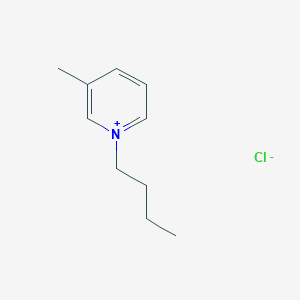
1-Butyl-3-methylpyridiniumchlorid
Übersicht
Beschreibung
1-Butyl-3-methylpyridinium chloride is an ionic liquid composed of a pyridinium cation and a chloride anion. It is known for its unique properties, such as low volatility, high thermal stability, and the ability to dissolve a wide range of organic and inorganic compounds . These characteristics make it a valuable compound in various scientific and industrial applications.
Wissenschaftliche Forschungsanwendungen
1-Butyl-3-methylpyridinium chloride has a wide range of applications in scientific research:
Biology: The compound is used in the extraction and purification of biomolecules, as well as in the stabilization of proteins and enzymes.
Biochemische Analyse
Biochemical Properties
The biochemical properties of 1-Butyl-3-methylpyridinium Chloride are not fully understood yet. It is known that this compound can interact with various biomolecules. For instance, it has been used as a solvent in various chemical reactions due to its ability to dissolve a wide range of organic and inorganic compounds .
Cellular Effects
The cellular effects of 1-Butyl-3-methylpyridinium Chloride are not well-documented. It is known that ionic liquids can have significant effects on cellular processes. For example, some ionic liquids have been found to interact with extracellular polymeric substances (EPSs), leading to changes in the conformational structure of EPSs and potentially affecting microbial cell activity .
Molecular Mechanism
It is known that the properties of ionic liquids, including this compound, depend on the nature and energy of the interaction between the cation and anion .
Temporal Effects in Laboratory Settings
It is known that the properties of ionic liquids can change over time due to factors such as temperature and vibrational and conformational motions .
Metabolic Pathways
It is known that ionic liquids can interact with various enzymes and cofactors .
Transport and Distribution
It is known that ionic liquids can interact with various transporters and binding proteins .
Subcellular Localization
It is known that ionic liquids can interact with various cellular compartments and organelles .
Vorbereitungsmethoden
1-Butyl-3-methylpyridinium chloride can be synthesized through the reaction of pyridine with 1-chlorobutane in the presence of a base . The reaction typically involves mixing pyridine and 1-chlorobutane, followed by the addition of a base to facilitate the reaction. The product is then purified through crystallization. Industrial production methods may involve similar synthetic routes but on a larger scale, with optimizations for yield and purity .
Analyse Chemischer Reaktionen
1-Butyl-3-methylpyridinium chloride undergoes various chemical reactions, including:
Substitution Reactions: It can participate in nucleophilic substitution reactions where the chloride ion is replaced by other nucleophiles.
Oxidation and Reduction: The compound can be involved in redox reactions, although specific conditions and reagents depend on the desired outcome.
Complex Formation: It can form complexes with various metal ions, which can be useful in catalysis and other applications.
Common reagents used in these reactions include strong bases, oxidizing agents, and reducing agents. The major products formed depend on the specific reaction conditions and the reagents used.
Wirkmechanismus
The mechanism of action of 1-Butyl-3-methylpyridinium chloride involves its ability to interact with various molecular targets through ionic interactions and hydrogen bonding. These interactions can stabilize or destabilize certain molecular structures, facilitating chemical reactions or stabilizing biomolecules . The specific pathways involved depend on the application and the molecular targets.
Vergleich Mit ähnlichen Verbindungen
1-Butyl-3-methylpyridinium chloride can be compared with other similar ionic liquids, such as:
1-Butyl-3-methylimidazolium chloride: Similar in structure but with an imidazolium cation instead of a pyridinium cation.
1-Butyl-1-methylpyrrolidinium chloride: Contains a pyrrolidinium cation, offering different chemical reactivity and physical properties.
