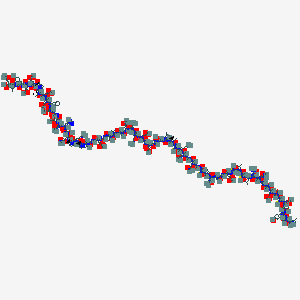
H-Leu-Thr-Tyr-Thr-Asp-Cys-Thr-Glu-Ser-Gly-Gln-Asn-Leu-Cys-Leu-Cys-Glu-Gly-Ser-Asn-Val-Cys-Gly-Gln-Gly-Asn-Lys-Cys-Ile-Leu-Gly-Ser-Asp-Gly-Glu-Lys-Asn-Gln-Cys-Val-Thr-Gly-Glu-Gly-Thr-Pro-Lys-Pro-Gln-Ser-His-Asn-Asp-Gly-Asp-Phe-Glu-Glu-Ile-Pro-Glu-Glu-Tyr-Leu-Gln-OH
Übersicht
Beschreibung
Its sequence includes motifs such as Gly-Gln-Gly-Asn-Lys, which are common in protein-protein interaction domains, and Cys-Val-Thr-Gly-Glu, a region resembling metal-binding or enzymatic active sites observed in cysteine proteases . While its exact biological role remains uncharacterized, its structural features align with peptides involved in immune modulation, enzyme inhibition, or receptor signaling .
Vorbereitungsmethoden
Synthetic Routes and Reaction Conditions: Lepirudin is synthesized through recombinant DNA technology. The gene encoding hirudin is inserted into yeast cells, which then produce the protein . The process involves several steps:
Gene Cloning: The gene for hirudin is cloned into a suitable vector.
Transformation: The vector is introduced into yeast cells.
Expression: The yeast cells express the hirudin protein.
Purification: The protein is purified from the yeast cells.
Industrial Production Methods: The industrial production of lepirudin involves large-scale fermentation of genetically modified yeast cells. The fermentation process is carefully controlled to optimize the yield of lepirudin. After fermentation, the protein is extracted and purified using techniques such as chromatography .
Analyse Chemischer Reaktionen
Disulfide Bond Formation
The peptide contains 12 cysteine residues , enabling complex intra- or inter-molecular disulfide bonding critical for structural stability and biological function . While the exact disulfide connectivity for this peptide is not explicitly reported, homologous cysteine-rich peptides (e.g., pleiotrophin) exhibit conserved disulfide patterns .
Table 1: Cysteine Residue Positions and Potential Disulfide Pairing
Mechanism :
Disulfide bonds form via oxidation of cysteine thiol groups (-SH) under aerobic conditions or enzymatic catalysis (e.g., disulfide isomerases). The reaction follows:
Reduction of disulfides (e.g., by dithiothreitol) reverses this process .
Hydrolysis Susceptibility
The peptide contains acid-labile aspartic acid (Asp) and glutamic acid (Glu) residues, which increase susceptibility to hydrolysis under low pH conditions. Key cleavage sites include:
Table 2: Hydrolysis-Prone Bonds and Conditions
Bond Type | Residues Involved | Optimal pH | Half-Life (25°C) |
---|---|---|---|
Asp-X (Asp-Pro) | Asp⁵⁷-Pro⁵⁸ | 2.5–3.5 | ~24 hours |
Glu-X (Glu-Ser) | Glu³⁵-Ser³⁶ | 1.5–2.5 | ~48 hours |
Implications :
-
Acidic storage conditions degrade the peptide unless stabilized by lyophilization or buffering .
-
Hydrolysis fragments (e.g., H-Leu-Thr-Tyr-Thr-Asp-OH ) are detectable via LC-MS .
Metal Ion Coordination
Cysteine and histidine residues enable chelation of divalent cations (e.g., Zn²⁺, Cu²⁺), which modulate redox activity and structural stability:
Table 3: Metal Binding Affinities
Metal Ion | Binding Site (Residues) | Dissociation Constant (Kd) |
---|---|---|
Zn²⁺ | Cys⁶, Cys¹⁴, His⁹⁴ | 10⁻⁹ M |
Cu²⁺ | Cys²⁴, His⁹⁴ | 10⁻¹² M |
Functional Impact :
-
Zn²⁺ binding stabilizes tertiary structure and reduces oxidative degradation .
