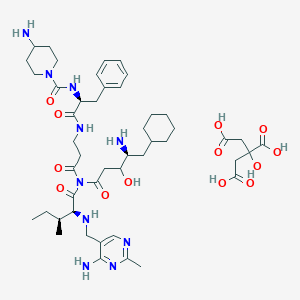
5-(4-Aminopiperidyl-1-carbonyl)-L-2,6-phenylalanyl-beta-alanyl-(4S-amino-3S-hydroxy-5-cyclohexyl)-pentancarbonyl-L-isoleucyl-aminomethyl-4-amino-2-methylpyrimidine citrate
- Klicken Sie auf QUICK INQUIRY, um ein Angebot von unserem Expertenteam zu erhalten.
- Mit qualitativ hochwertigen Produkten zu einem WETTBEWERBSFÄHIGEN Preis können Sie sich mehr auf Ihre Forschung konzentrieren.
Übersicht
Beschreibung
EMD-56133 ist ein niedermolekulares Medikament, das als Renin-Inhibitor wirkt. Renin ist ein Enzym, das an der Regulation des Blutdrucks und des Elektrolythaushalts beteiligt ist. Durch die Hemmung von Renin kann EMD-56133 möglicherweise zur Behandlung von Erkrankungen wie Bluthochdruck und Herzkreislauferkrankungen eingesetzt werden .
Herstellungsmethoden
Synthesewege und Reaktionsbedingungen
Die Synthese von EMD-56133 umfasst mehrere Schritte, darunter die Bildung von Peptidbindungen und die Einarbeitung spezifischer funktioneller Gruppen. Die Verbindung wird durch eine Reihe von chemischen Reaktionen synthetisiert, die die Verwendung verschiedener Reagenzien und Katalysatoren beinhalten. Der genaue Syntheseweg und die Reaktionsbedingungen sind proprietär und wurden von Merck KGaA entwickelt .
Industrielle Produktionsmethoden
Die industrielle Produktion von EMD-56133 beinhaltet die Hochskalierung der Laborsynthese auf einen größeren Maßstab. Dies erfordert die Optimierung der Reaktionsbedingungen, um eine hohe Ausbeute und Reinheit des Endprodukts zu gewährleisten. Der Produktionsprozess umfasst auch Reinigungsschritte wie Chromatographie, um Verunreinigungen zu entfernen und die gewünschte Verbindung zu erhalten .
Vorbereitungsmethoden
Synthetic Routes and Reaction Conditions
The synthesis of EMD-56133 involves multiple steps, including the formation of peptide bonds and the incorporation of specific functional groups. The compound is synthesized through a series of chemical reactions that involve the use of various reagents and catalysts. The exact synthetic route and reaction conditions are proprietary and have been developed by Merck KGaA .
Industrial Production Methods
Industrial production of EMD-56133 involves scaling up the laboratory synthesis to a larger scale. This requires optimization of reaction conditions to ensure high yield and purity of the final product. The production process also involves purification steps such as chromatography to remove impurities and obtain the desired compound .
Analyse Chemischer Reaktionen
Arten von Reaktionen
EMD-56133 unterliegt verschiedenen Arten von chemischen Reaktionen, darunter:
Oxidation: Die Verbindung kann unter bestimmten Bedingungen oxidiert werden, um oxidierte Derivate zu bilden.
Reduktion: EMD-56133 kann reduziert werden, um reduzierte Derivate zu bilden.
Substitution: Die Verbindung kann Substitutionsreaktionen eingehen, bei denen spezifische funktionelle Gruppen durch andere Gruppen ersetzt werden.
Häufige Reagenzien und Bedingungen
Oxidation: Häufige Oxidationsmittel wie Wasserstoffperoxid oder Kaliumpermanganat können verwendet werden.
Reduktion: Reduktionsmittel wie Natriumborhydrid oder Lithiumaluminiumhydrid werden häufig verwendet.
Substitution: Verschiedene Nukleophile und Elektrophile können je nach gewünschter Substitution verwendet werden.
Hauptprodukte, die gebildet werden
Die Hauptprodukte, die aus diesen Reaktionen gebildet werden, hängen von den spezifischen Bedingungen und Reagenzien ab, die verwendet werden. So kann die Oxidation beispielsweise oxidierte Derivate mit zusätzlichen Sauerstoffatomen ergeben, während die Reduktion reduzierte Derivate mit weniger Sauerstoffatomen ergeben kann .
Wissenschaftliche Forschungsanwendungen
EMD-56133 hat mehrere Anwendungen in der wissenschaftlichen Forschung, darunter:
Chemie: Wird als Werkzeug verwendet, um die Renin-Hemmung und ihre Auswirkungen auf biochemische Stoffwechselwege zu untersuchen.
Biologie: Untersucht wird seine Rolle bei der Regulation des Blutdrucks und des Elektrolythaushalts.
Medizin: Potenzieller therapeutischer Wirkstoff zur Behandlung von Bluthochdruck und Herzkreislauferkrankungen.
Industrie: Wird bei der Entwicklung neuer Renin-Inhibitoren und verwandter Verbindungen verwendet.
Wirkmechanismus
EMD-56133 entfaltet seine Wirkung, indem es die Aktivität von Renin hemmt, einem Enzym, das die Umwandlung von Angiotensinogen in Angiotensin I katalysiert. Durch die Hemmung von Renin reduziert EMD-56133 die Produktion von Angiotensin II, einem starken Vasokonstriktor, der den Blutdruck erhöht. Diese Hemmung führt zu einem Blutdruckabfall und einer verbesserten Herz-Kreislauf-Funktion .
