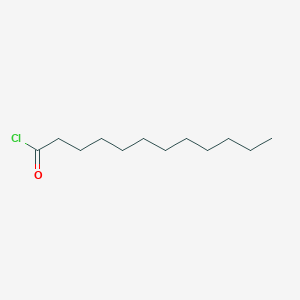
Lauroylchlorid
Übersicht
Beschreibung
Es ist das Säurechlorid-Derivat der Laurinsäure und wird üblicherweise als Reagenz zur Einführung der Lauroylgruppe in verschiedenen chemischen Reaktionen verwendet . Diese Verbindung ist eine farblose Flüssigkeit mit stechendem Geruch und wird hauptsächlich zur Herstellung von Dilauroylperoxid verwendet, das in freiradikalischen Polymerisationen weit verbreitet ist .
Wissenschaftliche Forschungsanwendungen
Lauroylchlorid hat eine breite Palette von Anwendungen in der wissenschaftlichen Forschung:
Chemie: Es wird als maßgeschneidertes Mittel zur chemischen Modifizierung von Nanocellulosen, nanofibrillierter Cellulose und Cellulose-Nanokristallen verwendet.
Medizin: Es wird bei der Synthese verschiedener pharmazeutischer Zwischenprodukte und Wirkstoffe eingesetzt.
5. Wirkmechanismus
This compound wirkt als reaktives Zwischenprodukt in chemischen Reaktionen. Es unterliegt nucleophilen Substitutionsreaktionen mit verschiedenen Nucleophilen, wie Aminen und Alkoholen, unter Bildung von Amiden bzw. Estern . Diese Reaktivität ermöglicht die Verwendung in der Synthese einer breiten Palette von Verbindungen. Die molekularen Ziele und Wege, die an diesen Reaktionen beteiligt sind, sind in erster Linie die nucleophilen Stellen an den reagierenden Molekülen.
Wirkmechanismus
Lauroyl chloride, also known as dodecanoyl chloride, is an organic compound with the formula CH3(CH2)10COCl . It is the acid chloride of lauric acid .
Target of Action
Lauroyl chloride is a standard reagent for installing the lauroyl group . It is mainly used as a precursor to dilauroyl peroxide, which is widely used in free-radical polymerizations . It is also used as a tailoring agent for chemical modification of nanocelluloses of different length, nanofibrillated cellulose, and cellulose nanocrystals .
Mode of Action
Lauroyl chloride is a substrate for diverse reactions characteristic of acid chlorides . With base, it converts to laurone, a ketone with the formula [CH3(CH2)10]2CO . With sodium azide, it reacts to give undecyl isocyanate via a Curtius rearrangement of the acyl azide .
Biochemical Pathways
It is known that lauroyl chloride can react with bases to form laurone , and with sodium azide to form undecyl isocyanate .
Result of Action
It is known that lauroyl chloride can be used to modify nanocelluloses and collagen, improving their water solubility and surface activity .
Action Environment
Lauroyl chloride may be incompatible with strong oxidizing and reducing agents. It may also be incompatible with many amines, nitrides, azo/diazo compounds, alkali metals, and epoxides . Environmental factors such as temperature, pH, and the presence of other chemicals can influence the action, efficacy, and stability of lauroyl chloride.
Safety and Hazards
Lauroyl chloride is toxic; inhalation, ingestion, or skin contact with the material may cause severe injury or death . Contact with molten substance may cause severe burns to skin and eyes . It is also considered hazardous by the 2012 OSHA Hazard Communication Standard (29 CFR 1910.1200) due to its potential to cause skin corrosion/irritation, serious eye damage/eye irritation, skin sensitization, and specific target organ toxicity (single exposure) with the target organs being the respiratory system .
Zukünftige Richtungen
Biochemische Analyse
Biochemical Properties
Lauroyl chloride plays a significant role in biochemical reactions, primarily as a reagent for acylation. It interacts with various enzymes, proteins, and other biomolecules through the formation of covalent bonds. One notable interaction is with hydroxyl and amino groups in proteins and enzymes, leading to the formation of esters and amides . This acylation process can modify the activity and function of these biomolecules, influencing biochemical pathways and cellular processes .