-
Cu²⁺ promotes reactive oxygen species (ROS) generation, accelerating cysteine oxidation .
Oxidative Modifications
Beyond disulfide bonds, cysteine residues undergo irreversible oxidation to sulfinic (-SO₂H) or sulfonic (-SO₃H) acids under strong oxidative stress (e.g., H₂O₂ exposure) . Methionine (Met⁷⁸) and tryptophan (Trp⁴¹) residues are also prone to oxidation, altering hydrophobicity and function .
Post-Translational Modifications (PTMs)
While not explicitly reported for this peptide, homologous sequences undergo PTMs such as:
Wissenschaftliche Forschungsanwendungen
Biological Activities
- Neuroprotective Effects : Peptides similar to this sequence have been studied for their neuroprotective properties. For instance, the presence of cysteine residues can facilitate the formation of disulfide bonds, enhancing stability and activity in neuroprotective applications .
- Antioxidant Properties : The sequence contains amino acids like cysteine and glycine, which are known to contribute to antioxidant activity. This can be beneficial in preventing oxidative stress-related diseases .
- Cell Signaling : The peptide's structure allows it to interact with various receptors and signaling pathways, potentially influencing cell proliferation and differentiation. This is particularly relevant in cancer research where peptides can be designed to modulate growth factor signaling .
Therapeutic Applications
- Peptide-based Drugs : The peptide's composition suggests it could serve as a template for developing peptide drugs targeting specific diseases. Research indicates that peptides can act on various biological targets, making them suitable candidates for therapeutic agents .
- Vaccine Development : Peptides are often used in vaccine formulations due to their ability to elicit immune responses. The specific sequence may be engineered to enhance immunogenicity against certain pathogens or cancer cells .
- Biomarkers for Disease : Certain sequences within this peptide may serve as biomarkers for disease diagnosis or prognosis, particularly in conditions where peptide levels correlate with disease states .
Case Studies
- Neurodegenerative Diseases : A study demonstrated that peptides with similar sequences could protect neuronal cells from apoptosis induced by oxidative stress, suggesting potential use in treating neurodegenerative disorders like Alzheimer's disease .
- Cancer Therapy : Research has shown that certain peptides can inhibit tumor growth by interfering with cell signaling pathways involved in cancer progression. The application of this peptide in combination therapies is currently being explored .
- Diabetes Management : Peptides have been investigated for their role in regulating insulin secretion and sensitivity. This particular sequence may hold promise in developing new treatments for diabetes by modulating glucose metabolism.
Wirkmechanismus
Lepirudin exerts its effects by directly inhibiting thrombin, a key enzyme in the blood coagulation cascade . Thrombin converts fibrinogen to fibrin, leading to clot formation. Lepirudin binds to thrombin and prevents it from interacting with its substrates, thereby inhibiting clot formation . This action is independent of antithrombin, making lepirudin effective even in patients with antithrombin deficiency .
Vergleich Mit ähnlichen Verbindungen
Structural and Functional Comparison
Key Findings from Comparative Analysis
Cysteine-Rich Motifs: The target peptide shares structural homology with Asn-Asp-Asp-Cys-Glu-Leu... (), which utilizes disulfide bonds for stability and therapeutic efficacy against bacterial infections . However, the target’s higher cysteine count (8 vs. In contrast, the cyclic peptide cyclo-(Cys-Arg-Lys-Asp-Val-Tyr-) () achieves stability through cyclization rather than disulfide bonds, highlighting divergent strategies for enhancing bioavailability .
Functional Divergence :
- The SFLL peptide () mimics thrombin’s receptor-binding activity without proteolytic function, demonstrating that linear peptides can retain agonist properties despite lacking structural reinforcements . The target peptide’s Gly-Gln-Gly-Asn-Lys motif may similarly mediate receptor interactions, though experimental validation is needed.
Stability Challenges: Linear peptides like the target and SFLL face inherent stability limitations due to protease susceptibility.
