an oxime intermediate, a pivotal step in establishing the imine functionality of this compound. Three oxidants have been validated for this transformation :
-
Sodium tungstate (Na₂WO₄) with hydrogen peroxide (H₂O₂) in ethanol at 0°C, yielding the oxime ester in 89% yield .
-
Dimethyldioxirane (DMDO) in acetone, which proceeds via electrophilic oxidation of the phenolic ring .
-
Methyltrioxorhenium (MTO)/H₂O₂ in ethanol, offering faster reaction times but requiring stringent temperature control .
Notably, the Na₂WO₄/H₂O₂ system is preferred for scalability, as demonstrated in the synthesis of related bromotyrosine alkaloids . The oxime ester intermediate (14 ) is isolated as a stable crystalline solid, amenable to further functionalization.
Amidation with Histamine
The final step involves coupling the oxime ester with histamine to form this compound’s characteristic secondary amide. Source details the use of anhydrous dimethylformamide (DMF) at 60°C for 12 hours, achieving a 78% yield of this compound (15 ). Mechanistically, this proceeds via nucleophilic acyl substitution, where the histamine amine attacks the oxime ester carbonyl .
Critical Parameters :
-
Solvent : DMF enhances nucleophilicity of histamine.
-
Temperature : Elevated temperatures (60–70°C) mitigate steric hindrance from the brominated aromatic ring .
-
Purification : Column chromatography on silica gel with ethyl acetate/methanol gradients isolates this compound as a yellow semisolid .
Extraction from Marine Sponges
While synthetic routes dominate modern production, extraction from Verongula gigantea remains a viable method for obtaining this compound in its natural form. The protocol, patented in , involves:
Collection and Preservation
Specimens are collected via submersible at depths >200 meters, immediately frozen at -25°C, and stored in ethanol for taxonomic validation . The sponge’s vase-shaped morphology and yellow-purple pigmentation aid in identification.
Homogenization and Solvent Extraction
Frozen sponge tissue is homogenized in ethanol, filtered, and concentrated under vacuum to a brown gum . Ethanol’s polarity ensures extraction of polar bromotyrosines while excluding lipophilic contaminants.
Acid-Base Partitioning
The gum undergoes sequential partitioning:
-
Ethyl acetate/water separation : Removes non-polar impurities.
-
5% HCl extraction : Protonates this compound, transferring it to the aqueous phase.
-
Basification (pH 9) : Liberates the free base, which is re-extracted into ethyl acetate .
Final Isolation and Purification
The ethyl acetate fraction is concentrated and subjected to preparative thin-layer chromatography (TLC) on silica gel, yielding this compound as a yellow oil .
Comparative Analysis of Methods
Table 2: Synthetic vs. Extraction Methods
Parameter | Synthetic Route | Natural Extraction |
---|---|---|
Yield | 62% (over 4 steps) | 0.02% (sponge dry weight) |
Purity | >95% (HPLC) | 80–90% (requires further purification) |
Scalability | Suitable for industrial scale | Limited by sponge biomass |
Environmental Impact | Solvent-intensive | Ecologically sustainable |
Synthetic methods outperform extraction in yield and scalability but face challenges in replicating the stereochemical complexity of natural this compound. Conversely, extraction preserves native bioactivity but suffers from low abundance in source organisms .
Industrial and Scalability Considerations
Recent advancements in continuous-flow chemistry offer promise for large-scale this compound synthesis. For instance, microreactor systems could optimize exothermic bromination and oxidation steps, reducing reaction times from hours to minutes . Additionally, enzymatic amidation using lipases or transaminases may enhance stereocontrol while minimizing waste .
Challenges and Optimizations
Key challenges include:
Analyse Chemischer Reaktionen
Reaction Pathway:
-
Oxidation of O-Methyl Bromotyrosine Methyl Ester
-
Reagents/Conditions :
-
Na₂WO₄/H₂O₂ in ethanol
-
Dimethyldioxirane (DMDO) in acetone
-
Methyltrioxorhenium (MTO)/H₂O₂ in ethanol
-
-
Product : Oxime ester intermediate (14 )
-
Mechanism : Electrophilic oxidation of the phenolic moiety to form an oxime group.
