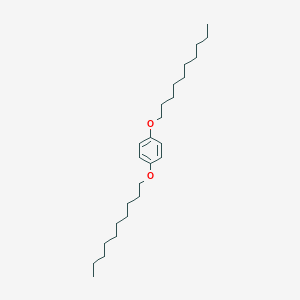
1,4-Bis(decyloxy)benzene
Übersicht
Beschreibung
1,4-Bis(decyloxy)benzene is an organic compound with the molecular formula C26H46O2. It is a derivative of benzene, where two decyloxy groups are attached to the 1 and 4 positions of the benzene ring. This compound is known for its liquid crystalline properties and is used in various applications, including materials science and organic electronics.
Vorbereitungsmethoden
Synthetic Routes and Reaction Conditions
1,4-Bis(decyloxy)benzene can be synthesized through the Williamson ether synthesis. The general reaction involves the reaction of 1,4-dihydroxybenzene with decyl bromide in the presence of a strong base such as potassium carbonate. The reaction is typically carried out in an aprotic solvent like dimethylformamide (DMF) at elevated temperatures.
Reaction:
C6H4(OH)2+2C10H21Br→C6H4(OC10H21)2+2HBr
Industrial Production Methods
Industrial production of this compound follows similar synthetic routes but on a larger scale. The process involves the use of continuous flow reactors to ensure efficient mixing and heat transfer. The reaction conditions are optimized to maximize yield and purity, and the product is purified through distillation or recrystallization.
Analyse Chemischer Reaktionen
Types of Reactions
1,4-Bis(decyloxy)benzene primarily undergoes substitution reactions due to the presence of the aromatic ring. It can also participate in oxidation and reduction reactions under specific conditions.
Common Reagents and Conditions
Substitution Reactions: Electrophilic aromatic substitution reactions can occur with reagents such as bromine (Br2) in the presence of a catalyst like iron(III) bromide (FeBr3).
Oxidation Reactions: Oxidation can be carried out using strong oxidizing agents like potassium permanganate (KMnO4) or chromium trioxide (CrO3).
Reduction Reactions: Reduction can be achieved using reducing agents like lithium aluminum hydride (LiAlH4) or hydrogen gas (H2) in the presence of a palladium catalyst.
Major Products
Substitution: Halogenated derivatives such as 1,4-bis(decyloxy)-2-bromobenzene.
Oxidation: Carboxylic acid derivatives.
Reduction: Hydrogenated derivatives.
Wissenschaftliche Forschungsanwendungen
Organic Synthesis
Building Block for Complex Molecules
1,4-Bis(decyloxy)benzene serves as a versatile building block in organic synthesis. It can undergo various chemical reactions, including:
- Nucleophilic Substitution : The compound can be modified to introduce other functional groups, enhancing its utility in creating complex organic molecules.
- Coupling Reactions : It is utilized in coupling reactions, such as Suzuki-Miyaura coupling, to form biaryl compounds, which are important in pharmaceuticals and materials science .
Materials Science
Liquid Crystals and Organic Electronics
The compound is significant in the development of liquid crystals and organic electronics. Its structural characteristics allow it to be incorporated into:
- Liquid Crystal Displays (LCDs) : this compound derivatives exhibit favorable mesomorphic properties, making them suitable for LCD applications.
- Organic Light-Emitting Diodes (OLEDs) : The compound is used as a precursor in synthesizing conjugated polymers for OLEDs, contributing to efficient light emission .
Medicinal Chemistry
Antimicrobial and Anticancer Activities
Research indicates that this compound derivatives possess notable biological activities:
- Antimicrobial Properties : Studies have shown that derivatives exhibit bacteriostatic effects against Gram-negative bacteria like Escherichia coli, with minimum inhibitory concentrations (MIC) ranging from 135 µg/mL to 145 µg/mL depending on the alkyl chain length .
- Anticancer Activity : The compound has demonstrated the ability to inhibit the proliferation of various cancer cell lines, suggesting potential as an anticancer agent. Mechanistically, it induces apoptosis via specific signaling pathways .
Chemical Sensors
Detection of Chemical Species
this compound is also being explored for its potential in chemical sensing applications:
- Sensor Development : Its ability to interact with various chemical species makes it a candidate for developing sensors that can detect environmental pollutants or biological markers.
