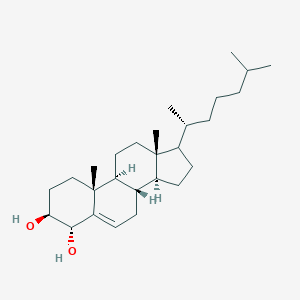
4alpha-Hydroxy Cholesterol
Übersicht
Beschreibung
4α-Hydroxy cholesterol (4α-OHC) is a hydroxylated derivative of cholesterol, characterized by a hydroxyl group (-OH) at the 4α position of the sterol backbone. Structurally, it belongs to the class of cholestane steroids and shares the core 3β-hydroxyl-Δ⁵-steroid framework with cholesterol . Unlike its isomer 4β-hydroxycholesterol (4β-OHC), which is a well-established biomarker for cytochrome P450 3A (CYP3A) enzyme activity, 4α-OHC is primarily associated with oxidative stress and non-enzymatic oxidation processes .
Vorbereitungsmethoden
Chemical Synthesis of 4α-Hydroxycholesterol
Direct Oxidation of Cholesterol
The most straightforward method for synthesizing 4α-HC involves the chemical oxidation of cholesterol. This process typically employs oxidizing agents such as tert-butyl hydroperoxide (TBHP) or hydrogen peroxide (H₂O₂) under controlled conditions. The reaction proceeds via radical-mediated mechanisms, where reactive oxygen species abstract a hydrogen atom from the C4 position of cholesterol, leading to hydroxylation at the 4α site .
Key Reaction Conditions:
-
Solvent System: A mixture of dichloromethane (DCM) and methanol (MeOH) in a 3:1 ratio.
-
Temperature: 40–60°C to optimize radical formation without degrading the sterol backbone.
-
Catalyst: Transition metals such as iron(II) sulfate (FeSO₄) or copper(I) chloride (CuCl) to accelerate radical generation .
Mechanistic Insights:
The oxidation begins with the abstraction of a hydrogen atom from C4, forming a carbon-centered radical. Subsequent reaction with molecular oxygen (O₂) generates a peroxyl radical, which undergoes disproportionation to yield the 4α-hydroxylated product. Stereochemical control is challenging, as competing pathways may produce 4β-HC. To mitigate this, steric hindrance from the cholesterol side chain favors hydroxylation at the less hindered 4α position .
Yield and Purity:
Typical yields range from 15–30%, with purity exceeding 90% after silica gel chromatography. Impurities often include 4β-HC, 7-ketocholesterol, and epoxycholesterol derivatives .
Stereoselective Hydroxylation Using Chiral Auxiliaries
To improve stereochemical control, chiral auxiliaries or directing groups can be employed. For example, the Weitz-Scheffer epoxidation has been adapted to guide hydroxylation to the 4α position.
Procedure:
-
Epoxidation: Cholesterol is treated with meta-chloroperbenzoic acid (mCPBA) to form 5,6α-epoxycholesterol.
-
Acid-Catalyzed Ring Opening: The epoxide is hydrolyzed using boron trifluoride etherate (BF₃·OEt₂) in aqueous tetrahydrofuran (THF), directing hydroxylation to the 4α position .
Advantages:
-
Higher stereoselectivity (up to 70% 4α-HC).
-
Reduced formation of 4β-HC and other byproducts.
Limitations:
-
Multi-step synthesis increases complexity.
-
Requires rigorous purification to isolate 4α-HC from diastereomers .
Industrial-Scale Production Strategies
Microbial Biotransformation
Recent advances in synthetic biology have enabled the use of engineered Saccharomyces cerevisiae strains to produce 4α-HC. These strains overexpress cytochrome P450 reductase (CPR) and ferredoxin to enhance hydroxylation activity.
Optimized Conditions:
-
Substrate: Cholesterol dissolved in β-cyclodextrin to improve solubility.
-
Culture Medium: YPD (yeast extract-peptone-dextrose) supplemented with 0.1% Tween-80.
Challenges:
-
Competition from endogenous ergosterol biosynthesis.
Comparative Analysis of Preparation Methods
Method | Yield (%) | Purity (%) | Stereoselectivity | Scalability |
---|---|---|---|---|
Chemical Oxidation | 15–30 | 90–95 | Low | Moderate |
Chiral Auxiliaries | 40–70 | 85–90 | High | Low |
Microbial Biosynthesis | 50–80 | 95–98 | Moderate | High |
Table 1. Comparison of 4α-HC synthesis methods. Microbial biosynthesis offers the best balance of yield and scalability but requires specialized bioreactor setups .
