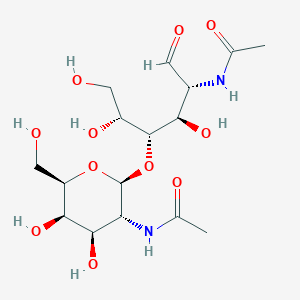
2-Acetamido-4-O-(2-acetamido-2-deoxygalactopyranosyl)-2-deoxyglucopyranose
- Klicken Sie auf QUICK INQUIRY, um ein Angebot von unserem Expertenteam zu erhalten.
- Mit qualitativ hochwertigen Produkten zu einem WETTBEWERBSFÄHIGEN Preis können Sie sich mehr auf Ihre Forschung konzentrieren.
Übersicht
Beschreibung
2-Acetamido-4-O-(2-acetamido-2-deoxygalactopyranosyl)-2-deoxyglucopyranose, commonly known as LacNAc, is a complex carbohydrate that plays a crucial role in various biological processes. It is a disaccharide composed of N-acetylgalactosamine (GalNAc) and N-acetylglucosamine (GlcNAc) and is found in the glycan chains of various glycoproteins and glycolipids. LacNAc has been the subject of extensive research due to its unique structure and biological functions.
Wissenschaftliche Forschungsanwendungen
Presence in Pluripotent Cells
This compound is a glycan that is found in pluripotent cells . Pluripotent cells have the ability to differentiate into almost any type of cell in the body. Therefore, this compound could potentially play a role in stem cell research and regenerative medicine.
Biological Properties
The compound has been shown to have biological properties that inhibit leukemia inhibitory factor . This suggests that it could potentially be used in the treatment of certain types of cancer.
Role in Peptidoglycan Synthesis
2-Acetamido-4-O-(2-acetamido-2-deoxygalactopyranosyl)-2-deoxyglucopyranose is a pivotal compound in biomedicine, embracing a momentous function as a glycosylated constituent during peptidoglycan synthesis . Peptidoglycan is a major component of the cell wall of bacteria, providing structural strength and protecting the cell from osmotic lysis.
Potential Therapeutic Applications
Its versatile applications encompass the creation of therapeutic agents that combat bacterial infections or dysfunctions arising from compromised peptidoglycan metabolism . This suggests that it could be used to develop new antibiotics or treatments for conditions such as inflammatory bowel disease, which can be caused by imbalances in the gut microbiota.
Enzymatic Synthesis
The compound can be synthesized enzymatically, as demonstrated by a study that used the beta-N-acetylhexosaminidase enzyme from Aspergillus oryzae . This suggests potential applications in biotechnology and industrial processes, where enzymatic synthesis can offer advantages such as mild reaction conditions, high selectivity, and environmental friendliness.
Pharmaceutical Testing
The compound is used for pharmaceutical testing . It can serve as a reference standard for accurate results in pharmaceutical analysis and quality control.
Eigenschaften
IUPAC Name |
N-[(2S,3R,4R,5R,6R)-2-[(2R,3S,4R,5R)-5-acetamido-1,2,4-trihydroxy-6-oxohexan-3-yl]oxy-4,5-dihydroxy-6-(hydroxymethyl)oxan-3-yl]acetamide |
Source
|
---|---|---|
Source | PubChem | |
URL | https://pubchem.ncbi.nlm.nih.gov | |
Description | Data deposited in or computed by PubChem | |
InChI |
InChI=1S/C16H28N2O11/c1-6(22)17-8(3-19)12(25)15(9(24)4-20)29-16-11(18-7(2)23)14(27)13(26)10(5-21)28-16/h3,8-16,20-21,24-27H,4-5H2,1-2H3,(H,17,22)(H,18,23)/t8-,9+,10+,11+,12+,13-,14+,15+,16-/m0/s1 |
Source
|
Source | PubChem | |
URL | https://pubchem.ncbi.nlm.nih.gov | |
Description | Data deposited in or computed by PubChem | |
InChI Key |
PLJAKLUDUPBLGD-HFMMELOMSA-N |
Source
|
Source | PubChem | |
URL | https://pubchem.ncbi.nlm.nih.gov | |
Description | Data deposited in or computed by PubChem | |
Canonical SMILES |
CC(=O)NC1C(C(C(OC1OC(C(CO)O)C(C(C=O)NC(=O)C)O)CO)O)O |
Source
|
Source | PubChem | |
URL | https://pubchem.ncbi.nlm.nih.gov | |
Description | Data deposited in or computed by PubChem | |
Isomeric SMILES |
CC(=O)N[C@@H]1[C@H]([C@H]([C@H](O[C@H]1O[C@H]([C@@H](CO)O)[C@@H]([C@H](C=O)NC(=O)C)O)CO)O)O |
Source
|
Source | PubChem | |
URL | https://pubchem.ncbi.nlm.nih.gov | |
Description | Data deposited in or computed by PubChem | |
Molecular Formula |
C16H28N2O11 |
Source
|
Source | PubChem | |
URL | https://pubchem.ncbi.nlm.nih.gov | |
Description | Data deposited in or computed by PubChem | |
Molecular Weight |
424.40 g/mol |
Source
|
Source | PubChem | |
URL | https://pubchem.ncbi.nlm.nih.gov | |
Description | Data deposited in or computed by PubChem | |
Product Name |
2-Acetamido-4-O-(2-acetamido-2-deoxygalactopyranosyl)-2-deoxyglucopyranose | |
CAS RN |
136198-41-9 |
Source
|
Record name | N-Acetylgalactosaminyl-1-4-N-acetylglucosamine | |
Source | ChemIDplus | |
URL | https://pubchem.ncbi.nlm.nih.gov/substance/?source=chemidplus&sourceid=0136198419 | |
Description | ChemIDplus is a free, web search system that provides access to the structure and nomenclature authority files used for the identification of chemical substances cited in National Library of Medicine (NLM) databases, including the TOXNET system. | |
Haftungsausschluss und Informationen zu In-Vitro-Forschungsprodukten
Bitte beachten Sie, dass alle Artikel und Produktinformationen, die auf BenchChem präsentiert werden, ausschließlich zu Informationszwecken bestimmt sind. Die auf BenchChem zum Kauf angebotenen Produkte sind speziell für In-vitro-Studien konzipiert, die außerhalb lebender Organismen durchgeführt werden. In-vitro-Studien, abgeleitet von dem lateinischen Begriff "in Glas", beinhalten Experimente, die in kontrollierten Laborumgebungen unter Verwendung von Zellen oder Geweben durchgeführt werden. Es ist wichtig zu beachten, dass diese Produkte nicht als Arzneimittel oder Medikamente eingestuft sind und keine Zulassung der FDA für die Vorbeugung, Behandlung oder Heilung von medizinischen Zuständen, Beschwerden oder Krankheiten erhalten haben. Wir müssen betonen, dass jede Form der körperlichen Einführung dieser Produkte in Menschen oder Tiere gesetzlich strikt untersagt ist. Es ist unerlässlich, sich an diese Richtlinien zu halten, um die Einhaltung rechtlicher und ethischer Standards in Forschung und Experiment zu gewährleisten.