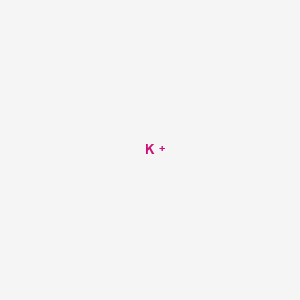
Potassium ion
Übersicht
Beschreibung
Potassium ion (K⁺) is a monoatomic cation essential for cellular function in all living organisms. Biologically, K⁺ is highly concentrated in plant cells and fruits, making it a vital nutrient in human diets . SHE) and abundance in the Earth’s crust (1.5 wt%) . Its ionic radius (1.38 Å) and charge density influence its interaction with biological ion channels (e.g., hERG) and synthetic ion-exchange materials .
Vorbereitungsmethoden
Synthetic Routes::
Electrolysis of Potassium Chloride (KCl): In the laboratory, potassium metal can be synthesized by electrolyzing molten potassium chloride (KCl). The reduction of K⁺ ions at the cathode produces potassium metal.
Thermal Reduction of Potassium Hydroxide (KOH): Industrially, potassium is produced by heating potassium hydroxide (KOH) with a reducing agent like carbon. The reaction yields potassium metal and water vapor.
Analyse Chemischer Reaktionen
Reaktionstypen::
Oxidation: Kalium gibt leicht ein Elektron ab, um K⁺ zu bilden, was es zu einem starken Reduktionsmittel macht.
Substitution: Kaliumionen können andere Kationen in verschiedenen Verbindungen ersetzen.
Komplexierung: K⁺ bildet in der Koordinationschemie Komplexe mit Liganden.
Kaliumhydroxid (KOH): Wird aufgrund seiner basischen Natur in verschiedenen Reaktionen eingesetzt.
Kaliumpermanganat (KMnO₄): Ein Oxidationsmittel.
Kaliumcarbonat (K₂CO₃): Wird in der Glasproduktion und als Puffer verwendet.
Kaliumhydroxid (KOH): Entsteht bei der Reaktion von Kalium mit Wasser.
Kaliumchlorid (KCl): Wird üblicherweise durch die Reaktion von K⁺ mit Salzsäure erhalten.
Wissenschaftliche Forschungsanwendungen
Biological Applications
Role in Cellular Functions
Potassium ions are vital for maintaining cellular homeostasis and are involved in numerous physiological processes, including:
- Nerve Transmission : K is essential for the generation and propagation of action potentials in neurons.
- Muscle Contraction : It plays a critical role in muscle contraction mechanisms.
- Fluid Balance : K helps regulate osmotic pressure and fluid balance within cells.
Genetically-Encoded Potassium Ion Biosensors
Recent advancements have led to the development of genetically-encoded fluorescent biosensors for potassium ions, such as GINKO2. These biosensors allow for real-time imaging and monitoring of K dynamics in various model organisms, including plants and animals. They provide insights into K dependent processes under both normal and pathological conditions, enhancing our understanding of cellular functions and disorders .
Agricultural Applications
Fertilizers
Potassium ions are a key component of fertilizers used in agriculture. They are crucial for plant growth, influencing:
- Photosynthesis : Enhances the photosynthetic process.
- Nutrient Transport : Aids in the transport of nutrients within plants.
- Disease Resistance : Improves plants' resistance to diseases.
The primary forms of potassium fertilizers include potassium chloride (KCl), potassium sulfate (KSO), and potassium nitrate (KNO). Approximately 95% of global potassium production is utilized in fertilizers, making it indispensable for modern agriculture .
Energy Storage Applications
Potassium-Ion Batteries
Potassium-ion batteries (KIBs) have emerged as a promising alternative to lithium-ion batteries due to the abundance of potassium and its favorable electrochemical properties. Key features include:
- Cost-Effectiveness : KIBs can be cheaper to produce than lithium-ion batteries.
- High Energy Density : Recent developments have achieved energy densities comparable to lithium-ion systems, with reports indicating gravimetric energy densities between 160-180 Wh/kg .