Analyse Chemischer Reaktionen
Types of Reactions: 4alpha-Hydroxy Cholesterol undergoes various chemical reactions, including:
Oxidation: Further oxidation can lead to the formation of other oxysterols.
Reduction: Reduction reactions can convert it back to cholesterol or other derivatives.
Substitution: Functional groups on the molecule can be substituted with other groups under specific conditions.
Common Reagents and Conditions:
Oxidation: Common oxidizing agents include molecular oxygen and hydrogen peroxide.
Reduction: Reducing agents such as sodium borohydride or lithium aluminum hydride are used.
Substitution: Reagents like alkyl halides or acyl chlorides can be used for substitution reactions.
Major Products: The major products formed from these reactions include various oxysterols, which have distinct biological activities and functions .
Wissenschaftliche Forschungsanwendungen
Biochemical Role and Mechanism of Action
4α-HC plays a significant role in lipid metabolism and homeostasis. It is known to influence the expression of genes involved in cholesterol and lipid transport and metabolism. The compound acts as a signaling molecule that can modulate the activity of liver X receptors (LXRs), which are crucial for regulating cholesterol homeostasis.
- Mechanism : 4α-HC is thought to activate LXRs, leading to the induction of genes responsible for cholesterol efflux (e.g., ATP-binding cassette transporter A1, ABCA1) and repressing cholesterol influx by inhibiting transporters such as lectin-like oxidized LDL receptor-1 .
Applications in Drug Development
The compound has emerged as a valuable biomarker in pharmacokinetic studies, particularly in assessing drug-drug interactions (DDIs) involving cytochrome P450 enzymes.
- CYP3A Activity Marker : 4α-HC levels have been used to evaluate CYP3A enzyme activity in clinical trials. For instance, studies have shown that potent CYP3A inhibitors like ketoconazole significantly decrease plasma levels of 4α-HC, while inducers like rifampicin markedly increase its levels .
Table 1: Effects of CYP3A Modulators on Plasma 4α-HC Levels
Treatment | Change in 4α-HC Levels | Statistical Significance |
---|---|---|
Ketoconazole | Decreased by 13% | |
Rifampicin | Increased by 220% | |
Placebo | No significant change | N/A |
Clinical Implications
The elevation of 4α-HC levels has been linked to various clinical conditions, including liver diseases and metabolic disorders. Its role as a biomarker for hepatic function is being explored.
- Liver Disease : Elevated levels of 4α-HC may indicate altered cholesterol metabolism in liver diseases, providing insights into disease progression and treatment efficacy .
Case Studies
Several studies have documented the use of 4α-HC as a biomarker in clinical settings:
- Study on Drug Interactions : A clinical trial involving healthy subjects assessed the impact of rifampicin and ketoconazole on plasma levels of midazolam and its correlation with changes in 4α-HC levels. The results indicated that monitoring 4α-HC could serve as a reliable surrogate marker for evaluating CYP3A activity during drug development .
- Cholesterol Metabolism Study : Research demonstrated that individuals with specific genetic polymorphisms affecting CYP3A enzymes exhibited altered plasma levels of 4α-HC, suggesting its potential use in personalized medicine approaches for optimizing drug therapy .
Wirkmechanismus
4alpha-Hydroxy Cholesterol exerts its effects primarily through its interaction with liver X receptors (LXRs), which are nuclear receptors involved in the regulation of lipid metabolism . Upon binding to LXRs, it modulates the expression of genes involved in cholesterol transport, efflux, and homeostasis. This pathway is crucial for maintaining cellular cholesterol levels and preventing the accumulation of cholesterol in tissues .
Vergleich Mit ähnlichen Verbindungen
Structural and Functional Differences
The following table summarizes key structural and functional distinctions between 4α-OHC and related hydroxycholesterols:
Key Research Findings
4α-OHC vs. 4β-OHC in CKD
- Plasma Levels : In CKD patients with CYP3A51 allele, plasma 4β-OHC concentrations significantly decreased with disease progression (49.3 ± 16.0 ng/mL in stage 3 vs. 30.2 ± 15.6 ng/mL in stage 4–5D; *p = 0.0053), while 4α-OHC levels remained stable (median ~6.0 ng/mL; p = 0.32) .