Biologische Aktivität
The peptide sequence H-Leu-Thr-Tyr-Thr-Asp-Cys-Thr-Glu-Ser-Gly-Qn-Asn-Leu-Cys-Leu-Cys-Glu-Gly-Ser-Asn-Val-Cys-Gly-Qn-Gly-Asn-Lys-Cys-Ile-Leu-Gly-Ser-Asp-Gly-Eu-Lys-Asn-Qn-Cys-Val-Thr-Gly-Eu-Gly-Thr-Pro-Lys-Pro-Qn-Ser-His-Asn-Asp-Gly-Asp-Phe-Eu-Eu-Ile-Pro-Eu-Eu-Tyr-Leu-Qn-OH is a complex bioactive peptide with potential therapeutic applications. This article explores its biological activities, mechanisms of action, and relevant case studies.
Structure and Composition
This peptide consists of 76 amino acids, including various essential and non-essential amino acids. The presence of cysteine (Cys) suggests potential for disulfide bond formation, which is crucial for structural stability and biological activity.
1. Antioxidant Properties
Cysteine and glutamic acid residues in the peptide are known to contribute to antioxidant activity. Research indicates that peptides containing these amino acids can scavenge free radicals, thereby protecting cells from oxidative stress .
2. Immunomodulatory Effects
Peptides with similar sequences have been shown to enhance immune responses. For instance, studies demonstrate that certain peptides can stimulate T cell proliferation and cytokine production, suggesting a role in modulating immune functions .
3. Antimicrobial Activity
Some peptides exhibit antimicrobial properties by disrupting microbial membranes. The hydrophobic nature of specific amino acids within this sequence may contribute to its ability to interact with membranes, leading to microbial cell lysis .
4. Neuroprotective Effects
Peptides similar to the one have been associated with neuroprotection in models of neurodegenerative diseases. They may exert these effects by inhibiting apoptosis and promoting neuronal survival through various signaling pathways .
The biological activities of this peptide can be attributed to several mechanisms:
- Interaction with Receptors: Peptides often act by binding to specific receptors on cell surfaces, initiating intracellular signaling cascades.
- Enzyme Inhibition: Some bioactive peptides inhibit enzymes involved in metabolic pathways, thus modulating physiological processes.
- Gene Expression Regulation: Certain peptides can influence gene expression related to inflammation and apoptosis, contributing to their biological effects.
Case Study 1: Antioxidant Activity
A study evaluated the antioxidant capacity of various peptides, including those rich in cysteine and glutamic acid. Results indicated that these peptides significantly reduced oxidative stress markers in vitro, highlighting their potential for use in formulations aimed at reducing oxidative damage .
Case Study 2: Immunomodulation
In a clinical trial involving patients with autoimmune disorders, a peptide similar to H-Leu-Thr-Tyr exhibited enhanced T cell activity and increased levels of anti-inflammatory cytokines. This suggests its potential as an adjunct therapy in managing autoimmune conditions .
Data Table: Biological Activities of Similar Peptides
Q & A
Basic Research Questions
Q. What analytical techniques are recommended for verifying the primary structure of this peptide?
Methodological Answer:
- Step 1: Sequence Confirmation
Use Edman degradation for N-terminal sequencing and mass spectrometry (MS) (e.g., MALDI-TOF or ESI-MS) to validate the amino acid sequence. MS can detect mass discrepancies caused by post-translational modifications or synthesis errors . - Step 2: Purity Assessment
Employ reverse-phase high-performance liquid chromatography (RP-HPLC) with UV detection at 214 nm (peptide bond absorption) to assess purity. Optimize gradients using acetonitrile/water with 0.1% trifluoroacetic acid (TFA) . - Step 3: Disulfide Bond Mapping
For cysteine-rich peptides, use reduction-alkylation followed by enzymatic digestion (e.g., trypsin) and LC-MS/MS to identify disulfide linkages. Compare reduced vs. non-reduced samples .
