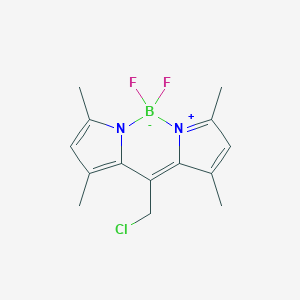
Bodipy 8-Chloromethan
Übersicht
Beschreibung
Bodipy 8-Chloromethane is an organic compound with the chemical formula C14H16BClF2N2 and a molecular weight of 296.55. It is a yellow to orange crystalline solid that contains carbon, hydrogen, chlorine, boron, fluorine, and nitrogen elements. This compound is widely used in various fields such as chemical sensing, fluorescent dyes, biological markers, and photosensitive materials. It serves as a fluorescent probe and microscopy imaging reagent, enabling real-time monitoring of molecules, cells, and structures within biological systems .
Wissenschaftliche Forschungsanwendungen
Bodipy 8-Chloromethane has a wide range of scientific research applications, including:
Biochemische Analyse
Biochemical Properties
Bodipy 8-Chloromethane has been employed in areas like photodynamic therapy . It interacts with various biomolecules, playing a significant role in biochemical reactions
Cellular Effects
Bodipy 8-Chloromethane has been used as a probe in applications like imaging and sensing . It can monitor molecules, cells, and structures within biological systems in real-time
Molecular Mechanism
It is known that BODIPY-derived triplet photosensitizers have mechanisms including the heavy atom effect, exciton coupling, and charge recombination (CR)-induced ISC
Vorbereitungsmethoden
Bodipy 8-Chloromethane is typically synthesized through chemical synthesis methods. The preparation process involves introducing appropriate raw materials and reagents into the reaction system, followed by reaction, purification, and crystallization to obtain the final product . The specific synthetic routes and reaction conditions can vary, but common methods include:
Pd-catalyzed cross-coupling reactions: These reactions involve the coupling of boron dipyrromethene (BODIPY) core with chloromethane under palladium catalysis.
Grignard reactions: This method involves the reaction of a Grignard reagent with a suitable precursor to form the desired compound.
Knoevenagel reaction: This reaction involves the condensation of an aldehyde or ketone with a malonic acid derivative in the presence of a base.
Industrial production methods for Bodipy 8-Chloromethane are similar to laboratory synthesis but are scaled up to meet commercial demands. These methods ensure high purity and yield of the compound.
Analyse Chemischer Reaktionen
Bodipy 8-Chloromethane undergoes various chemical reactions, including:
Common reagents and conditions used in these reactions include strong oxidizing agents, reducing agents, and catalysts like palladium or platinum. The major products formed from these reactions depend on the specific reaction conditions and reagents used.
Wirkmechanismus
The mechanism by which Bodipy 8-Chloromethane exerts its effects involves its photophysical properties. When exposed to light, the compound absorbs photons and transitions to an excited state. This excited state can then transfer energy to molecular oxygen, generating reactive oxygen species such as singlet oxygen. These reactive species can oxidize biomolecules, leading to cell death in photodynamic therapy applications . The molecular targets and pathways involved include cellular membranes, proteins, and nucleic acids.
Vergleich Mit ähnlichen Verbindungen
Bodipy 8-Chloromethane is unique compared to other similar compounds due to its specific photophysical properties, such as strong fluorescence, high photostability, and ease of functionalization. Similar compounds include:
Bodipy-Fluorene-Fullerene Conjugates: These compounds exhibit high molar extinction coefficients and good singlet oxygen quantum yields, making them effective photosensitizers.
Bodipy-Fluorene-Bodipy Conjugates: These compounds are also used as photosensitizers but have different photophysical properties compared to Bodipy 8-Chloromethane.
Biologische Aktivität
Bodipy (Boron-dipyrromethene) derivatives, including 8-chloromethane, have gained significant attention in biological applications due to their unique photophysical properties. This article explores the biological activity of Bodipy 8-chloromethane, focusing on its synthesis, photophysical properties, and applications in biomedical research.
Bodipy 8-chloromethane is synthesized through a series of chlorination reactions on the BODIPY core. The chlorination typically occurs at the 8-position, followed by regioselective functionalization at other positions. The synthesis involves:
- Reagents : Trichloroisocyanuric acid (TCCA) is commonly used for chlorination.
- Conditions : The reactions are often carried out in acetic acid under reflux conditions to enhance yield and selectivity.
The resulting compound exhibits distinct spectral properties that are essential for its biological applications. For instance, the absorption and emission wavelengths can be tuned by modifying substituents on the BODIPY core, making it suitable for various imaging techniques .
Photophysical Properties
Bodipy 8-chloromethane displays remarkable photophysical characteristics:
- Absorption Maximum : Typically around 700 nm.
- Emission Maximum : Approximately 738 nm.
- Quantum Yield : High fluorescence quantum yield, which is advantageous for imaging applications.
These properties enable Bodipy derivatives to be employed as fluorescent probes in biological systems, allowing for real-time imaging of cellular processes .
Fluorescent Probes
Bodipy 8-chloromethane has been utilized as a fluorescent probe in various biological studies:
- Cell Imaging : Due to its high quantum yield and stability, it is effective for imaging live cells. Studies have demonstrated its ability to stain specific cellular components, aiding in the visualization of cellular structures and dynamics.
- Detection of Biomolecules : The compound can be functionalized to target specific biomolecules, such as proteins or nucleic acids. For example, Bodipy derivatives have been developed to detect amyloid aggregates associated with neurodegenerative diseases .