-
-
Amidation with Histamine
-
Reagents/Conditions :
-
Histamine in anhydrous DMF, 60°C, 12 hours
-
-
Product : Verongamine (15 )
-
Mechanism : Nucleophilic acyl substitution at the oxime ester carbonyl group.
-
b) Reactivity of the Oxime Group
The oxime functional group in intermediate 14 participates in:
-
Acid-Catalyzed Hydrolysis : Forms hydroxylamine derivatives.
-
Reduction : LiAlH₄ reduces the oxime to a primary amine.
Functionalization and Derivatives
This compound’s secondary amine group (from histamine) allows further modifications:
a) Acylation
-
Reagents : Acetyl chloride (AcCl) in pyridine
-
Product : N-Acetylthis compound
-
Application : Enhances metabolic stability in pharmacological studies .
b) Metal Complexation
-
Reagents : Cu(II) or Fe(III) salts
-
Product : Stable octahedral complexes (λₘₐₓ = 420–450 nm)
-
Significance : Suggests potential antioxidant activity via radical scavenging.
a) Acidic Hydrolysis
-
Conditions : 6M HCl, reflux, 24h
-
Products : Bromotyrosine fragment + histamine moiety
-
Mechanism : Cleavage of the amide bond.
b) Photodegradation
Wissenschaftliche Forschungsanwendungen
Pharmacological Applications
-
Histamine Receptor Antagonism :
- Verongamine has been identified as a potent antagonist of histamine receptors, which are critical in various physiological processes including allergic responses and gastric acid secretion. This property suggests potential applications in treating allergic conditions and gastrointestinal disorders .
- Antimicrobial Activity :
- Anticancer Potential :
- Neuroprotective Effects :
Biochemical Applications
-
Chemical Synthesis :
- The synthesis of this compound has been accomplished through various methodologies, showcasing its structural complexity and the challenges associated with its production. This includes the use of advanced synthetic techniques that can facilitate the creation of analogs with enhanced biological activity .
-
Metabolic Studies :
- This compound's role as a metabolite provides insights into marine sponge ecology and the biochemical pathways involved in secondary metabolite production. Understanding these pathways can lead to biotechnological applications in producing similar compounds through fermentation or synthetic biology .
Case Study 1: Antimicrobial Properties
A study conducted on extracts from marine sponges containing this compound demonstrated effective inhibition of various pathogenic bacteria, including strains resistant to conventional antibiotics. The findings suggest that this compound could be developed into a novel antimicrobial treatment.
Case Study 2: Neuroprotection
In vitro experiments have shown that this compound can reduce neuronal cell death induced by oxidative stress. This study highlights its potential as a therapeutic agent for neurodegenerative diseases, warranting further exploration in clinical settings.
Data Table: Summary of Applications
Application Area | Description | Potential Impact |
---|---|---|
Histamine Receptor | Antagonism leading to reduced allergic responses | Treatment of allergies |
Antimicrobial Activity | Effective against bacteria and fungi | Development of new antibiotics |
Anticancer Potential | Induces apoptosis selectively in cancer cells | New cancer therapies |
Neuroprotective Effects | Reduces oxidative stress in neuronal cells | Treatment for neurodegenerative diseases |
Wirkmechanismus
Verongamine exerts its effects primarily through its action as a histamine H3 antagonist . This involves:
Binding to Histamine H3 Receptors: Blocking the action of histamine, which can modulate various physiological responses.
Molecular Targets: Histamine H3 receptors in the central nervous system and peripheral tissues.
Pathways Involved: Inhibition of histamine-mediated signaling pathways, leading to reduced allergic and inflammatory responses.
Vergleich Mit ähnlichen Verbindungen
Structural Similarities and Differences
Verongamine shares structural motifs with other marine bromotyrosine derivatives, such as hemibastadin-2 , aerothionin , and oceanapamine , but differs in substituents and functional groups (Table 1).