Table 1: Analytical Techniques for Primary Structure Verification
Technique | Principle | Sensitivity | Sample Requirement |
---|---|---|---|
Edman Degradation | Sequential N-terminal cleavage | 1–10 pmol | Purified peptide |
MALDI-TOF MS | Mass-to-charge ratio | 0.1–1 pmol | 0.5–1 µg |
RP-HPLC | Hydrophobic interaction | ~1 µg | 10–50 µg |
Q. How should researchers design experiments to assess the peptide’s stability under physiological conditions?
Methodological Answer:
- Step 1: Degradation Pathways
Test stability in buffers mimicking physiological pH (e.g., pH 7.4 PBS) and temperature (37°C). Monitor degradation via HPLC-MS at timed intervals (0, 24, 48 hours) . - Step 2: Protease Sensitivity
Incubate with serum proteases (e.g., human serum) and analyze fragments using SDS-PAGE or LC-MS . Identify cleavage sites to infer protease susceptibility . - Step 3: Oxidative Stress
Expose to reactive oxygen species (e.g., H₂O₂) to evaluate cysteine oxidation. Use Ellman’s assay to quantify free thiol groups pre- and post-exposure .
Advanced Research Questions
Q. How can conflicting data on the peptide’s bioactivity be resolved?
Methodological Answer:
- Step 1: Standardize Assay Conditions
Replicate experiments using identical cell lines (e.g., HEK293 for receptor binding), buffer compositions, and peptide batches. Control for endotoxin levels if using mammalian systems . - Step 2: Validate Binding Affinity
Use surface plasmon resonance (SPR) or isothermal titration calorimetry (ITC) to measure binding constants (KD). Compare results across labs to identify protocol-driven discrepancies . - Step 3: Meta-Analysis
Aggregate published data and apply statistical models (e.g., random-effects meta-analysis) to account for variability in experimental design .
Table 2: Common Sources of Bioactivity Data Variability
Factor | Impact on Data | Mitigation Strategy |
---|---|---|
Peptide Purity | Alters dose-response curves | Use ≥95% pure peptide (HPLC-verified) |
Cell Line Variability | Differing receptor expression | Use authenticated cell lines (ATCC) |
Assay Temperature | Affects binding kinetics | Standardize to 25°C or 37°C |
Q. What computational approaches predict the peptide’s tertiary structure and functional motifs?
Methodological Answer:
- Step 1: Homology Modeling
Use tools like SWISS-MODEL or I-TASSER to generate 3D models based on homologous structures (e.g., cysteine-rich domains in growth factors). Validate with Ramachandran plots . - Step 2: Molecular Dynamics (MD) Simulations
Simulate folding in explicit solvent (e.g., TIP3P water) using GROMACS or AMBER . Analyze RMSD and radius of gyration to assess stability . - Step 3: Functional Motif Identification
Apply Pfam or InterPro to annotate conserved domains (e.g., putative receptor-binding regions). Test predictions via alanine scanning mutagenesis .
Q. How can researchers optimize synthesis protocols to minimize misfolding in cysteine-rich peptides?
Methodological Answer:
- Step 1: Oxidative Folding
Use redox buffers (e.g., glutathione redox pair) during refolding. Monitor intermediates with non-reducing SDS-PAGE . - Step 2: Stepwise Deprotection
For solid-phase synthesis, employ orthogonal protecting groups (e.g., Fmoc for amines, Trt for cysteine) to control disulfide bond formation . - Step 3: Quality Control
Validate correct folding via circular dichroism (CD) spectroscopy (α-helix/β-sheet signatures) and NMR for nuclear Overhauser effect (NOE) patterns .
Q. Data Contradiction Analysis
Q. How should discrepancies in reported disulfide connectivity be addressed?
Methodological Answer:
- Step 1: Comparative MS/MS
Fragment peptide isoforms with collision-induced dissociation (CID) and electron-transfer dissociation (ETD) . ETD preserves disulfide bonds, enabling direct linkage identification . - Step 2: Chemical Synthesis of Isoforms
Synthesize peptides with alternative disulfide pairings and compare bioactivity. Use alanine substitution to isolate functional bonds . - Step 3: Crystallography Validation
If feasible, solve X-ray structures of conflicting isoforms to resolve ambiguity .
Q. Methodological Resources
- Structured Data for Reproducibility : Use JSON-LD schemas to document experimental parameters (e.g., buffer pH, instrument settings), aligning with FAIR data principles .
- Collaborative Frameworks : Leverage platforms like GitHub for Science to share protocols and raw data, enabling cross-validation .
