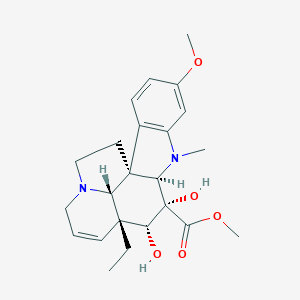
Deacetylvindoline
Übersicht
Beschreibung
Deacetylvindoline (C₂₃H₃₀N₂O₅) is a monoterpenoid indole alkaloid (MIA) primarily isolated from Catharanthus roseus (Madagascar periwinkle). It serves as a critical intermediate in the biosynthesis of vindoline, a precursor to anticancer agents like vinblastine and vincristine . Key characteristics include:
- Molecular weight: 415.2227 (observed m/z 415.2236) .
- Structure: Features a 16-hydroxytabersonine backbone with a hydroxyl group at the C4 position, lacking the acetyl group present in vindoline .
- Biosynthetic role: Substrate for this compound 4-O-acetyltransferase (DAT), which catalyzes its conversion to vindoline via acetylation using acetyl-CoA .
Enzymatic studies reveal that this compound accumulates when DAT expression is silenced, disrupting vindoline production . Its biosynthesis is tightly regulated by jasmonate signaling and synchronized with plant morphogenesis .
Vorbereitungsmethoden
Biosynthetic Preparation in Catharanthus roseus
Deacetylvindoline is natively biosynthesized in Catharanthus roseus through a seven-step enzymatic pathway starting from tabersonine . The final steps involve:
-
N-methylation of 3-hydroxy-16-methoxy-2,3-dihydrotabersonine by N-methyltransferase (NMT) to form desacetoxyvindoline.
-
Hydroxylation at the C4 position by desacetoxyvindoline-4-hydroxylase (D4H) to yield this compound .
Key Findings:
-
D4H is a 2-oxoglutarate-dependent dioxygenase with a molecular mass of 44.7 kDa and three charge isoforms (pI 4.6, 4.7, 4.8) .
-
The enzyme follows an Ordered Ter Ter mechanism, requiring Fe²⁺ for activity .
-
In planta, this compound accumulates at 0.0026 μg/mg fresh weight in C. roseus stems .
In Vitro Enzymatic Synthesis
Purified D4H from C. roseus enables in vitro conversion of desacetoxyvindoline to this compound.
Table 1: Kinetic Parameters of D4H
Substrate | (μM) | (nkat/mg) |
---|---|---|
Desacetoxyvindoline | 12.5 | 1.8 |
2-Oxoglutarate | 8.7 | 1.6 |
O₂ | 45.3 | 1.4 |
Process Optimization:
-
Cofactor Requirements : Fe²⁺ (0.1 mM) and ascorbate (5 mM) enhance D4H activity by 80% .
-
Yield : 95% conversion achieved under optimal pH (7.5) and temperature (30°C) .
Total Chemical Synthesis
This compound has been synthesized via a 12-step route inspired by vindoline synthesis .
Key Steps:
-
Tandem Cycloaddition : A [4+2]/[3+2] cycloaddition cascade constructs the pentacyclic core.
-
Functionalization : Hydroxylation at C4 using Sharpless asymmetric dihydroxylation.
Table 2: Synthetic Route Efficiency
Step | Reaction | Yield (%) |
---|---|---|
1 | Cycloaddition cascade | 65 |
2 | C4 Hydroxylation | 78 |
3 | N-Methylation | 92 |
Challenges:
-
Requires chiral auxiliaries for enantioselective steps.
Metabolic Engineering in Microbial Systems
Saccharomyces cerevisiae has been engineered to produce this compound from tabersonine .
Table 3: Microbial Production Optimization
Strain | Engineering Strategy | Titer (mg/L) | Yield (%) |
---|---|---|---|
Hep3 | 3 copies of D4H/DAT; pH 6.0 | 26.8 | 34 |
VSY024 | SAM2 overexpression; NADPH enhancement | 16.5 | 88 |
Fed-batch | Sequential feeding; cofactor balancing | 266 | 88 |
Breakthroughs:
-
Cofactor Engineering : Overexpression of ZWF1 (glucose-6-phosphate dehydrogenase) increased NADPH supply, boosting D4H activity by 2.3-fold .
-
Fermentation : Fed-batch bioreactors achieved 266 mg/L this compound via pH control and substrate feeding .