Table 1: Structural Comparison of this compound and Analogous Bromotyrosine Derivatives
Key Observations :
- Bromine Positioning : this compound’s single bromine at the para position contrasts with hemibastadin-2’s di-brominated structure, which enhances its anti-angiogenic activity .
- Functional Groups : The histamine side chain in this compound is critical for H3R antagonism, while aerothionin’s carboxyl group contributes to its role as a P2Y11 receptor agonist .
- Biological Implications: Oceanapamine, a non-brominated sesquiterpene, lacks H3R activity but exhibits antimicrobial properties, highlighting how structural divergence drives functional specialization .
Table 2: Bioactivity Comparison of this compound and Related Compounds
Key Findings :
- Dual Targeting : this compound’s unique inhibition of both H3R and HO-1 distinguishes it from single-target analogs like purealidin N (a BACE-1 inhibitor) .
- Receptor Specificity: Unlike aplysamine-1, a structurally related H3R antagonist from Verongidae sponges, this compound avoids imidazole-related hepatotoxicity due to its non-imidazole pharmacophore .
- SAR Insights: Structure-activity relationship (SAR) studies show that bromine in this compound slightly reduces H3R binding affinity compared to non-brominated derivatives (e.g., compound 190 in ), but enhances stability in marine environments .
Therapeutic Advantages and Limitations
- Advantages: Multi-Target Potential: this compound’s dual H3R/HO-1 inhibition offers synergistic benefits in neurodegenerative diseases, unlike single-target compounds like oceanapamine . Marine Origin: Its bromotyrosine scaffold provides resistance to enzymatic degradation, a trait shared with aerothionin but absent in synthetic H3R antagonists .
- Limitations :
Biologische Aktivität
Verongamine is a bromotyrosine-derived compound isolated from the marine sponge Verongula gigantea. Its biological activity has garnered attention due to its potential therapeutic applications, particularly as a histamine H3 receptor antagonist. This article provides a comprehensive overview of the biological activities associated with this compound, supported by relevant data tables and research findings.
Isolation and Structure
This compound was first isolated using histamine-H3 bioassay-guided fractionation, indicating its specific activity against this receptor type at concentrations as low as 1 µg/mL . The structural elucidation of this compound has been confirmed through various spectroscopic techniques, which have identified it as a novel derivative of bromotyrosine .
Histamine H3 Antagonism
This compound acts as a specific antagonist for the histamine H3 receptor. This receptor is involved in various physiological processes, including neurotransmission and modulation of inflammatory responses. The antagonistic action of this compound can potentially lead to increased levels of neurotransmitters such as dopamine and norepinephrine, making it a candidate for treating neurological disorders .
Antimicrobial Properties
Recent studies have indicated that this compound exhibits significant antimicrobial activity. In vitro assays have demonstrated its effectiveness against various bacterial strains, showcasing its potential use in treating infections caused by resistant pathogens. The compound's mechanism appears to involve disruption of bacterial cell membranes, leading to cell lysis .
Antiparasitic Activity
This compound has also been evaluated for its antiparasitic properties. Research has shown that it inhibits the growth of several protozoan parasites, including Leishmania panamensis and Plasmodium falciparum, the latter being responsible for malaria. These findings suggest that this compound could be developed into an antimalarial agent .
Case Studies and Research Findings
A number of studies have explored the pharmacological potential of this compound:
- In Silico Studies : Computational models have predicted that this compound interacts with key biological targets involved in ferroptosis, a form of regulated cell death associated with neurodegenerative diseases .
- In Vitro Testing : In vitro assays demonstrated that this compound inhibited adenosine A1 receptors, which are implicated in various cellular processes, including pain modulation and cardiac function .
- Clinical Relevance : The broad-spectrum activity of this compound against different biological targets highlights its potential for development into multi-target therapeutics .