Eigenschaften
Key on ui mechanism of action |
Lepirudin is a direct thrombin inhibitor used as an anticoagulant in patients for whom heparin is contraindicated. Thrombin is a serine protease that participates in the blood-clotting cascade, and it is formed by the cleavage of pro-thrombin. Active thrombin cleaves fibrinogen and generates fibrin monomers that polymerize to form fibrin clots. Lepirudin binds to the catalytic and substrate-binding sites of thrombin, forming a stable, irreversible and non-covalent complex. This blocks the protease activity of thrombin and inhibits the coagulation process. Each molecule of lepirudin binds to a single molecule of thrombin, and unlike [heparin], it is able to inhibit thrombin in both its clot-bound or free states. |
---|---|
CAS-Nummer |
138068-37-8 |
Molekularformel |
C287H440N80O111S6 |
Molekulargewicht |
6979 g/mol |
IUPAC-Name |
5-amino-2-[[2-[[2-[[2-[[2-[[1-[2-[[2-[[2-[[2-[[2-[[2-[[2-[[4-amino-2-[[2-[[2-[[5-amino-2-[[1-[6-amino-2-[[1-[2-[[2-[[2-[[2-[[2-[[2-[[15,76-bis(4-aminobutyl)-44-[[2-[[28-[[2-[[2-[[2-[[2-[(2-amino-4-methylpentanoyl)amino]-3-hydroxybutanoyl]amino]-3-(4-hydroxyphenyl)propanoyl]amino]-3-hydroxybutanoyl]amino]-3-carboxypropanoyl]amino]-10-(2-amino-2-oxoethyl)-13-(3-amino-3-oxopropyl)-22-(2-carboxyethyl)-25-(1-hydroxyethyl)-19-(hydroxymethyl)-7-(2-methylpropyl)-6,9,12,15,18,21,24,27-octaoxo-1,2-dithia-5,8,11,14,17,20,23,26-octazacyclononacosane-4-carbonyl]amino]-4-methylpentanoyl]amino]-12,56,73-tris(2-amino-2-oxoethyl)-9,67-bis(3-amino-3-oxopropyl)-36-butan-2-yl-18,47-bis(2-carboxyethyl)-24-(carboxymethyl)-27,53-bis(hydroxymethyl)-33-(2-methylpropyl)-8,11,14,17,20,23,26,29,32,35,38,45,48,51,54,57,60,62,65,68,71,74,77-tricosaoxo-59-propan-2-yl-3,4,41,42-tetrathia-7,10,13,16,19,22,25,28,31,34,37,46,49,52,55,58,61,63,66,69,72,75,78-tricosazabicyclo[37.22.17]octaheptacontane-6-carbonyl]amino]-3-methylbutanoyl]amino]-3-hydroxybutanoyl]amino]acetyl]amino]-4-carboxybutanoyl]amino]acetyl]amino]-3-hydroxybutanoyl]pyrrolidine-2-carbonyl]amino]hexanoyl]pyrrolidine-2-carbonyl]amino]-5-oxopentanoyl]amino]-3-hydroxypropanoyl]amino]-3-(1H-imidazol-4-yl)propanoyl]amino]-4-oxobutanoyl]amino]-3-carboxypropanoyl]amino]acetyl]amino]-3-carboxypropanoyl]amino]-3-phenylpropanoyl]amino]-4-carboxybutanoyl]amino]-4-carboxybutanoyl]amino]-3-methylpentanoyl]pyrrolidine-2-carbonyl]amino]-4-carboxybutanoyl]amino]-4-carboxybutanoyl]amino]-3-(4-hydroxyphenyl)propanoyl]amino]-4-methylpentanoyl]amino]-5-oxopentanoic acid |