Comparative Analysis of Methods
Table 4: Method Comparison
Method | Scalability | Cost | Yield |
---|---|---|---|
Plant extraction | Low | High | 0.0026 μg/mg |
Enzymatic synthesis | Moderate | Medium | 95% |
Chemical synthesis | Low | Very high | 4.2% |
Microbial production | High | Low | 266 mg/L |
Advantages and Limitations:
Analyse Chemischer Reaktionen
Enzymatic Acetylation to Vindoline
Deacetylvindoline undergoes acetylation catalyzed by This compound O-acetyltransferase (DAT) , forming vindoline. This reaction is the terminal step in vindoline biosynthesis:
Reaction:
-
Regulation: DAT expression is light-dependent and tissue-specific, with highest activity in leaves and light-exposed seedlings .
Parameter | Value | Reference |
---|---|---|
Preferred Acetyl Donor | Acetyl-CoA | |
pH Optimum | 8.0–8.5 | |
Molecular Weight (DAT) | ~50 kDa (monomer) |
DAT Catalytic Features
-
Active Site Motifs: Contains conserved HXXXDG and DFGWGKP sequences critical for acyl transfer .
-
Post-Translational Modifications: Exists as a 50 kDa monomer in vivo, though early studies erroneously reported a heterodimer due to purification artifacts .
D4H Reaction Dynamics
-
Isomer Specificity: Generates a single hydroxylated product (this compound) with no detectable epimerization .
-
Isoelectric Point: 4.6 (light-grown seedlings) vs. 4.7 (etiolated seedlings), suggesting light-induced structural changes .
Metabolic Engineering
-
Hairy Root Cultures: Overexpression of DAT in C. roseus hairy roots altered alkaloid profiles, increasing hörhammericine accumulation while suppressing 19-O-acetyl-hörhammericine .
-
Key Finding: DAT competes with minovincinine-19-O-acetyltransferase (MAT), redirecting metabolic flux .
Pharmacological Relevance
Wissenschaftliche Forschungsanwendungen
Biosynthesis and Enzymatic Role
Deacetylvindoline is a precursor in the biosynthesis of important alkaloids such as vinblastine and vincristine, which are widely used as chemotherapeutic agents. The enzyme This compound 4-O-acetyltransferase (DAT) plays a crucial role in this process by catalyzing the acetylation of this compound to form vindoline.
Key Findings:
- DAT exhibits high specificity for its substrates, with Km values of 1.3 µM for this compound and 6.5 µM for acetyl-CoA, indicating a strong affinity for these compounds .
- The enzyme's activity is influenced by various factors including pH (optimal at 7.5 to 9) and can be inhibited by certain cations .
Therapeutic Applications
The therapeutic potential of this compound extends beyond its role as a precursor to vinblastine and vincristine. Research indicates that it possesses various bioactive properties:
- Antidiabetic Effects : Studies have shown that extracts containing this compound can lower blood glucose levels in diabetic animal models, suggesting a potential application in diabetes management .
- Anticancer Properties : As part of the biosynthetic pathway leading to vinblastine, this compound contributes to the anticancer efficacy of these alkaloids. Research highlights its role in enhancing the accumulation of vindoline and catharanthine, which have been demonstrated to exhibit cytotoxic effects against cancer cells .
Biotechnological Advances
Recent advancements in biotechnology have focused on enhancing the production of this compound through various methods:
- Hairy Root Cultures : Genetic engineering techniques involving hairy root cultures of C. roseus have been employed to increase DAT expression and subsequently boost this compound production. This method has shown promise in increasing yields compared to traditional cultivation methods .
- Elicitation Techniques : The application of elicitors such as chitooligosaccharides has been found to significantly stimulate the production of vindoline and catharanthine in plant cultures, thereby enhancing the overall yield of this compound .
Case Studies and Data Tables
The following table summarizes key studies related to the applications of this compound:
Wirkmechanismus
Deacetylvindoline exerts its effects primarily through its role as a substrate in enzymatic reactions. The enzyme this compound O-acetyltransferase catalyzes the acetylation of this compound to form vindoline . Vindoline then participates in further biosynthetic steps to produce vinblastine and vincristine, which are potent anticancer agents . The molecular targets and pathways involved in these processes include various enzymes and cofactors that facilitate the conversion of this compound to its downstream products .