Cellular Effects
Lauroyl chloride affects various types of cells and cellular processes. It can influence cell function by modifying cell signaling pathways, gene expression, and cellular metabolism. For instance, the acylation of proteins by lauroyl chloride can alter their conformation and activity, impacting signal transduction pathways . Additionally, lauroyl chloride can affect gene expression by modifying transcription factors and other regulatory proteins, leading to changes in cellular metabolism and function .
Molecular Mechanism
The molecular mechanism of lauroyl chloride involves its reactivity with nucleophilic groups in biomolecules. It forms covalent bonds with hydroxyl, amino, and thiol groups, leading to the acylation of proteins and enzymes . This acylation can result in enzyme inhibition or activation, depending on the specific biomolecule and the site of modification . Additionally, lauroyl chloride can induce changes in gene expression by modifying transcription factors and other regulatory proteins .
Temporal Effects in Laboratory Settings
In laboratory settings, the effects of lauroyl chloride can change over time due to its stability and degradation. Lauroyl chloride is relatively stable under dry conditions but can hydrolyze in the presence of moisture, leading to the formation of lauric acid and hydrochloric acid . This hydrolysis can affect its long-term impact on cellular function, as the degradation products may have different biological activities . In vitro and in vivo studies have shown that prolonged exposure to lauroyl chloride can lead to cumulative effects on cellular processes and function .
Dosage Effects in Animal Models
The effects of lauroyl chloride vary with different dosages in animal models. At low doses, lauroyl chloride can acylate specific proteins and enzymes, leading to subtle changes in cellular function . At high doses, it can cause toxic effects, including cell membrane disruption, enzyme inhibition, and oxidative stress . These adverse effects are dose-dependent and can lead to significant physiological changes and toxicity in animal models .
Metabolic Pathways
Lauroyl chloride is involved in various metabolic pathways, primarily through its role as an acylating agent. It interacts with enzymes and cofactors involved in lipid metabolism, leading to the formation of acylated intermediates . These intermediates can affect metabolic flux and alter the levels of metabolites in cells . Additionally, lauroyl chloride can influence the synthesis and degradation of fatty acids and other lipids, impacting overall cellular metabolism .
Transport and Distribution
Within cells and tissues, lauroyl chloride is transported and distributed through passive diffusion and interactions with transport proteins . It can bind to specific transporters and binding proteins, facilitating its movement across cellular membranes and within different cellular compartments . The localization and accumulation of lauroyl chloride can affect its biological activity and impact cellular processes .
Subcellular Localization
Lauroyl chloride is localized in various subcellular compartments, including the cytoplasm, endoplasmic reticulum, and mitochondria . Its activity and function can be influenced by its subcellular localization, as different compartments provide distinct environments for biochemical reactions . Targeting signals and post-translational modifications can direct lauroyl chloride to specific organelles, affecting its role in cellular processes .
Vorbereitungsmethoden
Lauroylchlorid wird durch Reaktion von Laurinsäure mit Thionylchlorid synthetisiert. Die Reaktion beinhaltet typischerweise das Erhitzen von Laurinsäure mit Thionylchlorid in Gegenwart eines Katalysators wie Imidazol oder 2-Methylimidazol . Die Reaktionsbedingungen umfassen die Aufrechterhaltung der Temperatur bei etwa 90 °C und die Gewährleistung eines sanften Rückflusses von Phosgen. Die Reaktion wird fortgesetzt, bis die Entwicklung von Chlorwasserstoff aufhört . Industrielle Produktionsverfahren folgen ähnlichen Verfahren, jedoch in größerem Maßstab, um eine effiziente Umwandlung und hohe Ausbeute zu gewährleisten.
Analyse Chemischer Reaktionen
Lauroylchlorid unterliegt verschiedenen Arten chemischer Reaktionen, die für Säurechloride charakteristisch sind:
Nucleophile Substitution: This compound reagiert mit Nucleophilen wie Aminen und Alkoholen unter Bildung von Amiden bzw. Estern.