Case Studies
- Cancer Detection : Research has shown that Bodipy-based probes can selectively target cancer cells. For instance, a study demonstrated that a Bodipy conjugate could effectively visualize tumor cells in vivo, providing insights into tumor dynamics and treatment responses .
- Oxidative Stress Monitoring : Bodipy derivatives have been designed to detect reactive oxygen species (ROS) in cells. These probes exhibit a significant increase in fluorescence upon reaction with ROS, allowing for the monitoring of oxidative stress levels within biological systems .
Data Table: Summary of Key Properties
Property | Value |
---|---|
Chemical Formula | C14H16BClF2N2 |
Absorption Maximum | ~700 nm |
Emission Maximum | ~738 nm |
Quantum Yield | High |
Solubility | Soluble in organic solvents |
Eigenschaften
IUPAC Name |
8-(chloromethyl)-2,2-difluoro-4,6,10,12-tetramethyl-3-aza-1-azonia-2-boranuidatricyclo[7.3.0.03,7]dodeca-1(12),4,6,8,10-pentaene | |
---|---|---|
Source | PubChem | |
URL | https://pubchem.ncbi.nlm.nih.gov | |
Description | Data deposited in or computed by PubChem | |
InChI |
InChI=1S/C14H16BClF2N2/c1-8-5-10(3)19-13(8)12(7-16)14-9(2)6-11(4)20(14)15(19,17)18/h5-6H,7H2,1-4H3 | |
Source | PubChem | |
URL | https://pubchem.ncbi.nlm.nih.gov | |
Description | Data deposited in or computed by PubChem | |
InChI Key |
PFXASTXHAMZMNO-UHFFFAOYSA-N | |
Source | PubChem | |
URL | https://pubchem.ncbi.nlm.nih.gov | |
Description | Data deposited in or computed by PubChem | |
Canonical SMILES |
[B-]1(N2C(=CC(=C2C(=C3[N+]1=C(C=C3C)C)CCl)C)C)(F)F | |
Source | PubChem | |
URL | https://pubchem.ncbi.nlm.nih.gov | |
Description | Data deposited in or computed by PubChem | |
Molecular Formula |
C14H16BClF2N2 | |
Source | PubChem | |
URL | https://pubchem.ncbi.nlm.nih.gov | |
Description | Data deposited in or computed by PubChem | |
DSSTOX Substance ID |
DTXSID40479458 | |
Record name | AGN-PC-0NI0KZ | |
Source | EPA DSSTox | |
URL | https://comptox.epa.gov/dashboard/DTXSID40479458 | |
Description | DSSTox provides a high quality public chemistry resource for supporting improved predictive toxicology. | |
Molecular Weight |
296.55 g/mol | |
Source | PubChem | |
URL | https://pubchem.ncbi.nlm.nih.gov | |
Description | Data deposited in or computed by PubChem | |
CAS No. |
208462-25-3 | |
Record name | AGN-PC-0NI0KZ | |
Source | EPA DSSTox | |
URL | https://comptox.epa.gov/dashboard/DTXSID40479458 | |
Description | DSSTox provides a high quality public chemistry resource for supporting improved predictive toxicology. | |
Retrosynthesis Analysis
AI-Powered Synthesis Planning: Our tool employs the Template_relevance Pistachio, Template_relevance Bkms_metabolic, Template_relevance Pistachio_ringbreaker, Template_relevance Reaxys, Template_relevance Reaxys_biocatalysis model, leveraging a vast database of chemical reactions to predict feasible synthetic routes.
One-Step Synthesis Focus: Specifically designed for one-step synthesis, it provides concise and direct routes for your target compounds, streamlining the synthesis process.
Accurate Predictions: Utilizing the extensive PISTACHIO, BKMS_METABOLIC, PISTACHIO_RINGBREAKER, REAXYS, REAXYS_BIOCATALYSIS database, our tool offers high-accuracy predictions, reflecting the latest in chemical research and data.
Strategy Settings
Precursor scoring | Relevance Heuristic |
---|---|
Min. plausibility | 0.01 |
Model | Template_relevance |
Template Set | Pistachio/Bkms_metabolic/Pistachio_ringbreaker/Reaxys/Reaxys_biocatalysis |
Top-N result to add to graph | 6 |
Feasible Synthetic Routes
Haftungsausschluss und Informationen zu In-Vitro-Forschungsprodukten
Bitte beachten Sie, dass alle Artikel und Produktinformationen, die auf BenchChem präsentiert werden, ausschließlich zu Informationszwecken bestimmt sind. Die auf BenchChem zum Kauf angebotenen Produkte sind speziell für In-vitro-Studien konzipiert, die außerhalb lebender Organismen durchgeführt werden. In-vitro-Studien, abgeleitet von dem lateinischen Begriff "in Glas", beinhalten Experimente, die in kontrollierten Laborumgebungen unter Verwendung von Zellen oder Geweben durchgeführt werden. Es ist wichtig zu beachten, dass diese Produkte nicht als Arzneimittel oder Medikamente eingestuft sind und keine Zulassung der FDA für die Vorbeugung, Behandlung oder Heilung von medizinischen Zuständen, Beschwerden oder Krankheiten erhalten haben. Wir müssen betonen, dass jede Form der körperlichen Einführung dieser Produkte in Menschen oder Tiere gesetzlich strikt untersagt ist. Es ist unerlässlich, sich an diese Richtlinien zu halten, um die Einhaltung rechtlicher und ethischer Standards in Forschung und Experiment zu gewährleisten.