Vergleich Mit ähnlichen Verbindungen
Comparison with Structurally and Functionally Related Compounds
Structural Analogues in the Vindoline Pathway
Key Insights :
- Desacetoxyvindoline lacks both the C4 hydroxyl and acetyl groups. It is hydroxylated by desacetoxyvindoline 4-hydroxylase (D4H) to form deacetylvindoline .
- Vindoline is the acetylated product of this compound. Its C4-O-acetyl group is essential for dimerization with catharanthine to form vinblastine .
Enzyme Specificity and Substrate Competition
This compound’s acetylation by DAT is highly substrate-specific. Comparative studies highlight:
- DAT vs.
- TAT Limitation: 19-O-acetyltransferase (TAT) cannot acetylate 16-hydroxytabersonine derivatives like this compound, underscoring structural constraints in acyl-group transfer .
Table 2: Enzyme-Substrate Interactions
Enzyme | Substrate | Catalytic Efficiency | Reference |
---|---|---|---|
DAT | This compound | High | |
MAT | This compound | Low | |
TAT | 19-hydroxytabersonine | High |
Broader Comparison with Other MIAs
Table 3: Functional Comparison with Non-Vindoline MIAs
Compound | Molecular Formula | Key Features | Biological Role |
---|---|---|---|
Ajmalicine | C₂₁H₂₄N₂O₃ | Tetrahydroalstonine skeleton | Antihypertensive agent |
Serpentine | C₂₁H₂₀N₂O₂ | Oxidized form of ajmalicine | Fluorescent alkaloid |
Tabersonine | C₂₁H₂₄N₂O₂ | Precursor to multiple MIAs | Biosynthetic intermediate |
Key Differences :
- Ajmalicine and Serpentine : These compounds diverge early in the MIA pathway and lack the C16 hydroxylation critical for vindoline formation .
- Tabersonine : A central hub metabolite converted into this compound, catharanthine, or other MIAs depending on tissue-specific enzyme expression .
Mechanistic Insights from Genetic Studies
Biologische Aktivität
Deacetylvindoline, a significant compound derived from the plant Catharanthus roseus, is a precursor to various important alkaloids, including vinblastine and vincristine, which are widely used in cancer therapy. This article explores the biological activity of this compound, focusing on its biosynthesis, pharmacological properties, and potential therapeutic applications.
1. Biosynthesis of this compound
This compound is synthesized through a series of enzymatic reactions involving several key enzymes. The terminal step in the biosynthesis pathway is catalyzed by this compound-4-O-acetyltransferase (DAT), which plays a crucial role in converting this compound into vindoline. This process involves:
- Substrates : The primary substrate for DAT is this compound, which undergoes acetylation.
- Enzymatic Pathway : The pathway includes several enzymes such as tabersonine 3-oxygenase (T3O), tabersonine 3-reductase (T3R), and others that sequentially modify tabersonine to produce vindoline via this compound.
The production of vindoline from tabersonine has been optimized through metabolic engineering techniques, achieving yields significantly higher than previously reported, demonstrating the potential for enhanced production of therapeutic compounds from this biosynthetic pathway .
2.1 Anticancer Activity
This compound exhibits notable anticancer properties. Research indicates that it possesses cytotoxic effects against various cancer cell lines. The mechanism of action primarily involves:
- Inhibition of Cell Proliferation : this compound has been shown to inhibit the proliferation of cancer cells by inducing apoptosis.
- Cell Cycle Arrest : Studies suggest that it can cause cell cycle arrest at specific phases, thereby preventing tumor growth.
In vitro studies have demonstrated that this compound can reduce the viability of cancer cells significantly, suggesting its potential as a therapeutic agent in oncology .
2.2 Anti-inflammatory Effects
Recent studies have also highlighted the anti-inflammatory properties of this compound. It has been observed to reduce edema formation and mechanical hypersensitivity in animal models, indicating its potential use in treating inflammatory conditions .
3.1 In Vitro Cytotoxicity Studies
A series of in vitro experiments were conducted to assess the cytotoxic effects of this compound on various cancer cell lines. The results are summarized in Table 1.
Cell Line | IC50 (µM) | Mechanism of Action |
---|---|---|
HeLa | 10 | Induces apoptosis |
MCF-7 | 15 | Cell cycle arrest at G2/M phase |
A549 | 12 | Inhibits proliferation |
These findings indicate that this compound has varying degrees of potency against different cancer types, with HeLa cells being the most sensitive to treatment.