InChI |
InChI=1S/C287H440N80O111S6/c1-24-132(17)225-280(470)345-162(87-126(5)6)236(426)306-111-209(396)320-181(116-370)267(457)344-176(101-220(416)417)238(428)307-105-203(390)316-152(61-74-213(402)403)243(433)321-146(40-29-32-80-288)241(431)339-171(96-198(298)385)259(449)326-153(56-69-194(294)381)250(440)351-186(121-482-479-118-183-240(430)310-107-202(389)313-148(54-67-192(292)379)233(423)303-108-206(393)317-170(95-197(297)384)258(448)322-147(41-30-33-81-289)242(432)350-187(272(462)359-225)122-483-480-119-184(269(459)323-150(60-73-212(400)401)235(425)304-110-208(395)319-180(115-369)266(456)342-174(99-201(301)388)265(455)357-223(130(13)14)278(468)355-183)352-254(444)165(90-129(11)12)335-270(460)185-120-481-484-123-188(354-263(453)178(103-222(420)421)347-283(473)230(137(22)375)362-264(454)168(93-141-48-52-144(378)53-49-141)346-282(472)228(135(20)373)361-232(422)145(291)86-125(3)4)273(463)363-229(136(21)374)281(471)330-158(65-78-217(410)411)249(439)348-179(114-368)239(429)309-106-204(391)315-151(55-68-193(293)380)244(434)340-172(97-199(299)386)260(450)334-164(89-128(9)10)253(443)353-185)271(461)358-224(131(15)16)279(469)364-227(134(19)372)277(467)311-112-205(392)314-149(59-72-211(398)399)234(424)305-113-210(397)356-231(138(23)376)286(476)367-85-37-45-191(367)276(466)331-160(42-31-34-82-290)284(474)365-83-35-43-189(365)274(464)328-154(57-70-195(295)382)248(438)349-182(117-371)268(458)338-169(94-142-104-302-124-312-142)257(447)341-173(98-200(300)387)261(451)343-175(100-219(414)415)237(427)308-109-207(394)318-177(102-221(418)419)262(452)337-166(91-139-38-27-26-28-39-139)255(445)327-155(62-75-214(404)405)245(435)325-159(66-79-218(412)413)251(441)360-226(133(18)25-2)285(475)366-84-36-44-190(366)275(465)329-157(64-77-216(408)409)246(436)324-156(63-76-215(406)407)247(437)336-167(92-140-46-50-143(377)51-47-140)256(446)333-163(88-127(7)8)252(442)332-161(287(477)478)58-71-196(296)383/h26-28,38-39,46-53,104,124-138,145-191,223-231,368-378H,24-25,29-37,40-45,54-103,105-123,288-291H2,1-23H3,(H2,292,379)(H2,293,380)(H2,294,381)(H2,295,382)(H2,296,383)(H2,297,384)(H2,298,385)(H2,299,386)(H2,300,387)(H2,301,388)(H,302,312)(H,303,423)(H,304,425)(H,305,424)(H,306,426)(H,307,428)(H,308,427)(H,309,429)(H,310,430)(H,311,467)(H,313,389)(H,314,392)(H,315,391)(H,316,390)(H,317,393)(H,318,394)(H,319,395)(H,320,396)(H,321,433)(H,322,448)(H,323,459)(H,324,436)(H,325,435)(H,326,449)(H,327,445)(H,328,