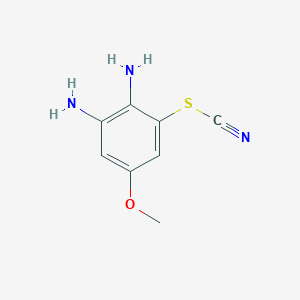
2,3-Diamino-5-methoxyphenyl thiocyanate
Übersicht
Beschreibung
2,3-Diamino-5-methoxyphenyl thiocyanate (CAS No. 1192806-19-1) is an organic compound . It has a molecular formula of C8H9N3OS and a molecular weight of 195.24 g/mol .
Physical And Chemical Properties Analysis
The boiling point and other specific physical and chemical properties of 2,3-Diamino-5-methoxyphenyl thiocyanate are not provided in the search results .Wissenschaftliche Forschungsanwendungen
Synthesis of Triazine Derivatives
Triazines are a class of nitrogen-containing heterocycles that have significant applications in various fields. The compound 2,3-Diamino-5-methoxyphenyl thiocyanate can be utilized in the synthesis of 1,3,5-triazines, which are known for their use in the production of herbicides and polymer photostabilizers . These triazine derivatives also exhibit biological properties, such as antitumor activities, making them valuable in pharmaceutical research.
Antitumor Properties
Some 1,3,5-triazines display important biological properties. For example, derivatives of these compounds are used clinically to treat lung, breast, and ovarian cancer due to their antitumor properties . The versatility of 2,3-Diamino-5-methoxyphenyl thiocyanate in forming these structures makes it a crucial starting material in cancer research.
Aromatase Inhibitory Activity
Recent studies have shown that 1,3,5-triazines exhibit significant aromatase inhibitory activity. This is crucial in the treatment of hormone-sensitive cancers, as aromatase inhibitors are used to decrease the amount of estrogen in the body and slow the growth of cancers . The compound could be a key ingredient in developing new aromatase inhibitors.
Siderophore-Mediated Drug Development
The compound has potential use in siderophore-mediated drug development. Siderophores are molecules that bind and transport iron in microorganisms, and their analogs can be used to deliver drugs in a targeted fashion, particularly in treating infections .
Corticotrophin-Releasing Factor 1 Receptor Antagonist
Structures derived from 2,3-Diamino-5-methoxyphenyl thiocyanate have shown potent activity as corticotrophin-releasing factor 1 receptor antagonists. These are promising for the treatment of depression and anxiety, as they can modulate the stress response in the body .
Leukotriene C4 Antagonist
Compounds synthesized from 2,3-Diamino-5-methoxyphenyl thiocyanate have been found to have potent activity against leukotriene C4 (LTC4) antagonist, which possess a protective effect on HCl.ethanol-induced gastric lesions . This suggests its potential application in developing treatments for gastrointestinal disorders.
Antiparasitic Activity
The substrate derived from 2,3-Diamino-5-methoxyphenyl thiocyanate presents good in vitro activity against the protozoan parasite Trypanosoma brucei, the causative organism of Human African Trypanosomiasis . This opens up avenues for research into new antiparasitic drugs.
Polymer Photostabilizers
The triazine derivatives obtained from this compound can be used as photostabilizers in polymers. These additives can enhance the durability and lifespan of polymers by protecting them from UV light degradation .
Safety and Hazards
Eigenschaften
IUPAC Name |
(2,3-diamino-5-methoxyphenyl) thiocyanate | |
---|---|---|
Source | PubChem | |
URL | https://pubchem.ncbi.nlm.nih.gov | |
Description | Data deposited in or computed by PubChem | |
InChI |
InChI=1S/C8H9N3OS/c1-12-5-2-6(10)8(11)7(3-5)13-4-9/h2-3H,10-11H2,1H3 | |
Source | PubChem | |
URL | https://pubchem.ncbi.nlm.nih.gov | |
Description | Data deposited in or computed by PubChem | |
InChI Key |
QORDBEUMBHJPQQ-UHFFFAOYSA-N | |
Source | PubChem | |
URL | https://pubchem.ncbi.nlm.nih.gov | |
Description | Data deposited in or computed by PubChem | |
Canonical SMILES |
COC1=CC(=C(C(=C1)SC#N)N)N | |
Source | PubChem | |
URL | https://pubchem.ncbi.nlm.nih.gov | |
Description | Data deposited in or computed by PubChem | |
Molecular Formula |
C8H9N3OS | |
Source | PubChem | |
URL | https://pubchem.ncbi.nlm.nih.gov | |
Description | Data deposited in or computed by PubChem | |
Molecular Weight |
195.24 g/mol | |
Source | PubChem | |
URL | https://pubchem.ncbi.nlm.nih.gov | |
Description | Data deposited in or computed by PubChem | |
Product Name |
2,3-Diamino-5-methoxyphenyl thiocyanate |
Retrosynthesis Analysis
AI-Powered Synthesis Planning: Our tool employs the Template_relevance Pistachio, Template_relevance Bkms_metabolic, Template_relevance Pistachio_ringbreaker, Template_relevance Reaxys, Template_relevance Reaxys_biocatalysis model, leveraging a vast database of chemical reactions to predict feasible synthetic routes.
One-Step Synthesis Focus: Specifically designed for one-step synthesis, it provides concise and direct routes for your target compounds, streamlining the synthesis process.
Accurate Predictions: Utilizing the extensive PISTACHIO, BKMS_METABOLIC, PISTACHIO_RINGBREAKER, REAXYS, REAXYS_BIOCATALYSIS database, our tool offers high-accuracy predictions, reflecting the latest in chemical research and data.
Strategy Settings
Precursor scoring | Relevance Heuristic |
---|---|
Min. plausibility | 0.01 |
Model | Template_relevance |
Template Set | Pistachio/Bkms_metabolic/Pistachio_ringbreaker/Reaxys/Reaxys_biocatalysis |
Top-N result to add to graph | 6 |
Feasible Synthetic Routes
Haftungsausschluss und Informationen zu In-Vitro-Forschungsprodukten
Bitte beachten Sie, dass alle Artikel und Produktinformationen, die auf BenchChem präsentiert werden, ausschließlich zu Informationszwecken bestimmt sind. Die auf BenchChem zum Kauf angebotenen Produkte sind speziell für In-vitro-Studien konzipiert, die außerhalb lebender Organismen durchgeführt werden. In-vitro-Studien, abgeleitet von dem lateinischen Begriff "in Glas", beinhalten Experimente, die in kontrollierten Laborumgebungen unter Verwendung von Zellen oder Geweben durchgeführt werden. Es ist wichtig zu beachten, dass diese Produkte nicht als Arzneimittel oder Medikamente eingestuft sind und keine Zulassung der FDA für die Vorbeugung, Behandlung oder Heilung von medizinischen Zuständen, Beschwerden oder Krankheiten erhalten haben. Wir müssen betonen, dass jede Form der körperlichen Einführung dieser Produkte in Menschen oder Tiere gesetzlich strikt untersagt ist. Es ist unerlässlich, sich an diese Richtlinien zu halten, um die Einhaltung rechtlicher und ethischer Standards in Forschung und Experiment zu gewährleisten.