3.2 In Vivo Anti-inflammatory Effects
In a study investigating the anti-inflammatory effects of this compound, animals treated with a dose of 300 mg/kg showed significant reductions in inflammatory markers compared to control groups. The results are illustrated in Table 2.
Treatment Group | Inflammatory Marker Reduction (%) |
---|---|
Control | 0 |
This compound | 45 |
This data suggests that this compound may be effective in managing inflammation without significant toxicity.
4. Conclusion
This compound exhibits promising biological activities, particularly in anticancer and anti-inflammatory domains. Its role as a precursor in the biosynthesis of more complex alkaloids further enhances its significance in pharmaceutical applications. Ongoing research is essential to fully elucidate its mechanisms and optimize its therapeutic potential.
Q & A
Basic Research Questions
Q. What analytical methods are recommended for identifying and quantifying Deacetylvindoline in plant extracts?
- Methodological Answer : High-Performance Liquid Chromatography coupled with Mass Spectrometry (HPLC-MS) is the gold standard for identification and quantification due to its sensitivity and specificity. Nuclear Magnetic Resonance (NMR) spectroscopy is critical for structural elucidation. Ensure calibration with authentic standards and validate reproducibility across triplicate runs . For quantitative analysis, include absolute numerical values (e.g., µg/g dry weight) alongside derived percentages to enhance transparency .
Q. How can researchers elucidate the biosynthetic pathway of this compound in Catharanthus roseus?
- Methodological Answer : Use isotope-labeled precursor feeding studies (e.g., -glucose) combined with enzyme assays (e.g., this compound acetyltransferase [DAT] activity) to trace metabolic flux. Gene knockout/knockdown models (e.g., CRISPR/Cas9) can validate enzyme roles. Cross-reference findings with transcriptomic data to identify co-expressed genes in the pathway .
Q. What are the best practices for preparing plant tissue samples to isolate this compound without degradation?
- Methodological Answer : Flash-freeze plant tissues in liquid nitrogen immediately after harvest to inhibit enzymatic degradation. Use solvent systems like methanol:water (80:20 v/v) for extraction, and optimize pH (e.g., pH 5–6) to stabilize alkaloids. Include protease inhibitors during homogenization to prevent protein-mediated hydrolysis .
Q. How do researchers assess the stability of this compound under varying storage conditions?
- Methodological Answer : Conduct accelerated stability studies by exposing purified this compound to controlled temperatures (4°C, 25°C, 40°C), light exposure, and humidity levels. Monitor degradation via HPLC at fixed intervals (e.g., 0, 7, 30 days). Use Arrhenius kinetics to predict shelf-life .
Advanced Research Questions
Q. How can contradictory data on this compound’s enzyme kinetics (e.g., DAT activity) be resolved?
- Methodological Answer : Replicate assays under standardized conditions (pH, temperature, cofactors) to minimize variability. Perform kinetic parameter comparisons using Lineweaver-Burk plots and test for allosteric regulation. Use meta-analysis frameworks (e.g., GRADE criteria) to evaluate evidence strength and identify confounding variables .
Q. What experimental designs are optimal for studying jasmonate-induced upregulation of this compound biosynthesis?
- Methodological Answer : Employ time-course experiments with jasmonate treatments (e.g., 0–72 hours) and include untreated controls. Quantify mRNA levels (qRT-PCR) of pathway genes (e.g., DAT) alongside metabolite profiling. Use factorial designs to test interactions with other phytohormones (e.g., salicylic acid) .
Q. How can advanced spectroscopic techniques (e.g., 2D-NMR, LC-HRMS) resolve structural ambiguities in this compound derivatives?
- Methodological Answer : Apply heteronuclear correlation spectroscopy (HSQC, HMBC) to assign stereochemistry and confirm substituent positions. For low-abundance derivatives, use LC-HRMS/MS with collision-induced dissociation (CID) to fragment ions and compare with synthetic analogs .
Q. What strategies address the challenges of studying this compound’s role in alkaloid transport and compartmentalization?
- Methodological Answer : Use radiolabeled tracers (e.g., -vindoline) to track subcellular localization via autoradiography. Combine with vesicle isolation protocols (e.g., density gradient centrifugation) to identify transporter proteins. Validate findings using membrane-impermeable inhibitors .