- Environmental Benefits : Utilizing potassium can help reduce reliance on scarce lithium resources.
Recent innovations involve using materials like Prussian White as cathodes, which enhance conductivity and overall battery performance .
Industrial Applications
Chemical Manufacturing
Potassium ions are integral to various chemical processes:
- Potassium Hydroxide (KOH) : Used for pH control, saponification, and as a strong base in industrial applications.
- Potassium Carbonate (KCO) : Employed in glass manufacturing, soap production, and as a drying agent.
- Potassium Permanganate (KMnO) : Utilized as an oxidizing agent in organic synthesis and water treatment.
These compounds demonstrate the versatility of potassium ions across multiple industrial sectors .
Case Studies
Wirkmechanismus
Cell Membrane Potential: K⁺ plays a crucial role in establishing the resting membrane potential in cells.
Ion Channels: Voltage-gated potassium channels regulate ion flow across cell membranes.
Vergleich Mit ähnlichen Verbindungen
Comparison with Similar Compounds and Ions
Sodium (Na⁺)
SHE) . These differences lead to distinct biological and chemical behaviors:
- Biological Selectivity: Potassium channels selectively permit K⁺ over Na⁺ due to precise pore sizing and hydration energy barriers.
- Bond Energies : K⁺ forms weaker bonds with nucleic acid bases (e.g., adenine, guanine) compared to Na⁺. Theoretical bond energies for K⁺-DNA complexes are ~50–70 kJ/mol, while Na⁺ complexes range ~70–90 kJ/mol .
Lithium (Li⁺)
Lithium ion (Li⁺) is smaller (0.76 Å) and has a higher charge density than K⁺, making it less suitable for ion-exchange processes requiring large-ion accommodation. For example:
- Ion Exchange : Titanium-based materials (e.g., Li₂TiO₃) exhibit Li⁺ exchange capacities of 0.2–8.0 mmol/g, while protonated potassium polytitanates (K₂Ti₄O₉) achieve comparable Li⁺ extraction efficiency (up to 8.0 mmol/g) but with lower synthesis costs .
Thallium (Tl⁺)
Thallium ion (Tl⁺) mimics K⁺ in ionic radius (1.49 Å) but is highly toxic. It crosses biological barriers (e.g., blood-brain, placental) and disrupts K⁺-dependent enzymes by binding to sulfhydryl and amino groups . This interference causes severe neurological and metabolic dysfunction.
Ammonium (NH₄⁺)
The ammonium ion (NH₄⁺) closely matches K⁺ in size (1.48 Å), leading to structural similarities in ionic compounds. For instance:
- Solubility: NH₄⁺ salts (e.g., NH₄NO₃) often mirror the solubility profiles of K⁺ salts (e.g., KNO₃) .
- Biological Systems : NH₄⁺ can substitute K⁺ in some transporters but is excluded in others due to charge differences (NH₄⁺: +1 vs. K⁺: +1) and hydrogen-bonding capabilities .
Titanium-Based Ion Exchangers
Potassium polytitanates (K₂O·nTiO₂) and protonated derivatives are compared with lithium titanates (Li₂TiO₃) for Li⁺ extraction:
- Capacity : Both achieve 0.2–8.0 mmol/g Li⁺ exchange capacity, but potassium polytitanates offer superior regeneration stability in acidic conditions .
- Cost : K⁺-based exchangers reduce precursor costs by avoiding lithium-containing raw materials .