4α-OHC vs. 7α-OHC in Metabolic Pathways
- 7α-OHC is a critical intermediate in bile acid synthesis, regulated by CYP7A1. Mutations in CYP7A1 cause hypercholesterolemia and gallstone formation, whereas 4α-OHC lacks direct involvement in this pathway .
Oxidative Stress Markers
- Both 4α-OHC and 7β-OHC are products of oxidative stress, but 7β-OHC is more strongly linked to inflammation and neurodegeneration. In contrast, 4α-OHC’s role in oxidative pathways is less characterized .
Discussion of Key Contrasts
- Enzymatic vs. Non-Enzymatic Origins: 4β-OHC and 7α-OHC are enzymatically derived (CYP3A and CYP7A1, respectively), whereas 4α-OHC arises predominantly from non-enzymatic oxidation, limiting its specificity as a metabolic marker .
- Biomarker Relevance: 4β-OHC is clinically validated for CYP3A phenotyping, while 4α-OHC’s utility is confined to contextualizing 4β-OHC measurements in specific populations (e.g., CKD) .
Biologische Aktivität
4α-Hydroxy cholesterol (4α-HC) is an oxysterol, a derivative of cholesterol that has gained attention due to its potential biological activities and implications in various metabolic processes. This article explores the biological activity of 4α-HC, focusing on its role as a ligand for nuclear receptors, its influence on lipid metabolism, and its potential as a biomarker in pharmacokinetics.
4α-HC is synthesized from cholesterol through enzymatic oxidation, primarily by cytochrome P450 enzymes. It is structurally similar to other oxysterols, such as 4β-hydroxy cholesterol (4β-HC), but exhibits distinct biological properties. The compound's chemical structure allows it to interact with various nuclear receptors, particularly the Liver X Receptor (LXR), which plays a crucial role in lipid homeostasis.
Lipid Metabolism Regulation
Research indicates that 4α-HC may act as a ligand for LXR, influencing lipid metabolism pathways. Studies have shown that 4α-HC can modulate the expression of genes involved in lipid synthesis and transport:
- Activation of LXR : 4α-HC activates LXR, leading to the transcriptional regulation of genes involved in fatty acid synthesis and cholesterol metabolism. This activation can promote lipogenic processes, similar to the effects observed with 4β-HC .
- Impact on SREBP Pathways : While 4β-HC selectively induces the master lipogenic transcription factor Sterol Regulatory Element Binding Protein 1c (SREBP1c), the specific effects of 4α-HC on SREBP pathways require further investigation. However, it is hypothesized that like other oxysterols, it may influence SREBP activity indirectly through LXR activation.
Potential as a Biomarker
4α-HC has been proposed as an endogenous biomarker for assessing cytochrome P450 3A (CYP3A) activity. Its plasma concentrations can reflect changes in CYP3A activity due to drug interactions:
- Drug Interaction Studies : Clinical studies have demonstrated that plasma levels of 4α-HC can serve as a surrogate marker for CYP3A activity when evaluating drug pharmacokinetics. For instance, increased levels of 4α-HC were observed in patients treated with strong CYP3A inducers .
- Comparison with Other Biomarkers : Unlike other markers influenced by dietary or metabolic factors, 4α-HC concentrations remain stable under various conditions, making it a reliable indicator for CYP3A activity assessment.
In Vivo Studies
Recent studies have explored the effects of administering 4α-HC in animal models:
- Lipid Accumulation : In mouse models, administration of 4α-HC resulted in increased hepatic lipid content and triglyceride accumulation. This effect was linked to enhanced lipogenesis via LXR activation .
- Cell Culture Experiments : In vitro studies using primary hepatocytes demonstrated that treatment with 4α-HC led to increased lipid droplet formation and triglyceride synthesis, indicating its role as a pro-lipogenic factor .
Summary Table of Biological Activities
Biological Activity | Effect | Mechanism |
---|---|---|
LXR Activation | Promotes lipid synthesis | Direct binding to LXR |
Influence on SREBP | Potential modulation | Indirect through LXR |
CYP3A Activity Marker | Reflects drug interaction dynamics | Stable plasma concentrations |
Lipid Droplet Formation | Increases triglyceride accumulation | Enhanced lipogenesis |
Q & A
Basic Research Questions
Q. What methodological approaches are recommended for quantifying 4alpha-Hydroxy Cholesterol in biological samples?
- Answer : The quantification of this compound (4α-HC) requires precise analytical techniques due to its structural similarity to other oxysterols. Gas chromatography-mass spectrometry (GC-MS) is the gold standard for separating and identifying 4α-HC, leveraging its unique retention time and mass fragmentation patterns . Enzymatic assays, such as cholesterol oxidase-based methods, can be adapted for preliminary screening but require validation via LC-MS/MS to avoid cross-reactivity with other sterols . For tissue-specific analysis, solid-phase extraction (SPE) coupled with derivatization (e.g., silylation) enhances detection sensitivity in complex matrices like serum or liver homogenates .