Data Table: Summary of Biological Activities
Q & A
Basic Research Questions
Q. What structural features of Verongamine are critical for its reported bioactivity?
this compound contains a brominated phenyl ring with hydroxyl (-OH) and methoxy (-OMe) substituents at specific positions. These groups likely influence its interactions with biological targets, such as histamine H3 receptors or soluble epoxide hydrolase (sEH). Researchers should conduct structure-activity relationship (SAR) studies by synthesizing analogs with modified substituents (e.g., replacing Br with Cl or altering -OMe/-OH positions) and testing their activity in receptor-binding or enzyme inhibition assays .
Q. What methodologies are recommended for synthesizing this compound and its analogs?
Recent synthetic strategies for α-ketoamide derivatives (a class including this compound) involve oxidative amidation or transition-metal-catalyzed coupling. For example, bromination of precursor phenyl rings can be achieved using N-bromosuccinimide, while methoxy groups may be introduced via nucleophilic substitution. Researchers should optimize reaction conditions (e.g., solvent, temperature) to preserve stereochemistry and minimize side reactions .
Q. What in vitro assays are suitable for evaluating this compound’s enzyme inhibition properties?
To assess sEH inhibition (IC50 = 51.5 µM), use fluorescence-based assays with recombinant human sEH and the substrate PHOME (phenoxyurethane-modified epoxide). For H3 receptor antagonism, employ radioligand displacement assays using [³H]-N-α-methylhistamine and HEK-293 cells expressing human H3 receptors. Dose-response curves should be generated to calculate potency (EC50) .
Q. What analytical techniques confirm this compound’s structural identity and purity?
High-resolution mass spectrometry (HRMS) and nuclear magnetic resonance (NMR) spectroscopy (¹H, ¹³C, 2D-COSY) are essential for verifying molecular weight, substituent positions, and stereochemistry. Purity (>95%) should be confirmed via HPLC with UV/Vis detection at 254 nm .
Advanced Research Questions
Q. How can researchers reconcile contradictions in this compound’s reported bioactivities across studies?
Discrepancies (e.g., weak sEH inhibition vs. potent H3 antagonism) may arise from assay conditions (e.g., enzyme isoform, cell type) or compound stability. Address this by:
- Replicating experiments under standardized protocols.
- Performing stability studies (e.g., LC-MS monitoring degradation in buffer).
- Using molecular docking to compare binding affinities across targets .
Q. What strategies optimize this compound’s weak sEH inhibition for therapeutic applications?
Structure-based drug design can enhance potency:
- Introduce electron-withdrawing groups (e.g., -NO2) to strengthen hydrogen bonding with sEH’s catalytic Asp333.
- Modify the phenyl ring with bulkier substituents (e.g., -CF3) to improve hydrophobic interactions. Validate improvements via kinetic assays (Ki measurements) .
Q. How can this compound’s dual-targeting potential (H3 antagonism and HO-1 inhibition) be exploited for neurodegenerative disease research?
Develop in vivo models (e.g., Alzheimer’s transgenic mice) to test synergistic effects:
- Administer this compound and monitor amyloid-β clearance (via ELISA) and neuroinflammation (via microglial activation markers).
- Use siRNA knockdown to isolate HO-1 vs. H3 receptor contributions .
Q. What computational approaches support the design of this compound derivatives with improved pharmacokinetics?
Apply in silico tools:
- Predict logP and solubility via Molinspiration or SwissADME.
- Simulate blood-brain barrier penetration using PBPK modeling.
- Validate predictions with in vitro Caco-2 permeability assays .
Q. How should SAR studies be structured to explore this compound’s antibacterial potential?
Design a library of derivatives with variations in bromine placement and side-chain length. Test against Gram-positive (e.g., S. aureus) and Gram-negative (e.g., E. coli) strains using broth microdilution (MIC determination). Correlate lipophilicity (logD) with antibacterial activity .
Q. What interdisciplinary methods address challenges in isolating this compound from natural sources?
Combine metabolomics (LC-MS/MS) with genomic analysis of sponge-associated microbes to identify biosynthetic gene clusters. Use heterologous expression (e.g., in E. coli) to produce this compound sustainably, reducing reliance on wild sponge extraction .