Hydroxides: NaOH vs. KOH
Key Research Findings and Data Tables
Table 1: Ionic and Electrochemical Properties
Ion | Ionic Radius (Å) | Electrode Potential (V vs. SHE) | Bond Energy with DNA (kJ/mol) |
---|---|---|---|
K⁺ | 1.38 | -2.93 | 50–70 |
Na⁺ | 0.95 | -2.71 | 70–90 |
Li⁺ | 0.76 | -3.04 | 90–110 |
Tl⁺ | 1.49 | -0.34 | N/A |
Table 2: Ion Exchange Performance
Material | Target Ion | Capacity (mmol/g) | Stability After Regeneration |
---|---|---|---|
K₂Ti₄O₉ (Protonated) | Li⁺ | 8.0 | High |
Li₂TiO₃ | Li⁺ | 8.0 | Moderate |
Table 3: Battery Performance of K⁺ vs. Na⁺
Metric | K⁺-Ion Battery (SNC-650) | Na⁺-Ion Battery |
---|---|---|
Capacity (0.1 A/g) | 311.2 mAh/g | ~300 mAh/g |
Cycle Stability (1 A/g) | 96% after 100 cycles | ~80% after 100 cycles |
Biologische Aktivität
Potassium ions (K) are essential for various biological processes across different organisms, including bacteria, plants, and animals. Their role extends from maintaining cellular homeostasis to facilitating critical physiological functions such as nerve transmission and muscle contraction. This article explores the biological activity of potassium ions, supported by data tables, case studies, and detailed research findings.
Importance of Potassium Ions in Biological Systems
Potassium is the primary intracellular cation in all living cells, playing a crucial role in maintaining fluid and electrolyte balance. The concentration gradient of potassium across cell membranes is vital for generating membrane potentials necessary for electrical signaling in neurons and muscle cells.
- Concentration Levels :
-
Membrane Potential Generation :
Potassium ions contribute significantly to the resting membrane potential of cells. The differential distribution of potassium and sodium ions across the cell membrane creates an electrochemical gradient that is fundamental for action potentials in neurons and muscle fibers . -
Transport Mechanisms :
Potassium transport is mediated by various channels and transporters, including:
Physiological Roles
- Nerve Transmission : K is crucial for repolarizing the neuronal membrane after depolarization during action potentials.
- Muscle Contraction : In cardiac and skeletal muscles, K helps regulate contraction by affecting action potential duration and excitability.
- Fluid Balance : It plays a significant role in controlling osmotic balance in cells, influencing blood pressure regulation and kidney function .
Case Study 1: Potassium Homeostasis in Bacteria
Research indicates that potassium homeostasis is vital for bacterial survival. For instance, potassium channels in bacteria help maintain osmotic balance under stress conditions such as high salinity or nutrient limitation. A study demonstrated that mutations affecting potassium transport led to increased susceptibility to osmotic stress, highlighting the importance of K in microbial physiology .
Case Study 2: Potassium Deficiency Effects
A deficiency in potassium can lead to severe physiological consequences. Clinical studies have shown that hypokalemia (low serum potassium levels) can result in muscle weakness, cardiac arrhythmias, and increased blood pressure due to impaired vascular smooth muscle function. A notable study found that patients with chronic kidney disease often exhibit hypokalemia due to impaired renal handling of potassium .
Data Table: Effects of Potassium on Physiological Functions
Function | Description | Consequence of Deficiency |
---|---|---|
Nerve Transmission | Maintains resting membrane potential | Neurological dysfunction |
Muscle Contraction | Regulates excitability and contraction strength | Muscle weakness |
Fluid Balance | Controls osmotic pressure within cells | Hypertension |
Acid-Base Balance | Influences bicarbonate reabsorption in kidneys | Metabolic alkalosis |
Q & A
Basic Research Questions
Q. How can potassium ion concentrations be accurately measured in complex biological or environmental samples?
- Methodological Answer : Use ion-selective electrodes (ISEs) or flame photometry for direct quantification in biological fluids (e.g., blood, urine). For environmental samples (e.g., soil), employ ion meters after extraction via standardized protocols like the 2:1 water-soil ratio method. Validation through atomic absorption spectroscopy (AAS) or inductively coupled plasma mass spectrometry (ICP-MS) ensures precision .
Q. What statistical approaches are effective in analyzing the impact of K⁺ on microbial fermentation processes?
- Methodological Answer : Implement Box-Wilson experimental designs to model interactions between K⁺, Na⁺, and Zn²⁺ in ethanol production. Use response surface methodology (RSM) to identify optimal ion concentrations and validate predictions via ANOVA. For example, a tripartite ion model achieved 4.19% ethanol yield at 7900 mg/L K⁺, highlighting K⁺'s dominant role over interactive effects .