Reduktion: Es kann unter Verwendung von Basen zu Laurone, einem Keton, reduziert werden.
Curtius-Umlagerung: Bei Reaktion mit Natriumazid bildet this compound über eine Curtius-Umlagerung des Acylazias Undecylisocyanat.
Häufige Reagenzien, die in diesen Reaktionen verwendet werden, sind Natriumazid, Basen und verschiedene Nucleophile. Die Hauptprodukte, die aus diesen Reaktionen entstehen, sind Amide, Ester, Ketone und Isocyanate.
Vergleich Mit ähnlichen Verbindungen
Lauroylchlorid ähnelt anderen Acylchloriden wie Acetylchlorid, Benzoylchlorid und Stearoylchlorid. Seine Besonderheit liegt in seiner längeren Kohlenstoffkette, die ihm andere physikalische und chemische Eigenschaften verleiht. So hat this compound im Vergleich zu Acetylchlorid und Benzoylchlorid einen höheren Siedepunkt und andere Löslichkeitseigenschaften . Ähnliche Verbindungen umfassen:
- Acetylchlorid (CH₃COCl)
- Benzoylchlorid (C₆H₅COCl)
- Stearoylchlorid (C₁₇H₃₅COCl)
Diese Verbindungen weisen ähnliche Reaktivitätsmuster auf, unterscheiden sich jedoch in ihren Kohlenstoffkettenlängen und spezifischen Anwendungen.
Eigenschaften
IUPAC Name |
dodecanoyl chloride | |
---|---|---|
Source | PubChem | |
URL | https://pubchem.ncbi.nlm.nih.gov | |
Description | Data deposited in or computed by PubChem | |
InChI |
InChI=1S/C12H23ClO/c1-2-3-4-5-6-7-8-9-10-11-12(13)14/h2-11H2,1H3 | |
Source | PubChem | |
URL | https://pubchem.ncbi.nlm.nih.gov | |
Description | Data deposited in or computed by PubChem | |
InChI Key |
NQGIJDNPUZEBRU-UHFFFAOYSA-N | |
Source | PubChem | |
URL | https://pubchem.ncbi.nlm.nih.gov | |
Description | Data deposited in or computed by PubChem | |
Canonical SMILES |
CCCCCCCCCCCC(=O)Cl | |
Source | PubChem | |
URL | https://pubchem.ncbi.nlm.nih.gov | |
Description | Data deposited in or computed by PubChem | |
Molecular Formula |
C12H23ClO | |
Source | PubChem | |
URL | https://pubchem.ncbi.nlm.nih.gov | |
Description | Data deposited in or computed by PubChem | |
DSSTOX Substance ID |
DTXSID5044368 | |
Record name | Dodecanoyl chloride | |
Source | EPA DSSTox | |
URL | https://comptox.epa.gov/dashboard/DTXSID5044368 | |
Description | DSSTox provides a high quality public chemistry resource for supporting improved predictive toxicology. | |
Molecular Weight |
218.76 g/mol | |
Source | PubChem | |
URL | https://pubchem.ncbi.nlm.nih.gov | |
Description | Data deposited in or computed by PubChem | |
Physical Description |
Liquid, Water white liquid; [HSDB] Colorless liquid; [MSDSonline] | |
Record name | Dodecanoyl chloride | |
Source | EPA Chemicals under the TSCA | |
URL | https://www.epa.gov/chemicals-under-tsca | |
Description | EPA Chemicals under the Toxic Substances Control Act (TSCA) collection contains information on chemicals and their regulations under TSCA, including non-confidential content from the TSCA Chemical Substance Inventory and Chemical Data Reporting. | |
Record name | Dodecanoyl chloride | |
Source | Haz-Map, Information on Hazardous Chemicals and Occupational Diseases | |
URL | https://haz-map.com/Agents/5048 | |
Description | Haz-Map® is an occupational health database designed for health and safety professionals and for consumers seeking information about the adverse effects of workplace exposures to chemical and biological agents. | |
Explanation | Copyright (c) 2022 Haz-Map(R). All rights reserved. Unless otherwise indicated, all materials from Haz-Map are copyrighted by Haz-Map(R). No part of these materials, either text or image may be used for any purpose other than for personal use. Therefore, reproduction, modification, storage in a retrieval system or retransmission, in any form or by any means, electronic, mechanical or otherwise, for reasons other than personal use, is strictly prohibited without prior written permission. | |