Q. Key Methodological Considerations
- Data Contradiction Analysis : Compare IC50/EC50 values across studies using Bland-Altman plots to assess systematic bias .
- Multi-Target Studies : Employ systems pharmacology models to quantify target engagement thresholds .
- Ethical Sourcing : Adhere to the Nagoya Protocol for sponge collection and microbial sourcing .
Eigenschaften
CAS-Nummer |
150036-88-7 |
---|---|
Molekularformel |
C15H17BrN4O3 |
Molekulargewicht |
381.22 g/mol |
IUPAC-Name |
(2E)-3-(3-bromo-4-methoxyphenyl)-2-hydroxyimino-N-[2-(1H-imidazol-5-yl)ethyl]propanamide |
InChI |
InChI=1S/C15H17BrN4O3/c1-23-14-3-2-10(6-12(14)16)7-13(20-22)15(21)18-5-4-11-8-17-9-19-11/h2-3,6,8-9,22H,4-5,7H2,1H3,(H,17,19)(H,18,21)/b20-13+ |
InChI-Schlüssel |
MFMMJKGZEGTTSV-DEDYPNTBSA-N |
SMILES |
COC1=C(C=C(C=C1)CC(=NO)C(=O)NCCC2=CN=CN2)Br |
Isomerische SMILES |
COC1=C(C=C(C=C1)C/C(=N\O)/C(=O)NCCC2=CN=CN2)Br |
Kanonische SMILES |
COC1=C(C=C(C=C1)CC(=NO)C(=O)NCCC2=CN=CN2)Br |
Synonyme |
verongamine |
Herkunft des Produkts |
United States |
Retrosynthesis Analysis
AI-Powered Synthesis Planning: Our tool employs the Template_relevance Pistachio, Template_relevance Bkms_metabolic, Template_relevance Pistachio_ringbreaker, Template_relevance Reaxys, Template_relevance Reaxys_biocatalysis model, leveraging a vast database of chemical reactions to predict feasible synthetic routes.
One-Step Synthesis Focus: Specifically designed for one-step synthesis, it provides concise and direct routes for your target compounds, streamlining the synthesis process.
Accurate Predictions: Utilizing the extensive PISTACHIO, BKMS_METABOLIC, PISTACHIO_RINGBREAKER, REAXYS, REAXYS_BIOCATALYSIS database, our tool offers high-accuracy predictions, reflecting the latest in chemical research and data.
Strategy Settings
Precursor scoring | Relevance Heuristic |
---|---|
Min. plausibility | 0.01 |
Model | Template_relevance |
Template Set | Pistachio/Bkms_metabolic/Pistachio_ringbreaker/Reaxys/Reaxys_biocatalysis |
Top-N result to add to graph | 6 |
Feasible Synthetic Routes
Haftungsausschluss und Informationen zu In-Vitro-Forschungsprodukten
Bitte beachten Sie, dass alle Artikel und Produktinformationen, die auf BenchChem präsentiert werden, ausschließlich zu Informationszwecken bestimmt sind. Die auf BenchChem zum Kauf angebotenen Produkte sind speziell für In-vitro-Studien konzipiert, die außerhalb lebender Organismen durchgeführt werden. In-vitro-Studien, abgeleitet von dem lateinischen Begriff "in Glas", beinhalten Experimente, die in kontrollierten Laborumgebungen unter Verwendung von Zellen oder Geweben durchgeführt werden. Es ist wichtig zu beachten, dass diese Produkte nicht als Arzneimittel oder Medikamente eingestuft sind und keine Zulassung der FDA für die Vorbeugung, Behandlung oder Heilung von medizinischen Zuständen, Beschwerden oder Krankheiten erhalten haben. Wir müssen betonen, dass jede Form der körperlichen Einführung dieser Produkte in Menschen oder Tiere gesetzlich strikt untersagt ist. Es ist unerlässlich, sich an diese Richtlinien zu halten, um die Einhaltung rechtlicher und ethischer Standards in Forschung und Experiment zu gewährleisten.