Q. How do researchers standardize K⁺ measurements across laboratories to minimize pre-analytical variability?
- Methodological Answer : Control transport time and temperature during sample handling, as plasma K⁺ levels can artificially rise due to hemolysis or delayed processing. Protocols should include immediate centrifugation, refrigeration, and standardized calibration of ion meters .
Advanced Research Questions
Q. What experimental designs resolve mechanistic contradictions in potassium channel gating dynamics?
- Methodological Answer : Combine ultrafast spectroscopy with molecular dynamics (MD) simulations to capture real-time ion translocation. For example, semisynthetic channel proteins enable direct observation of voltage-dependent conformational changes, distinguishing between "knock-on" and "direct coupling" transport models . Phase-voltage space filling protocols (e.g., using hERG channel models) optimize clamp protocols to map gating transitions .
Q. How can model discrepancy be quantified in predictive simulations of K⁺ channel behavior?
- Methodological Answer : Train ensemble models using diverse electrophysiological protocols (e.g., step-voltage clamps) to expose parameter conflicts. Bayesian uncertainty quantification identifies discrepancies arising from oversimplified conductance models. For instance, conflicting activation thresholds in IKr models highlight misspecification in inactivation kinetics .
Q. What synthesis strategies improve structural stability of K⁺ battery anode materials like MoSe₂?
- Methodological Answer : Integrate organic small-molecule intercalation (e.g., urea) to weaken interlayer forces, followed by carbon-skeleton confinement to prevent pulverization. Electrochemical testing shows ultra-dispersed MoSe₂@carbon achieves 580 mAh/g capacity over 500 cycles, validated via TEM and cyclic voltammetry .
Q. How to address contradictory findings in K⁺ migration pathways within mineral frameworks?
- Methodological Answer : Apply bond-valence site energy (BVSE) modeling to predict 1D vs. 3D diffusion barriers. Compare with tracer experiments in litidionite-group minerals, where Na⁺ migrates preferentially (barrier: 0.8 eV) over K⁺ (barrier: 2.1 eV), resolving discrepancies between theoretical and empirical data .
Q. Data Contradiction & Validation
Q. How should researchers analyze conflicting data on K⁺'s role in neuronal excitability?
- Methodological Answer : Reconcile contradictions by testing hypotheses across multiple scales:
- Cellular : Patch-clamp electrophysiology to isolate K⁺ currents (e.g., Kv channels) in neurons.
- Molecular : Fluorescence imaging (e.g., K⁺-sensitive dyes) to map intracellular flux.
- Theoretical : Compare Hodgkin-Huxley models with all-atom MD simulations of ion hydration effects. Discrepancies often arise from neglecting glial buffering or non-canonical channel isoforms .
Q. What criteria validate K⁺'s biomarker potential in male infertility studies?
- Methodological Answer : Use case-control designs to correlate sperm K⁺ conductance (via CatSper channel activity) with ART outcomes. Confounders like pH or Ca²⁺ crosstalk require multivariate regression. Recent studies link dysfunctional K⁺ flux to failed fertilization, but causal validation needs CRISPR-edited models .
Q. Methodological Innovation
Q. How to optimize K⁺-specific fluorescent probes for dynamic imaging in live cells?
- Methodological Answer : Design genetically encoded sensors (e.g., GEPIIs) with high K⁺/Na⁺ selectivity ratios (>100:1). Validate via two-photon microscopy in brain slices, comparing FRET responses under varying ion concentrations. Calibrate using patch-clamp simultaneous recordings .
Q. What computational frameworks integrate multi-omics data to study K⁺ homeostasis in plants?
- Methodological Answer : Couple transcriptomic networks (e.g., K⁺-responsive genes like HAK5) with ionomics datasets using machine learning. For example, random forest models trained on Arabidopsis root data predict K⁺ uptake efficiency under salinity stress (R² > 0.85) .