Q. How is this compound synthesized and characterized in laboratory settings?
- Answer : 4α-HC synthesis typically involves enzymatic oxidation of cholesterol using cytochrome P450 enzymes (e.g., CYP8B1) or chemical methods like the use of oxidizing agents (e.g., tert-butyl hydroperoxide) under controlled conditions . Post-synthesis, characterization requires nuclear magnetic resonance (NMR) for structural confirmation (e.g., distinguishing 4α-HC from 4β-isomers) and high-resolution mass spectrometry (HRMS) for purity assessment . Researchers should cross-reference spectral data with established databases (e.g., SciFinder, Reaxys) to verify novelty or confirm identity against known compounds .
Q. What role does this compound play in cholesterol biosynthesis regulation?
- Answer : 4α-HC is a key intermediate in the alternative pathway of bile acid synthesis, where it modulates sterol 4alpha-methyl oxidase (SC4MOL) activity. Inhibition of SC4MOL by 4α-HC disrupts the conversion of lanosterol to cholesterol, leading to accumulation of methylsterols like 4,4'-dimethylzymosterol . This feedback mechanism is critical in studies investigating cholesterol-lowering agents, such as garlic-derived compounds (e.g., diallyl disulfide), which mimic 4α-HC’s inhibitory effects .
Advanced Research Questions
Q. How does this compound interact with sterol 4alpha-methyl oxidase (SC4MOL) to inhibit cholesterol synthesis?
- Answer : 4α-HC competitively binds to SC4MOL’s active site, blocking the oxidation of 4,4'-dimethylzymosterol—a critical step in cholesterol biosynthesis. This inhibition is validated via radiolabeled tracer studies (e.g., 14C-mevalonate) showing reduced cholesterol synthesis and accumulation of upstream intermediates like 4α-methylzymosterol . Knockout models of SC4MOL further confirm 4α-HC’s role, with hepatic lipidomics revealing elevated methylsterols and suppressed cholesterol levels .
Q. What transcriptional regulatory mechanisms govern enzymes involved in this compound metabolism?
- Answer : Hepatocyte nuclear factor 4alpha (HNF4α) regulates key enzymes in 4α-HC metabolism, including CYP8B1 (sterol 12α-hydroxylase) and CYP7A1 (cholesterol 7α-hydroxylase). Chromatin immunoprecipitation (ChIP) assays demonstrate HNF4α binding to the Cyp8b1 promoter, driving its circadian expression . Disruption of HNF4α in murine models reduces bile acid synthesis and alters 4α-HC homeostasis, highlighting its role in transcriptional regulation .
Q. How can contradictory data on this compound’s effects in experimental models be resolved?
- Answer : Contradictions often arise from differences in experimental design, such as:
- Temporal factors : 4α-HC levels fluctuate diurnally; studies sampling at inconsistent timepoints may yield conflicting results .
- Model systems : Cell culture models (e.g., hepatoma cells) may lack endogenous regulatory pathways present in vivo .
- Analytical specificity : Cross-reactivity in enzymatic assays vs. GC-MS specificity .
Resolution requires standardized protocols (e.g., synchronized sampling, genetic background controls) and multi-omics validation (e.g., combining lipidomics with transcriptomics) .
Q. Methodological Recommendations
- For pathway analysis : Use stable isotope labeling and tandem mass spectrometry to trace 4α-HC flux in bile acid vs. cholesterol pathways .
- For genetic studies : Employ CRISPR/Cas9 to knockout HNF4α or SC4MOL in hepatocyte models to isolate 4α-HC’s regulatory effects .
- For clinical correlations : Cross-reference 4α-HC levels with LDL-C/HDL-C ratios in hypercholesterolemia cohorts to assess its biomarker potential .