The uniqueness of 1-Butyl-3-methylpyridinium chloride lies in its specific combination of thermal stability, solubility, and reactivity, making it suitable for a wide range of applications.
Biologische Aktivität
1-Butyl-3-methylpyridinium chloride (BMPC) is a pyridinium-based ionic liquid that has garnered attention for its diverse biological activities and potential applications in various fields, including biochemistry, environmental science, and pharmacology. This article provides a comprehensive overview of the biological activity of BMPC, supported by relevant research findings, case studies, and data tables.
1-Butyl-3-methylpyridinium chloride has the chemical formula CHClN and a molecular weight of approximately 189.7 g/mol. Its structure includes a butyl group and a methyl group attached to a pyridine ring, contributing to its unique properties as an ionic liquid.
1. Antimicrobial Properties
BMPC has demonstrated significant antimicrobial activity against various bacterial strains. A study assessing its efficacy against both Gram-positive and Gram-negative bacteria found that BMPC exhibited a minimum inhibitory concentration (MIC) of 0.5 to 2.0 mg/mL for several strains, including Escherichia coli and Staphylococcus aureus. The mechanism of action appears to involve disruption of bacterial cell membranes, leading to cell lysis.
2. Cytotoxicity Studies
Research into the cytotoxic effects of BMPC on mammalian cells revealed that it has dose-dependent cytotoxicity. In vitro assays using human liver (HepG2) and breast cancer (MCF-7) cell lines showed IC values ranging from 50 to 100 µM, indicating moderate toxicity at higher concentrations. The cytotoxic effects were attributed to oxidative stress and apoptosis induction.
3. Ecotoxicological Impact
The ecotoxicological effects of BMPC have been evaluated in aquatic ecosystems. A life cycle assessment study indicated that while BMPC is less toxic than some imidazolium-based ionic liquids, it still poses risks to aquatic organisms at elevated concentrations. The study highlighted the need for careful management of ionic liquid disposal to mitigate environmental impacts.
Case Study 1: Antimicrobial Efficacy
In a controlled laboratory setting, BMPC was tested for its antimicrobial properties against Listeria monocytogenes. The results showed that BMPC effectively reduced bacterial counts by over 99% within 24 hours at concentrations as low as 1 mg/mL. This study underscores the potential use of BMPC as a natural preservative in food products.
Case Study 2: Cytotoxic Effects on Cancer Cells
A study investigating the effects of BMPC on MCF-7 cells demonstrated that treatment with 75 µM BMPC resulted in a significant reduction in cell viability (approximately 70%) compared to untreated controls. Flow cytometry analysis indicated that BMPC induced apoptosis, suggesting its potential as an anti-cancer agent.
Data Tables
Biological Activity | Test Organism/Cell Line | Effect Observed | Concentration Range |
---|---|---|---|
Antimicrobial | E. coli | Inhibition of growth | MIC: 0.5 - 2.0 mg/mL |
Antimicrobial | S. aureus | Inhibition of growth | MIC: 0.5 - 2.0 mg/mL |
Cytotoxicity | HepG2 | IC: 50 - 100 µM | Dose-dependent |
Cytotoxicity | MCF-7 | IC: 50 - 100 µM | Dose-dependent |
Ecotoxicity | Aquatic organisms | Toxicity observed | Elevated concentrations |
Research Findings
Recent studies have expanded our understanding of the biological activity of BMPC:
- Antimicrobial Mechanisms : Research indicates that BMPC disrupts microbial membranes through electrostatic interactions, leading to increased permeability and eventual cell death .
- Cytotoxic Mechanisms : The induction of oxidative stress is a primary pathway through which BMPC exerts cytotoxic effects on cancer cells, leading to DNA damage and apoptosis .
- Environmental Behavior : Investigations into the environmental persistence of BMPC suggest that it degrades more slowly than traditional solvents, raising concerns about its long-term ecological impact .