Data Summary
Application Area | Specific Use Cases | Key Findings/Properties |
---|---|---|
Organic Synthesis | Building block for complex molecules | Versatile reactivity; used in nucleophilic substitutions |
Materials Science | Liquid crystals; OLEDs | Exhibits favorable mesomorphic properties; efficient light emission |
Medicinal Chemistry | Antimicrobial; anticancer | Effective against E. coli; induces apoptosis in cancer cells |
Chemical Sensors | Detection of pollutants | Potential for environmental monitoring |
Case Study 1: Antimicrobial Activity
A study evaluating the antibacterial properties of synthesized bis(thiourea) derivatives derived from this compound showed significant activity against E. coli. The presence of long alkyl chains enhanced lipophilicity and facilitated membrane disruption, leading to effective antibacterial action .
Case Study 2: Anticancer Properties
In vitro studies on human cancer cell lines treated with this compound revealed a significant reduction in cell viability after 48 hours. The IC50 values varied across different cell lines, indicating its potential as a therapeutic agent in cancer treatment .
Wirkmechanismus
The mechanism of action of 1,4-Bis(decyloxy)benzene is primarily related to its ability to form liquid crystalline phases. The decyloxy groups provide flexibility and hydrophobicity, which facilitate the alignment of molecules in a liquid crystalline state. This alignment is crucial for its applications in organic electronics, where the ordered structure enhances charge transport properties.
Vergleich Mit ähnlichen Verbindungen
Similar Compounds
- 1,4-Bis(octyloxy)benzene
- 1,4-Bis(dodecyloxy)benzene
- 1,4-Bis(hexadecyloxy)benzene
Comparison
1,4-Bis(decyloxy)benzene is unique due to its specific chain length of the decyloxy groups, which provides an optimal balance between flexibility and rigidity. This balance is essential for forming stable liquid crystalline phases. In comparison, 1,4-Bis(octyloxy)benzene has shorter alkyl chains, resulting in lower melting points and less stable liquid crystalline phases. On the other hand, 1,4-Bis(dodecyloxy)benzene and 1,4-Bis(hexadecyloxy)benzene have longer alkyl chains, which can lead to higher melting points and increased viscosity, affecting the material’s processability.
Biologische Aktivität
1,4-Bis(decyloxy)benzene is an organic compound characterized by its unique structure, which includes two decyloxy groups attached to a benzene ring. This compound has garnered interest in various fields, particularly in medicinal chemistry and materials science, due to its potential biological activities. This article aims to explore the biological activity of this compound, summarizing research findings, case studies, and relevant data.
Synthesis of this compound
The synthesis of this compound typically involves the Williamson ether synthesis method. In a notable study, hydroquinone was reacted with bromodecane in the presence of potassium hydroxide (KOH) and dimethyl sulfoxide (DMSO). The reaction yielded a high purity product, confirmed by NMR spectroscopy .
Biological Activity Overview
This compound exhibits several biological activities that are primarily attributed to its structural properties. The following sections detail specific activities and findings from various studies.
Antimicrobial Activity
Research indicates that derivatives of this compound have significant antimicrobial properties. For instance, bis(thiourea) derivatives synthesized from this compound demonstrated effective bacteriostatic activity against Gram-negative bacteria such as Escherichia coli. The minimum inhibitory concentration (MIC) for some derivatives was reported to be as low as 135 µg/mL .
Table 1: Antimicrobial Activity of this compound Derivatives
Compound | MIC (µg/mL) | Target Organism |
---|---|---|
This compound | - | - |
Bis(thiourea derivative n=10) | 135 | E. coli |
Bis(thiourea derivative n=12) | 145 | E. coli |
Cytotoxicity and Cancer Research
The cytotoxic effects of this compound have been explored in various cancer cell lines. Studies have shown that the compound can induce apoptosis in cancer cells through mechanisms involving oxidative stress and the disruption of mitochondrial function. This property makes it a candidate for further investigation in cancer therapy .
Case Study: Apoptosis Induction
In vitro studies demonstrated that treatment with this compound resulted in increased levels of reactive oxygen species (ROS), leading to cell death in specific cancer cell lines. This effect was dose-dependent and highlighted the potential for developing therapeutic agents based on this compound .
The biological activity of this compound can be attributed to several mechanisms:
- Lipophilicity : The long alkyl chains enhance the lipophilicity of the molecule, facilitating its incorporation into cellular membranes and affecting membrane integrity.
- Reactive Oxygen Species Generation : The compound can induce oxidative stress within cells, leading to apoptosis.
- Interaction with Cellular Targets : It may interact with proteins involved in cell signaling pathways related to proliferation and apoptosis.