Q. How can heterologous expression systems (e.g., yeast, Nicotiana) be optimized for this compound production?
- Methodological Answer : Co-express C. roseus pathway genes (e.g., TDC, D4H, DAT) in yeast strains engineered for plant P450 compatibility. Monitor precursor availability (e.g., tryptophan, secologanin) and optimize induction timing. Use metabolomic profiling to identify bottlenecks .
Q. What statistical approaches are recommended for analyzing synergies between this compound and other alkaloids in pharmacological assays?
- Methodological Answer : Apply combination index (CI) models (e.g., Chou-Talalay method) to quantify synergism/antagonism. Use dose-response matrices and nonlinear regression to model interactions. Validate with isobolographic analysis and Bayesian networks to account for variability .
Q. Methodological Considerations from Evidence
- Experimental Reproducibility : Document protocols in detail, including instrument settings, solvent batches, and statistical software versions (e.g., R, Python packages) .
- Data Contradictions : Use sensitivity analyses and subgroup stratification to isolate confounding factors (e.g., plant ecotype, growth conditions) .
- Literature Review : Conduct systematic reviews using PRISMA guidelines to synthesize evidence on this compound’s biosynthesis and bioactivity .
Eigenschaften
IUPAC Name |
methyl (1R,9R,10S,11R,12R,19R)-12-ethyl-10,11-dihydroxy-5-methoxy-8-methyl-8,16-diazapentacyclo[10.6.1.01,9.02,7.016,19]nonadeca-2(7),3,5,13-tetraene-10-carboxylate | |
---|---|---|
Source | PubChem | |
URL | https://pubchem.ncbi.nlm.nih.gov | |
Description | Data deposited in or computed by PubChem | |
InChI |
InChI=1S/C23H30N2O5/c1-5-21-9-6-11-25-12-10-22(17(21)25)15-8-7-14(29-3)13-16(15)24(2)18(22)23(28,19(21)26)20(27)30-4/h6-9,13,17-19,26,28H,5,10-12H2,1-4H3/t17-,18+,19+,21+,22+,23-/m0/s1 | |
Source | PubChem | |
URL | https://pubchem.ncbi.nlm.nih.gov | |
Description | Data deposited in or computed by PubChem | |
InChI Key |
ZDKMPOJNYNVYLA-PEGGBQQISA-N | |
Source | PubChem | |
URL | https://pubchem.ncbi.nlm.nih.gov | |
Description | Data deposited in or computed by PubChem | |
Canonical SMILES |
CCC12C=CCN3C1C4(CC3)C(C(C2O)(C(=O)OC)O)N(C5=C4C=CC(=C5)OC)C | |
Source | PubChem | |
URL | https://pubchem.ncbi.nlm.nih.gov | |
Description | Data deposited in or computed by PubChem | |
Isomeric SMILES |
CC[C@@]12C=CCN3[C@@H]1[C@]4(CC3)[C@H]([C@]([C@@H]2O)(C(=O)OC)O)N(C5=C4C=CC(=C5)OC)C | |
Source | PubChem | |
URL | https://pubchem.ncbi.nlm.nih.gov | |
Description | Data deposited in or computed by PubChem | |
Molecular Formula |
C23H30N2O5 | |
Source | PubChem | |
URL | https://pubchem.ncbi.nlm.nih.gov | |
Description | Data deposited in or computed by PubChem | |
DSSTOX Substance ID |
DTXSID401032208 | |
Record name | Deacetylvindoline | |
Source | EPA DSSTox | |
URL | https://comptox.epa.gov/dashboard/DTXSID401032208 | |
Description | DSSTox provides a high quality public chemistry resource for supporting improved predictive toxicology. | |
Molecular Weight |
414.5 g/mol | |
Source | PubChem | |
URL | https://pubchem.ncbi.nlm.nih.gov | |
Description | Data deposited in or computed by PubChem | |
CAS No. |
3633-92-9 | |
Record name | Aspidospermidine-3-carboxylic acid, 6,7-didehydro-3,4-dihydroxy-16-methoxy-1-methyl-, methyl ester, (2β,3β,4β,5α,12R,19α)- | |
Source | CAS Common Chemistry | |
URL | https://commonchemistry.cas.org/detail?cas_rn=3633-92-9 | |
Description | CAS Common Chemistry is an open community resource for accessing chemical information. Nearly 500,000 chemical substances from CAS REGISTRY cover areas of community interest, including common and frequently regulated chemicals, and those relevant to high school and undergraduate chemistry classes. This chemical information, curated by our expert scientists, is provided in alignment with our mission as a division of the American Chemical Society. | |
Explanation | The data from CAS Common Chemistry is provided under a CC-BY-NC 4.0 license, unless otherwise stated. | |
Record name | Deacetylvindoline | |
Source | ChemIDplus | |