Boiling Point |
145 °C @ 18 MM HG | |
Record name | DODECANOYL CHLORIDE | |
Source | Hazardous Substances Data Bank (HSDB) | |
URL | https://pubchem.ncbi.nlm.nih.gov/source/hsdb/5567 | |
Description | The Hazardous Substances Data Bank (HSDB) is a toxicology database that focuses on the toxicology of potentially hazardous chemicals. It provides information on human exposure, industrial hygiene, emergency handling procedures, environmental fate, regulatory requirements, nanomaterials, and related areas. The information in HSDB has been assessed by a Scientific Review Panel. | |
Solubility |
SOL IN ETHER | |
Record name | DODECANOYL CHLORIDE | |
Source | Hazardous Substances Data Bank (HSDB) | |
URL | https://pubchem.ncbi.nlm.nih.gov/source/hsdb/5567 | |
Description | The Hazardous Substances Data Bank (HSDB) is a toxicology database that focuses on the toxicology of potentially hazardous chemicals. It provides information on human exposure, industrial hygiene, emergency handling procedures, environmental fate, regulatory requirements, nanomaterials, and related areas. The information in HSDB has been assessed by a Scientific Review Panel. | |
Color/Form |
WATER-WHITE LIQUID | |
CAS No. |
112-16-3 | |
Record name | Dodecanoyl chloride | |
Source | CAS Common Chemistry | |
URL | https://commonchemistry.cas.org/detail?cas_rn=112-16-3 | |
Description | CAS Common Chemistry is an open community resource for accessing chemical information. Nearly 500,000 chemical substances from CAS REGISTRY cover areas of community interest, including common and frequently regulated chemicals, and those relevant to high school and undergraduate chemistry classes. This chemical information, curated by our expert scientists, is provided in alignment with our mission as a division of the American Chemical Society. | |
Explanation | The data from CAS Common Chemistry is provided under a CC-BY-NC 4.0 license, unless otherwise stated. | |
Record name | Dodecanoyl chloride | |
Source | ChemIDplus | |
URL | https://pubchem.ncbi.nlm.nih.gov/substance/?source=chemidplus&sourceid=0000112163 | |
Description | ChemIDplus is a free, web search system that provides access to the structure and nomenclature authority files used for the identification of chemical substances cited in National Library of Medicine (NLM) databases, including the TOXNET system. | |
Record name | Lauroyl chloride | |
Source | DrugBank | |
URL | https://www.drugbank.ca/drugs/DB14670 | |
Description | The DrugBank database is a unique bioinformatics and cheminformatics resource that combines detailed drug (i.e. chemical, pharmacological and pharmaceutical) data with comprehensive drug target (i.e. sequence, structure, and pathway) information. | |
Explanation | Creative Common's Attribution-NonCommercial 4.0 International License (http://creativecommons.org/licenses/by-nc/4.0/legalcode) | |
Record name | Dodecanoyl chloride | |
Source | EPA Chemicals under the TSCA | |
URL | https://www.epa.gov/chemicals-under-tsca | |
Description | EPA Chemicals under the Toxic Substances Control Act (TSCA) collection contains information on chemicals and their regulations under TSCA, including non-confidential content from the TSCA Chemical Substance Inventory and Chemical Data Reporting. | |
Record name | Dodecanoyl chloride | |
Source | EPA DSSTox | |
URL | https://comptox.epa.gov/dashboard/DTXSID5044368 | |
Description | DSSTox provides a high quality public chemistry resource for supporting improved predictive toxicology. | |
Record name | Lauroyl chloride | |
Source | European Chemicals Agency (ECHA) | |
URL | https://echa.europa.eu/substance-information/-/substanceinfo/100.003.583 | |