Eigenschaften
IUPAC Name |
(3S,4S,8S,9S,10R,13R,14S,17R)-10,13-dimethyl-17-[(2R)-6-methylheptan-2-yl]-2,3,4,7,8,9,11,12,14,15,16,17-dodecahydro-1H-cyclopenta[a]phenanthrene-3,4-diol | |
---|---|---|
Source | PubChem | |
URL | https://pubchem.ncbi.nlm.nih.gov | |
Description | Data deposited in or computed by PubChem | |
InChI |
InChI=1S/C27H46O2/c1-17(2)7-6-8-18(3)20-11-12-21-19-9-10-23-25(29)24(28)14-16-27(23,5)22(19)13-15-26(20,21)4/h10,17-22,24-25,28-29H,6-9,11-16H2,1-5H3/t18-,19+,20-,21+,22+,24+,25+,26-,27-/m1/s1 | |
Source | PubChem | |
URL | https://pubchem.ncbi.nlm.nih.gov | |
Description | Data deposited in or computed by PubChem | |
InChI Key |
CZDKQKOAHAICSF-GFYXKKFXSA-N | |
Source | PubChem | |
URL | https://pubchem.ncbi.nlm.nih.gov | |
Description | Data deposited in or computed by PubChem | |
Canonical SMILES |
CC(C)CCCC(C)C1CCC2C1(CCC3C2CC=C4C3(CCC(C4O)O)C)C | |
Source | PubChem | |
URL | https://pubchem.ncbi.nlm.nih.gov | |
Description | Data deposited in or computed by PubChem | |
Isomeric SMILES |
C[C@H](CCCC(C)C)[C@H]1CC[C@@H]2[C@@]1(CC[C@H]3[C@H]2CC=C4[C@@]3(CC[C@@H]([C@H]4O)O)C)C | |
Source | PubChem | |
URL | https://pubchem.ncbi.nlm.nih.gov | |
Description | Data deposited in or computed by PubChem | |
Molecular Formula |
C27H46O2 | |
Source | PubChem | |
URL | https://pubchem.ncbi.nlm.nih.gov | |
Description | Data deposited in or computed by PubChem | |
Molecular Weight |
402.7 g/mol | |
Source | PubChem | |
URL | https://pubchem.ncbi.nlm.nih.gov | |
Description | Data deposited in or computed by PubChem | |
Retrosynthesis Analysis
AI-Powered Synthesis Planning: Our tool employs the Template_relevance Pistachio, Template_relevance Bkms_metabolic, Template_relevance Pistachio_ringbreaker, Template_relevance Reaxys, Template_relevance Reaxys_biocatalysis model, leveraging a vast database of chemical reactions to predict feasible synthetic routes.
One-Step Synthesis Focus: Specifically designed for one-step synthesis, it provides concise and direct routes for your target compounds, streamlining the synthesis process.
Accurate Predictions: Utilizing the extensive PISTACHIO, BKMS_METABOLIC, PISTACHIO_RINGBREAKER, REAXYS, REAXYS_BIOCATALYSIS database, our tool offers high-accuracy predictions, reflecting the latest in chemical research and data.
Strategy Settings
Precursor scoring | Relevance Heuristic |
---|---|
Min. plausibility | 0.01 |
Model | Template_relevance |
Template Set | Pistachio/Bkms_metabolic/Pistachio_ringbreaker/Reaxys/Reaxys_biocatalysis |
Top-N result to add to graph | 6 |
Feasible Synthetic Routes
Haftungsausschluss und Informationen zu In-Vitro-Forschungsprodukten
Bitte beachten Sie, dass alle Artikel und Produktinformationen, die auf BenchChem präsentiert werden, ausschließlich zu Informationszwecken bestimmt sind. Die auf BenchChem zum Kauf angebotenen Produkte sind speziell für In-vitro-Studien konzipiert, die außerhalb lebender Organismen durchgeführt werden. In-vitro-Studien, abgeleitet von dem lateinischen Begriff "in Glas", beinhalten Experimente, die in kontrollierten Laborumgebungen unter Verwendung von Zellen oder Geweben durchgeführt werden. Es ist wichtig zu beachten, dass diese Produkte nicht als Arzneimittel oder Medikamente eingestuft sind und keine Zulassung der FDA für die Vorbeugung, Behandlung oder Heilung von medizinischen Zuständen, Beschwerden oder Krankheiten erhalten haben. Wir müssen betonen, dass jede Form der körperlichen Einführung dieser Produkte in Menschen oder Tiere gesetzlich strikt untersagt ist. Es ist unerlässlich, sich an diese Richtlinien zu halten, um die Einhaltung rechtlicher und ethischer Standards in Forschung und Experiment zu gewährleisten.