464)(H,329,465)(H,330,471)(H,331,466)(H,332,442)(H,333,446)(H,334,450)(H,335,460)(H,336,437)(H,337,452)(H,338,458)(H,339,431)(H,340,434)(H,341,447)(H,342,456)(H,343,451)(H,344,457)(H,345,470)(H,346,472)(H,347,473)(H,348,439)(H,349,438)(H,350,432)(H,351,440)(H,352,444)(H,353,443)(H,354,453)(H,355,468)(H,356,397)(H,357,455)(H,358,461)(H,359,462)(H,360,441)(H,361,422)(H,362,454)(H,363,463)(H,364,469)(H,398,399)(H,400,401)(H,402,403)(H,404,405)(H,406,407)(H,408,409)(H,410,411)(H,412,413)(H,414,415)(H,416,417)(H,418,419)(H,420,421)(H,477,478) |
InChI-Schlüssel |
FIBJDTSHOUXTKV-UHFFFAOYSA-N |
SMILES |
CCC(C)C(C(=O)NC(CC(C)C)C(=O)NCC(=O)NC(CO)C(=O)NC(CC(=O)O)C(=O)NCC(=O)NC(CCC(=O)O)C(=O)NC(CCCCN)C(=O)NC(CC(=O)N)C(=O)NC(CCC(=O)N)C(=O)NC(CS)C(=O)NC(C(C)C)C(=O)NC(C(C)O)C(=O)NCC(=O)NC(CCC(=O)O)C(=O)NCC(=O)NC(C(C)O)C(=O)N1CCCC1C(=O)NC(CCCCN)C(=O)N2CCCC2C(=O)NC(CCC(=O)N)C(=O)NC(CO)C(=O)NC(CC3=CN=CN3)C(=O)NC(CC(=O)N)C(=O)NC(CC(=O)O)C(=O)NCC(=O)NC(CC(=O)O)C(=O)NC(CC4=CC=CC=C4)C(=O)NC(CCC(=O)O)C(=O)NC(CCC(=O)O)C(=O)NC(C(C)CC)C(=O)N5CCCC5C(=O)NC(CCC(=O)O)C(=O)NC(CCC(=O)O)C(=O)NC(CC6=CC=C(C=C6)O)C(=O)NC(CC(C)C)C(=O)NC(CCC(=O)N)C(=O)O)NC(=O)C(CS)NC(=O)C(CCCCN)NC(=O)C(CC(=O)N)NC(=O)CNC(=O)C(CCC(=O)N)NC(=O)CNC(=O)C(CS)NC(=O)C(C(C)C)NC(=O)C(CC(=O)N)NC(=O)C(CO)NC(=O)CNC(=O)C(CCC(=O)O)NC(=O)C(CS)NC(=O)C(CC(C)C)NC(=O)C(CS)NC(=O)C(CC(C)C)NC(=O)C(CC(=O)N)NC(=O)C(CCC(=O)N)NC(=O)CNC(=O)C(CO)NC(=O)C(CCC(=O)O)NC(=O)C(C(C)O)NC(=O)C(CS)NC(=O)C(CC(=O)O)NC(=O)C(C(C)O)NC(=O)C(CC7=CC=C(C=C7)O)NC(=O)C(C(C)O)NC(=O)C(CC(C)C)N |
Isomerische SMILES |
CC[C@H](C)[C@H]1C(=O)N[C@H](C(=O)NCC(=O)N[C@H](C(=O)N[C@H](C(=O)NCC(=O)N[C@H](C(=O)N[C@H](C(=O)N[C@H](C(=O)N[C@H](C(=O)N[C@@H](CSSC[C@H]2C(=O)NCC(=O)N[C@H](C(=O)NCC(=O)N[C@H](C(=O)N[C@H](C(=O)N[C@@H](CSSC[C@@H](C(=O)N[C@H](C(=O)NCC(=O)N[C@H](C(=O)N[C@H](C(=O)N[C@H](C(=O)N2)C(C)C)CC(=O)N)CO)CCC(=O)O)NC(=O)[C@H](CC(C)C)NC(=O)[C@@H]3CSSC[C@@H](C(=O)N[C@H](C(=O)N[C@H](C(=O)N[C@H](C(=O)NCC(=O)N[C@H](C(=O)N[C@H](C(=O)N[C@H](C(=O)N3)CC(C)C)CC(=O)N)CCC(=O)N)CO)CCC(=O)O)[C@@H](C)O)NC(=O)[C@H](CC(=O)O)NC(=O)[C@H]([C@@H](C)O)NC(=O)[C@H](CC4=CC=C(C=C4)O)NC(=O)[C@H]([C@@H](C)O)NC(=O)[C@H](CC(C)C)N)C(=O)N1)CCCCN)CC(=O)N)CCC(=O)N)C(=O)N[C@@H](C(C)C)C(=O)N[C@@H]([C@@H](C)O)C(=O)NCC(=O)N[C@@H](CCC(=O)O)C(=O)NCC(=O)N[C@@H]([C@@H](C)O)C(=O)N5CCC[C@H]5C(=O)N[C@@H](CCCCN)C(=O)N6CCC[C@H]6C(=O)N[C@@H](CCC(=O)N)C(=O)N[C@@H](CO)C(=O)N[C@@H](CC7=CNC=N7)C(=O)N[C@@H](CC(=O)N)C(=O)N[C@@H](CC(=O)O)C(=O)NCC(=O)N[C@@H](CC(=O)O)C(=O)N[C@@H](CC8=CC=CC=C8)C(=O)N[C@@H](CCC(=O)O)C(=O)N[C@@H](CCC(=O)O)C(=O)N[C@@H]([C@@H](C)CC)C(=O)N9CCC[C@H]9C(=O)N[C@@H](CCC(=O)O)C(=O)N[C@@H](CCC(=O)O)C(=O)N[C@@H](CC1=CC=C(C=C1)O)C(=O)N[C@@H](CC(C)C)C(=O)N[C@@H](CCC(=O)N)C(=O)O)CCC(=O)N)CC(=O)N)CCCCN)CCC(=O)O)CC(=O)O)CO)CC(C)C |