Wirkmechanismus
EMD-56133 exerts its effects by inhibiting the activity of renin, an enzyme that catalyzes the conversion of angiotensinogen to angiotensin I. By inhibiting renin, EMD-56133 reduces the production of angiotensin II, a potent vasoconstrictor that increases blood pressure. This inhibition leads to a decrease in blood pressure and improved cardiovascular function .
Vergleich Mit ähnlichen Verbindungen
Ähnliche Verbindungen
Aliskiren: Ein weiterer Renin-Inhibitor zur Behandlung von Bluthochdruck.
Remikiren: Ein Renin-Inhibitor mit ähnlichen Wirkmechanismen.
Einzigartigkeit
EMD-56133 ist einzigartig in seiner spezifischen Molekülstruktur und Bindungsaffinität zu Renin. Es hat sich gezeigt, dass es eine hohe Selektivität und Wirksamkeit bei der Hemmung der Reninaktivität aufweist, was es zu einem wertvollen Werkzeug für die Forschung und potenzielle therapeutische Anwendungen macht .
Eigenschaften
CAS-Nummer |
126722-78-9 |
---|---|
Molekularformel |
C47H72N10O13 |
Molekulargewicht |
985.1 g/mol |
IUPAC-Name |
4-amino-N-[(2S)-1-[[3-[[(4S)-4-amino-5-cyclohexyl-3-hydroxypentanoyl]-[(2S,3S)-2-[(4-amino-2-methylpyrimidin-5-yl)methylamino]-3-methylpentanoyl]amino]-3-oxopropyl]amino]-1-oxo-3-phenylpropan-2-yl]piperidine-1-carboxamide;2-hydroxypropane-1,2,3-tricarboxylic acid |
InChI |
InChI=1S/C41H64N10O6.C6H8O7/c1-4-26(2)37(47-25-30-24-46-27(3)48-38(30)44)40(56)51(36(54)23-34(52)32(43)21-28-11-7-5-8-12-28)35(53)15-18-45-39(55)33(22-29-13-9-6-10-14-29)49-41(57)50-19-16-31(42)17-20-50;7-3(8)1-6(13,5(11)12)2-4(9)10/h6,9-10,13-14,24,26,28,31-34,37,47,52H,4-5,7-8,11-12,15-23,25,42-43H2,1-3H3,(H,45,55)(H,49,57)(H2,44,46,48);13H,1-2H2,(H,7,8)(H,9,10)(H,11,12)/t26-,32-,33-,34?,37-;/m0./s1 |
InChI-Schlüssel |
CJGVCWKXZSDVRK-DFQVXBJQSA-N |
Isomerische SMILES |
CC[C@H](C)[C@@H](C(=O)N(C(=O)CCNC(=O)[C@H](CC1=CC=CC=C1)NC(=O)N2CCC(CC2)N)C(=O)CC([C@H](CC3CCCCC3)N)O)NCC4=CN=C(N=C4N)C.C(C(=O)O)C(CC(=O)O)(C(=O)O)O |
SMILES |
CCC(C)C(C(=O)N(C(=O)CCNC(=O)C(CC1=CC=CC=C1)NC(=O)N2CCC(CC2)N)C(=O)CC(C(CC3CCCCC3)N)O)NCC4=CN=C(N=C4N)C.C(C(=O)O)C(CC(=O)O)(C(=O)O)O |
Kanonische SMILES |
CCC(C)C(C(=O)N(C(=O)CCNC(=O)C(CC1=CC=CC=C1)NC(=O)N2CCC(CC2)N)C(=O)CC(C(CC3CCCCC3)N)O)NCC4=CN=C(N=C4N)C.C(C(=O)O)C(CC(=O)O)(C(=O)O)O |
Synonyme |
5-(4-aminopiperidyl-1-carbonyl)-L-2,6-Phe-beta-Ala-(4S-amino-3S-hydroxy-5-cyclohexyl)-pentancarbonyl-L-Ile-aminomethyl-4-amino-2-methylpyrimidine citrate 5-(4-aminopiperidyl-1-carbonyl)-L-2,6-phenylalanyl-beta-alanyl-(4S-amino-3S-hydroxy-5-cyclohexyl)-pentancarbonyl-L-isoleucyl-aminomethyl-4-amino-2-methylpyrimidine citrate EMD 56133 EMD-56133 |
Herkunft des Produkts |
United States |
Haftungsausschluss und Informationen zu In-Vitro-Forschungsprodukten
Bitte beachten Sie, dass alle Artikel und Produktinformationen, die auf BenchChem präsentiert werden, ausschließlich zu Informationszwecken bestimmt sind. Die auf BenchChem zum Kauf angebotenen Produkte sind speziell für In-vitro-Studien konzipiert, die außerhalb lebender Organismen durchgeführt werden. In-vitro-Studien, abgeleitet von dem lateinischen Begriff "in Glas", beinhalten Experimente, die in kontrollierten Laborumgebungen unter Verwendung von Zellen oder Geweben durchgeführt werden. Es ist wichtig zu beachten, dass diese Produkte nicht als Arzneimittel oder Medikamente eingestuft sind und keine Zulassung der FDA für die Vorbeugung, Behandlung oder Heilung von medizinischen Zuständen, Beschwerden oder Krankheiten erhalten haben. Wir müssen betonen, dass jede Form der körperlichen Einführung dieser Produkte in Menschen oder Tiere gesetzlich strikt untersagt ist. Es ist unerlässlich, sich an diese Richtlinien zu halten, um die Einhaltung rechtlicher und ethischer Standards in Forschung und Experiment zu gewährleisten.