Eigenschaften
IUPAC Name |
potassium(1+) | |
---|---|---|
Source | PubChem | |
URL | https://pubchem.ncbi.nlm.nih.gov | |
Description | Data deposited in or computed by PubChem | |
InChI |
InChI=1S/K/q+1 | |
Source | PubChem | |
URL | https://pubchem.ncbi.nlm.nih.gov | |
Description | Data deposited in or computed by PubChem | |
InChI Key |
NPYPAHLBTDXSSS-UHFFFAOYSA-N | |
Source | PubChem | |
URL | https://pubchem.ncbi.nlm.nih.gov | |
Description | Data deposited in or computed by PubChem | |
Canonical SMILES |
[K+] | |
Source | PubChem | |
URL | https://pubchem.ncbi.nlm.nih.gov | |
Description | Data deposited in or computed by PubChem | |
Molecular Formula |
K+ | |
Source | PubChem | |
URL | https://pubchem.ncbi.nlm.nih.gov | |
Description | Data deposited in or computed by PubChem | |
DSSTOX Substance ID |
DTXSID9042671 | |
Record name | Potassium cation | |
Source | EPA DSSTox | |
URL | https://comptox.epa.gov/dashboard/DTXSID9042671 | |
Description | DSSTox provides a high quality public chemistry resource for supporting improved predictive toxicology. | |
Molecular Weight |
39.0983 g/mol | |
Source | PubChem | |
URL | https://pubchem.ncbi.nlm.nih.gov | |
Description | Data deposited in or computed by PubChem | |
Physical Description |
Solid | |
Record name | Potassium | |
Source | Human Metabolome Database (HMDB) | |
URL | http://www.hmdb.ca/metabolites/HMDB0000586 | |
Description | The Human Metabolome Database (HMDB) is a freely available electronic database containing detailed information about small molecule metabolites found in the human body. | |
Explanation | HMDB is offered to the public as a freely available resource. Use and re-distribution of the data, in whole or in part, for commercial purposes requires explicit permission of the authors and explicit acknowledgment of the source material (HMDB) and the original publication (see the HMDB citing page). We ask that users who download significant portions of the database cite the HMDB paper in any resulting publications. | |
CAS No. |
24203-36-9, 7440-09-7 | |
Record name | Potassium(1+) | |
Source | CAS Common Chemistry | |
URL | https://commonchemistry.cas.org/detail?cas_rn=24203-36-9 | |
Description | CAS Common Chemistry is an open community resource for accessing chemical information. Nearly 500,000 chemical substances from CAS REGISTRY cover areas of community interest, including common and frequently regulated chemicals, and those relevant to high school and undergraduate chemistry classes. This chemical information, curated by our expert scientists, is provided in alignment with our mission as a division of the American Chemical Society. | |
Explanation | The data from CAS Common Chemistry is provided under a CC-BY-NC 4.0 license, unless otherwise stated. | |
Record name | Potassium cation | |
Source | ChemIDplus | |
URL | https://pubchem.ncbi.nlm.nih.gov/substance/?source=chemidplus&sourceid=0024203369 | |
Description | ChemIDplus is a free, web search system that provides access to the structure and nomenclature authority files used for the identification of chemical substances cited in National Library of Medicine (NLM) databases, including the TOXNET system. | |
Record name | Potassium cation | |
Source | DrugBank | |
URL | https://www.drugbank.ca/drugs/DB01345 | |
Description | The DrugBank database is a unique bioinformatics and cheminformatics resource that combines detailed drug (i.e. chemical, pharmacological and pharmaceutical) data with comprehensive drug target (i.e. sequence, structure, and pathway) information. | |
Explanation | Creative Common's Attribution-NonCommercial 4.0 International License (http://creativecommons.org/licenses/by-nc/4.0/legalcode) | |
Record name | Potassium cation | |
Source | EPA DSSTox | |
URL | https://comptox.epa.gov/dashboard/DTXSID9042671 | |
Description | DSSTox provides a high quality public chemistry resource for supporting improved predictive toxicology. | |
Record name | POTASSIUM CATION | |
Source | FDA Global Substance Registration System (GSRS) | |
URL | https://gsrs.ncats.nih.gov/ginas/app/beta/substances/295O53K152 | |