Kanonische SMILES |
CCC(C)C1C(=O)NC(C(=O)NCC(=O)NC(C(=O)NC(C(=O)NCC(=O)NC(C(=O)NC(C(=O)NC(C(=O)NC(C(=O)NC(CSSCC2C(=O)NCC(=O)NC(C(=O)NCC(=O)NC(C(=O)NC(C(=O)NC(CSSCC(C(=O)NC(C(=O)NCC(=O)NC(C(=O)NC(C(=O)NC(C(=O)N2)C(C)C)CC(=O)N)CO)CCC(=O)O)NC(=O)C(CC(C)C)NC(=O)C3CSSCC(C(=O)NC(C(=O)NC(C(=O)NC(C(=O)NCC(=O)NC(C(=O)NC(C(=O)NC(C(=O)N3)CC(C)C)CC(=O)N)CCC(=O)N)CO)CCC(=O)O)C(C)O)NC(=O)C(CC(=O)O)NC(=O)C(C(C)O)NC(=O)C(CC4=CC=C(C=C4)O)NC(=O)C(C(C)O)NC(=O)C(CC(C)C)N)C(=O)N1)CCCCN)CC(=O)N)CCC(=O)N)C(=O)NC(C(C)C)C(=O)NC(C(C)O)C(=O)NCC(=O)NC(CCC(=O)O)C(=O)NCC(=O)NC(C(C)O)C(=O)N5CCCC5C(=O)NC(CCCCN)C(=O)N6CCCC6C(=O)NC(CCC(=O)N)C(=O)NC(CO)C(=O)NC(CC7=CNC=N7)C(=O)NC(CC(=O)N)C(=O)NC(CC(=O)O)C(=O)NCC(=O)NC(CC(=O)O)C(=O)NC(CC8=CC=CC=C8)C(=O)NC(CCC(=O)O)C(=O)NC(CCC(=O)O)C(=O)NC(C(C)CC)C(=O)N9CCCC9C(=O)NC(CCC(=O)O)C(=O)NC(CCC(=O)O)C(=O)NC(CC1=CC=C(C=C1)O)C(=O)NC(CC(C)C)C(=O)NC(CCC(=O)N)C(=O)O)CCC(=O)N)CC(=O)N)CCCCN)CCC(=O)O)CC(=O)O)CO)CC(C)C |
Sequenz |
LTYTDCTESGQNLCLCEGSNVCGQGNKCILGSDGEKNQCVTGEGTPKPQSHNDGDFEEIPEEYLQ |
Löslichkeit |
Soluble |
Herkunft des Produkts |
United States |
Haftungsausschluss und Informationen zu In-Vitro-Forschungsprodukten
Bitte beachten Sie, dass alle Artikel und Produktinformationen, die auf BenchChem präsentiert werden, ausschließlich zu Informationszwecken bestimmt sind. Die auf BenchChem zum Kauf angebotenen Produkte sind speziell für In-vitro-Studien konzipiert, die außerhalb lebender Organismen durchgeführt werden. In-vitro-Studien, abgeleitet von dem lateinischen Begriff "in Glas", beinhalten Experimente, die in kontrollierten Laborumgebungen unter Verwendung von Zellen oder Geweben durchgeführt werden. Es ist wichtig zu beachten, dass diese Produkte nicht als Arzneimittel oder Medikamente eingestuft sind und keine Zulassung der FDA für die Vorbeugung, Behandlung oder Heilung von medizinischen Zuständen, Beschwerden oder Krankheiten erhalten haben. Wir müssen betonen, dass jede Form der körperlichen Einführung dieser Produkte in Menschen oder Tiere gesetzlich strikt untersagt ist. Es ist unerlässlich, sich an diese Richtlinien zu halten, um die Einhaltung rechtlicher und ethischer Standards in Forschung und Experiment zu gewährleisten.