Description | The European Chemicals Agency (ECHA) is an agency of the European Union which is the driving force among regulatory authorities in implementing the EU's groundbreaking chemicals legislation for the benefit of human health and the environment as well as for innovation and competitiveness. | |
Explanation | Use of the information, documents and data from the ECHA website is subject to the terms and conditions of this Legal Notice, and subject to other binding limitations provided for under applicable law, the information, documents and data made available on the ECHA website may be reproduced, distributed and/or used, totally or in part, for non-commercial purposes provided that ECHA is acknowledged as the source: "Source: European Chemicals Agency, http://echa.europa.eu/". Such acknowledgement must be included in each copy of the material. ECHA permits and encourages organisations and individuals to create links to the ECHA website under the following cumulative conditions: Links can only be made to webpages that provide a link to the Legal Notice page. | |
Record name | LAUROYL CHLORIDE | |
Source | FDA Global Substance Registration System (GSRS) | |
URL | https://gsrs.ncats.nih.gov/ginas/app/beta/substances/9LHL10777I | |
Description | The FDA Global Substance Registration System (GSRS) enables the efficient and accurate exchange of information on what substances are in regulated products. Instead of relying on names, which vary across regulatory domains, countries, and regions, the GSRS knowledge base makes it possible for substances to be defined by standardized, scientific descriptions. | |
Explanation | Unless otherwise noted, the contents of the FDA website (www.fda.gov), both text and graphics, are not copyrighted. They are in the public domain and may be republished, reprinted and otherwise used freely by anyone without the need to obtain permission from FDA. Credit to the U.S. Food and Drug Administration as the source is appreciated but not required. | |
Record name | DODECANOYL CHLORIDE | |
Source | Hazardous Substances Data Bank (HSDB) | |
URL | https://pubchem.ncbi.nlm.nih.gov/source/hsdb/5567 | |
Description | The Hazardous Substances Data Bank (HSDB) is a toxicology database that focuses on the toxicology of potentially hazardous chemicals. It provides information on human exposure, industrial hygiene, emergency handling procedures, environmental fate, regulatory requirements, nanomaterials, and related areas. The information in HSDB has been assessed by a Scientific Review Panel. | |
Melting Point |
-17 °C | |
Record name | DODECANOYL CHLORIDE | |
Source | Hazardous Substances Data Bank (HSDB) | |
URL | https://pubchem.ncbi.nlm.nih.gov/source/hsdb/5567 | |
Description | The Hazardous Substances Data Bank (HSDB) is a toxicology database that focuses on the toxicology of potentially hazardous chemicals. It provides information on human exposure, industrial hygiene, emergency handling procedures, environmental fate, regulatory requirements, nanomaterials, and related areas. The information in HSDB has been assessed by a Scientific Review Panel. | |
Synthesis routes and methods
Procedure details
Retrosynthesis Analysis
AI-Powered Synthesis Planning: Our tool employs the Template_relevance Pistachio, Template_relevance Bkms_metabolic, Template_relevance Pistachio_ringbreaker, Template_relevance Reaxys, Template_relevance Reaxys_biocatalysis model, leveraging a vast database of chemical reactions to predict feasible synthetic routes.
One-Step Synthesis Focus: Specifically designed for one-step synthesis, it provides concise and direct routes for your target compounds, streamlining the synthesis process.
Accurate Predictions: Utilizing the extensive PISTACHIO, BKMS_METABOLIC, PISTACHIO_RINGBREAKER, REAXYS, REAXYS_BIOCATALYSIS database, our tool offers high-accuracy predictions, reflecting the latest in chemical research and data.