Description | The FDA Global Substance Registration System (GSRS) enables the efficient and accurate exchange of information on what substances are in regulated products. Instead of relying on names, which vary across regulatory domains, countries, and regions, the GSRS knowledge base makes it possible for substances to be defined by standardized, scientific descriptions. | |
Explanation | Unless otherwise noted, the contents of the FDA website (www.fda.gov), both text and graphics, are not copyrighted. They are in the public domain and may be republished, reprinted and otherwise used freely by anyone without the need to obtain permission from FDA. Credit to the U.S. Food and Drug Administration as the source is appreciated but not required. | |
Record name | Potassium | |
Source | Human Metabolome Database (HMDB) | |
URL | http://www.hmdb.ca/metabolites/HMDB0000586 | |
Description | The Human Metabolome Database (HMDB) is a freely available electronic database containing detailed information about small molecule metabolites found in the human body. | |
Explanation | HMDB is offered to the public as a freely available resource. Use and re-distribution of the data, in whole or in part, for commercial purposes requires explicit permission of the authors and explicit acknowledgment of the source material (HMDB) and the original publication (see the HMDB citing page). We ask that users who download significant portions of the database cite the HMDB paper in any resulting publications. | |
Melting Point |
63.2 °C | |
Record name | Potassium cation | |
Source | DrugBank | |
URL | https://www.drugbank.ca/drugs/DB01345 | |
Description | The DrugBank database is a unique bioinformatics and cheminformatics resource that combines detailed drug (i.e. chemical, pharmacological and pharmaceutical) data with comprehensive drug target (i.e. sequence, structure, and pathway) information. | |
Explanation | Creative Common's Attribution-NonCommercial 4.0 International License (http://creativecommons.org/licenses/by-nc/4.0/legalcode) | |
Record name | Potassium | |
Source | Human Metabolome Database (HMDB) | |
URL | http://www.hmdb.ca/metabolites/HMDB0000586 | |
Description | The Human Metabolome Database (HMDB) is a freely available electronic database containing detailed information about small molecule metabolites found in the human body. | |
Explanation | HMDB is offered to the public as a freely available resource. Use and re-distribution of the data, in whole or in part, for commercial purposes requires explicit permission of the authors and explicit acknowledgment of the source material (HMDB) and the original publication (see the HMDB citing page). We ask that users who download significant portions of the database cite the HMDB paper in any resulting publications. | |
Synthesis routes and methods I
Procedure details
Synthesis routes and methods II
Procedure details
Synthesis routes and methods III
Procedure details
Synthesis routes and methods IV
Procedure details
Synthesis routes and methods V
Procedure details
Haftungsausschluss und Informationen zu In-Vitro-Forschungsprodukten
Bitte beachten Sie, dass alle Artikel und Produktinformationen, die auf BenchChem präsentiert werden, ausschließlich zu Informationszwecken bestimmt sind. Die auf BenchChem zum Kauf angebotenen Produkte sind speziell für In-vitro-Studien konzipiert, die außerhalb lebender Organismen durchgeführt werden. In-vitro-Studien, abgeleitet von dem lateinischen Begriff "in Glas", beinhalten Experimente, die in kontrollierten Laborumgebungen unter Verwendung von Zellen oder Geweben durchgeführt werden. Es ist wichtig zu beachten, dass diese Produkte nicht als Arzneimittel oder Medikamente eingestuft sind und keine Zulassung der FDA für die Vorbeugung, Behandlung oder Heilung von medizinischen Zuständen, Beschwerden oder Krankheiten erhalten haben. Wir müssen betonen, dass jede Form der körperlichen Einführung dieser Produkte in Menschen oder Tiere gesetzlich strikt untersagt ist. Es ist unerlässlich, sich an diese Richtlinien zu halten, um die Einhaltung rechtlicher und ethischer Standards in Forschung und Experiment zu gewährleisten.