Eigenschaften
IUPAC Name |
1-butyl-3-methylpyridin-1-ium;chloride | |
---|---|---|
Source | PubChem | |
URL | https://pubchem.ncbi.nlm.nih.gov | |
Description | Data deposited in or computed by PubChem | |
InChI |
InChI=1S/C10H16N.ClH/c1-3-4-7-11-8-5-6-10(2)9-11;/h5-6,8-9H,3-4,7H2,1-2H3;1H/q+1;/p-1 | |
Source | PubChem | |
URL | https://pubchem.ncbi.nlm.nih.gov | |
Description | Data deposited in or computed by PubChem | |
InChI Key |
PHCASOSWUQOQAG-UHFFFAOYSA-M | |
Source | PubChem | |
URL | https://pubchem.ncbi.nlm.nih.gov | |
Description | Data deposited in or computed by PubChem | |
Canonical SMILES |
CCCC[N+]1=CC=CC(=C1)C.[Cl-] | |
Source | PubChem | |
URL | https://pubchem.ncbi.nlm.nih.gov | |
Description | Data deposited in or computed by PubChem | |
Molecular Formula |
C10H16ClN | |
Source | PubChem | |
URL | https://pubchem.ncbi.nlm.nih.gov | |
Description | Data deposited in or computed by PubChem | |
DSSTOX Substance ID |
DTXSID2049212 | |
Record name | 1-Butyl-3-methylpyridinium chloride | |
Source | EPA DSSTox | |
URL | https://comptox.epa.gov/dashboard/DTXSID2049212 | |
Description | DSSTox provides a high quality public chemistry resource for supporting improved predictive toxicology. | |
Molecular Weight |
185.69 g/mol | |
Source | PubChem | |
URL | https://pubchem.ncbi.nlm.nih.gov | |
Description | Data deposited in or computed by PubChem | |
CAS No. |
125652-55-3 | |
Record name | 1-Butyl-3-methylpyridinium chloride | |
Source | EPA DSSTox | |
URL | https://comptox.epa.gov/dashboard/DTXSID2049212 | |
Description | DSSTox provides a high quality public chemistry resource for supporting improved predictive toxicology. | |
Record name | 1-Butyl-3-methylpyridinium Chloride | |
Source | European Chemicals Agency (ECHA) | |
URL | https://echa.europa.eu/information-on-chemicals | |
Description | The European Chemicals Agency (ECHA) is an agency of the European Union which is the driving force among regulatory authorities in implementing the EU's groundbreaking chemicals legislation for the benefit of human health and the environment as well as for innovation and competitiveness. | |
Explanation | Use of the information, documents and data from the ECHA website is subject to the terms and conditions of this Legal Notice, and subject to other binding limitations provided for under applicable law, the information, documents and data made available on the ECHA website may be reproduced, distributed and/or used, totally or in part, for non-commercial purposes provided that ECHA is acknowledged as the source: "Source: European Chemicals Agency, http://echa.europa.eu/". Such acknowledgement must be included in each copy of the material. ECHA permits and encourages organisations and individuals to create links to the ECHA website under the following cumulative conditions: Links can only be made to webpages that provide a link to the Legal Notice page. | |
Retrosynthesis Analysis
AI-Powered Synthesis Planning: Our tool employs the Template_relevance Pistachio, Template_relevance Bkms_metabolic, Template_relevance Pistachio_ringbreaker, Template_relevance Reaxys, Template_relevance Reaxys_biocatalysis model, leveraging a vast database of chemical reactions to predict feasible synthetic routes.
One-Step Synthesis Focus: Specifically designed for one-step synthesis, it provides concise and direct routes for your target compounds, streamlining the synthesis process.
Accurate Predictions: Utilizing the extensive PISTACHIO, BKMS_METABOLIC, PISTACHIO_RINGBREAKER, REAXYS, REAXYS_BIOCATALYSIS database, our tool offers high-accuracy predictions, reflecting the latest in chemical research and data.