Eigenschaften
IUPAC Name |
1,4-didecoxybenzene | |
---|---|---|
Source | PubChem | |
URL | https://pubchem.ncbi.nlm.nih.gov | |
Description | Data deposited in or computed by PubChem | |
InChI |
InChI=1S/C26H46O2/c1-3-5-7-9-11-13-15-17-23-27-25-19-21-26(22-20-25)28-24-18-16-14-12-10-8-6-4-2/h19-22H,3-18,23-24H2,1-2H3 | |
Source | PubChem | |
URL | https://pubchem.ncbi.nlm.nih.gov | |
Description | Data deposited in or computed by PubChem | |
InChI Key |
MMGPQYJNRFGRQK-UHFFFAOYSA-N | |
Source | PubChem | |
URL | https://pubchem.ncbi.nlm.nih.gov | |
Description | Data deposited in or computed by PubChem | |
Canonical SMILES |
CCCCCCCCCCOC1=CC=C(C=C1)OCCCCCCCCCC | |
Source | PubChem | |
URL | https://pubchem.ncbi.nlm.nih.gov | |
Description | Data deposited in or computed by PubChem | |
Molecular Formula |
C26H46O2 | |
Source | PubChem | |
URL | https://pubchem.ncbi.nlm.nih.gov | |
Description | Data deposited in or computed by PubChem | |
DSSTOX Substance ID |
DTXSID00373004 | |
Record name | 1,4-Bis(decyloxy)benzene | |
Source | EPA DSSTox | |
URL | https://comptox.epa.gov/dashboard/DTXSID00373004 | |
Description | DSSTox provides a high quality public chemistry resource for supporting improved predictive toxicology. | |
Molecular Weight |
390.6 g/mol | |
Source | PubChem | |
URL | https://pubchem.ncbi.nlm.nih.gov | |
Description | Data deposited in or computed by PubChem | |
CAS No. |
129236-97-1 | |
Record name | 1,4-Bis(decyloxy)benzene | |
Source | EPA DSSTox | |
URL | https://comptox.epa.gov/dashboard/DTXSID00373004 | |
Description | DSSTox provides a high quality public chemistry resource for supporting improved predictive toxicology. | |
Synthesis routes and methods
Procedure details
Retrosynthesis Analysis
AI-Powered Synthesis Planning: Our tool employs the Template_relevance Pistachio, Template_relevance Bkms_metabolic, Template_relevance Pistachio_ringbreaker, Template_relevance Reaxys, Template_relevance Reaxys_biocatalysis model, leveraging a vast database of chemical reactions to predict feasible synthetic routes.
One-Step Synthesis Focus: Specifically designed for one-step synthesis, it provides concise and direct routes for your target compounds, streamlining the synthesis process.
Accurate Predictions: Utilizing the extensive PISTACHIO, BKMS_METABOLIC, PISTACHIO_RINGBREAKER, REAXYS, REAXYS_BIOCATALYSIS database, our tool offers high-accuracy predictions, reflecting the latest in chemical research and data.
Strategy Settings
Precursor scoring | Relevance Heuristic |
---|---|
Min. plausibility | 0.01 |
Model | Template_relevance |
Template Set | Pistachio/Bkms_metabolic/Pistachio_ringbreaker/Reaxys/Reaxys_biocatalysis |
Top-N result to add to graph | 6 |
Feasible Synthetic Routes
Haftungsausschluss und Informationen zu In-Vitro-Forschungsprodukten
Bitte beachten Sie, dass alle Artikel und Produktinformationen, die auf BenchChem präsentiert werden, ausschließlich zu Informationszwecken bestimmt sind. Die auf BenchChem zum Kauf angebotenen Produkte sind speziell für In-vitro-Studien konzipiert, die außerhalb lebender Organismen durchgeführt werden. In-vitro-Studien, abgeleitet von dem lateinischen Begriff "in Glas", beinhalten Experimente, die in kontrollierten Laborumgebungen unter Verwendung von Zellen oder Geweben durchgeführt werden. Es ist wichtig zu beachten, dass diese Produkte nicht als Arzneimittel oder Medikamente eingestuft sind und keine Zulassung der FDA für die Vorbeugung, Behandlung oder Heilung von medizinischen Zuständen, Beschwerden oder Krankheiten erhalten haben. Wir müssen betonen, dass jede Form der körperlichen Einführung dieser Produkte in Menschen oder Tiere gesetzlich strikt untersagt ist. Es ist unerlässlich, sich an diese Richtlinien zu halten, um die Einhaltung rechtlicher und ethischer Standards in Forschung und Experiment zu gewährleisten.