Strategy Settings
Precursor scoring | Relevance Heuristic |
---|---|
Min. plausibility | 0.01 |
Model | Template_relevance |
Template Set | Pistachio/Bkms_metabolic/Pistachio_ringbreaker/Reaxys/Reaxys_biocatalysis |
Top-N result to add to graph | 6 |
Feasible Synthetic Routes
A: Lauroyl chloride has the molecular formula C12H23ClO and a molecular weight of 218.77 g/mol. []
A: Researchers commonly employ Fourier transform infrared spectroscopy (FTIR) and nuclear magnetic resonance (NMR) spectroscopy to characterize lauroyl chloride and its derivatives. FTIR analysis often reveals a characteristic peak for the carbonyl group (C=O) present in lauroyl chloride. [, , , , , ]
A: Lauroyl chloride primarily reacts with nucleophiles, such as alcohols and amines, through a nucleophilic acyl substitution mechanism. This reaction leads to the formation of esters and amides, respectively. [, , , , , ]
ANone: Lauroyl chloride finds use in various applications, including:
- Surface Modification: It can modify the surface properties of materials like cellulose, tourmaline powder, and wheat straw hemicelluloses, enhancing their hydrophobicity. [, , , , , ]
- Synthesis of Surfactants: Lauroyl chloride serves as a precursor for synthesizing various surfactants, including gemini surfactants, which exhibit unique properties. [, , ]
- Preparation of Biodegradable Materials: Researchers utilize lauroyl chloride to modify natural polymers like chitosan and cellulose, creating materials with potential applications in drug delivery and bioplastics. [, , , , ]
- Synthesis of Other Compounds: Lauroyl chloride acts as a building block for synthesizing various organic compounds, including pharmaceutical intermediates. [, , , , ]
A: Lauroyl chloride is soluble in various organic solvents like dimethylformamide (DMF), acetone, tetrahydrofuran (THF), and dichloromethane. [, , , ]
A: Increasing the DS of lauroyl chloride on cellulose generally enhances the hydrophobicity and tensile strength of the resulting material. [, ]
A: While lauroyl chloride itself might not act as a catalyst in typical reactions, it can be a component in catalyst systems. For instance, lauroyl peroxide, derived from lauroyl chloride, is a known polymerization catalyst. []
A: Modifying the alkyl chain length can significantly influence the properties of lauroyl chloride derivatives. For instance, increasing the chain length generally enhances the hydrophobicity and can impact the critical micelle concentration (CMC) in surfactants. [, , ]
A: Lauroyl chloride is susceptible to hydrolysis in the presence of water, leading to the formation of lauric acid and hydrochloric acid. Storage under anhydrous conditions is crucial for maintaining its stability. [, ]
A: Lauroyl chloride is corrosive and moisture-sensitive. Proper personal protective equipment, including gloves and eye protection, should be worn when handling this compound. It should be handled in a well-ventilated area to avoid inhalation. [, ]
Haftungsausschluss und Informationen zu In-Vitro-Forschungsprodukten
Bitte beachten Sie, dass alle Artikel und Produktinformationen, die auf BenchChem präsentiert werden, ausschließlich zu Informationszwecken bestimmt sind. Die auf BenchChem zum Kauf angebotenen Produkte sind speziell für In-vitro-Studien konzipiert, die außerhalb lebender Organismen durchgeführt werden. In-vitro-Studien, abgeleitet von dem lateinischen Begriff "in Glas", beinhalten Experimente, die in kontrollierten Laborumgebungen unter Verwendung von Zellen oder Geweben durchgeführt werden. Es ist wichtig zu beachten, dass diese Produkte nicht als Arzneimittel oder Medikamente eingestuft sind und keine Zulassung der FDA für die Vorbeugung, Behandlung oder Heilung von medizinischen Zuständen, Beschwerden oder Krankheiten erhalten haben. Wir müssen betonen, dass jede Form der körperlichen Einführung dieser Produkte in Menschen oder Tiere gesetzlich strikt untersagt ist. Es ist unerlässlich, sich an diese Richtlinien zu halten, um die Einhaltung rechtlicher und ethischer Standards in Forschung und Experiment zu gewährleisten.