Strategy Settings
Precursor scoring | Relevance Heuristic |
---|---|
Min. plausibility | 0.01 |
Model | Template_relevance |
Template Set | Pistachio/Bkms_metabolic/Pistachio_ringbreaker/Reaxys/Reaxys_biocatalysis |
Top-N result to add to graph | 6 |
Feasible Synthetic Routes
Q1: How does 1-butyl-3-methylpyridinium chloride enhance the hydrolysis of cellulose?
A: 1-butyl-3-methylpyridinium chloride ([BMP]Cl) acts as a reaction medium in cellulose hydrolysis alongside cellulase. [] Research suggests that [BMP]Cl enhances the hydrolysis kinetics by increasing the initial hydrolysis rates compared to untreated cellulose. [] This enhancement is attributed to the synergistic effects between [BMP]Cl and cellulase on the cellulose material. [] Further research is needed to fully elucidate the mechanism behind this synergistic effect.
Q2: Can you explain the role of 1-butyl-3-methylpyridinium chloride in separating hydrophobic compounds and provide an example?
A: 1-butyl-3-methylpyridinium chloride can be used as an adjuvant in Ethanolic Two-Phase Systems (ETPS) to separate hydrophobic compounds. [] For example, in an ETPS composed of polypropylene glycol 2000, ethylene glycol, ethanol, and 1-butyl-3-methylpyridinium chloride, the hydrophobic compound bixin preferentially migrates to the polypropylene glycol 2000-rich phase. [] This demonstrates the potential of using 1-butyl-3-methylpyridinium chloride-based ETPS for extracting and separating hydrophobic compounds from complex mixtures.
Q3: How does 1-butyl-3-methylpyridinium chloride impact the performance and stability of lead-free perovskite solar cells?
A: Incorporating 1-butyl-3-methylpyridinium chloride into lead-free Cs2AgBiBr6 perovskite solar cells has been shown to improve both their efficiency and long-term stability. [] The mechanism involves the ionic liquid's ability to inhibit bromide ion (Br–) migration, a significant factor limiting the operational lifespan of these solar cells. [] By mitigating Br– migration, 1-butyl-3-methylpyridinium chloride reduces film defects and enhances energy level matching, ultimately leading to improved power conversion efficiency and prolonged stability even under ambient humidity. []
Q4: What are the environmental concerns related to using ionic liquids like 1-butyl-3-methylpyridinium chloride, and are there any strategies for mitigating their impact?
A: While this specific research does not delve into the environmental impact of 1-butyl-3-methylpyridinium chloride, it is crucial to acknowledge that ionic liquids, in general, can pose environmental risks. Their toxicity, biodegradability, and potential for accumulation in the environment need to be carefully considered. [, ] Research on the development of less toxic ionic liquids, biodegradable options, and effective recycling and waste management strategies is crucial for ensuring the sustainable use of these compounds.
Haftungsausschluss und Informationen zu In-Vitro-Forschungsprodukten
Bitte beachten Sie, dass alle Artikel und Produktinformationen, die auf BenchChem präsentiert werden, ausschließlich zu Informationszwecken bestimmt sind. Die auf BenchChem zum Kauf angebotenen Produkte sind speziell für In-vitro-Studien konzipiert, die außerhalb lebender Organismen durchgeführt werden. In-vitro-Studien, abgeleitet von dem lateinischen Begriff "in Glas", beinhalten Experimente, die in kontrollierten Laborumgebungen unter Verwendung von Zellen oder Geweben durchgeführt werden. Es ist wichtig zu beachten, dass diese Produkte nicht als Arzneimittel oder Medikamente eingestuft sind und keine Zulassung der FDA für die Vorbeugung, Behandlung oder Heilung von medizinischen Zuständen, Beschwerden oder Krankheiten erhalten haben. Wir müssen betonen, dass jede Form der körperlichen Einführung dieser Produkte in Menschen oder Tiere gesetzlich strikt untersagt ist. Es ist unerlässlich, sich an diese Richtlinien zu halten, um die Einhaltung rechtlicher und ethischer Standards in Forschung und Experiment zu gewährleisten.