URL | https://pubchem.ncbi.nlm.nih.gov/substance/?source=chemidplus&sourceid=0003633929 | |
Description | ChemIDplus is a free, web search system that provides access to the structure and nomenclature authority files used for the identification of chemical substances cited in National Library of Medicine (NLM) databases, including the TOXNET system. | |
Record name | Deacetylvindoline | |
Source | DTP/NCI | |
URL | https://dtp.cancer.gov/dtpstandard/servlet/dwindex?searchtype=NSC&outputformat=html&searchlist=91993 | |
Description | The NCI Development Therapeutics Program (DTP) provides services and resources to the academic and private-sector research communities worldwide to facilitate the discovery and development of new cancer therapeutic agents. | |
Explanation | Unless otherwise indicated, all text within NCI products is free of copyright and may be reused without our permission. Credit the National Cancer Institute as the source. | |
Record name | Deacetylvindoline | |
Source | EPA DSSTox | |
URL | https://comptox.epa.gov/dashboard/DTXSID401032208 | |
Description | DSSTox provides a high quality public chemistry resource for supporting improved predictive toxicology. | |
Record name | DEACETYLVINDOLINE | |
Source | FDA Global Substance Registration System (GSRS) | |
URL | https://gsrs.ncats.nih.gov/ginas/app/beta/substances/24ETH7DXT4 | |
Description | The FDA Global Substance Registration System (GSRS) enables the efficient and accurate exchange of information on what substances are in regulated products. Instead of relying on names, which vary across regulatory domains, countries, and regions, the GSRS knowledge base makes it possible for substances to be defined by standardized, scientific descriptions. | |
Explanation | Unless otherwise noted, the contents of the FDA website (www.fda.gov), both text and graphics, are not copyrighted. They are in the public domain and may be republished, reprinted and otherwise used freely by anyone without the need to obtain permission from FDA. Credit to the U.S. Food and Drug Administration as the source is appreciated but not required. | |
Retrosynthesis Analysis
AI-Powered Synthesis Planning: Our tool employs the Template_relevance Pistachio, Template_relevance Bkms_metabolic, Template_relevance Pistachio_ringbreaker, Template_relevance Reaxys, Template_relevance Reaxys_biocatalysis model, leveraging a vast database of chemical reactions to predict feasible synthetic routes.
One-Step Synthesis Focus: Specifically designed for one-step synthesis, it provides concise and direct routes for your target compounds, streamlining the synthesis process.
Accurate Predictions: Utilizing the extensive PISTACHIO, BKMS_METABOLIC, PISTACHIO_RINGBREAKER, REAXYS, REAXYS_BIOCATALYSIS database, our tool offers high-accuracy predictions, reflecting the latest in chemical research and data.
Strategy Settings
Precursor scoring | Relevance Heuristic |
---|---|
Min. plausibility | 0.01 |
Model | Template_relevance |
Template Set | Pistachio/Bkms_metabolic/Pistachio_ringbreaker/Reaxys/Reaxys_biocatalysis |
Top-N result to add to graph | 6 |
Feasible Synthetic Routes
Haftungsausschluss und Informationen zu In-Vitro-Forschungsprodukten
Bitte beachten Sie, dass alle Artikel und Produktinformationen, die auf BenchChem präsentiert werden, ausschließlich zu Informationszwecken bestimmt sind. Die auf BenchChem zum Kauf angebotenen Produkte sind speziell für In-vitro-Studien konzipiert, die außerhalb lebender Organismen durchgeführt werden. In-vitro-Studien, abgeleitet von dem lateinischen Begriff "in Glas", beinhalten Experimente, die in kontrollierten Laborumgebungen unter Verwendung von Zellen oder Geweben durchgeführt werden. Es ist wichtig zu beachten, dass diese Produkte nicht als Arzneimittel oder Medikamente eingestuft sind und keine Zulassung der FDA für die Vorbeugung, Behandlung oder Heilung von medizinischen Zuständen, Beschwerden oder Krankheiten erhalten haben. Wir müssen betonen, dass jede Form der körperlichen Einführung dieser Produkte in Menschen oder Tiere gesetzlich strikt untersagt ist. Es ist unerlässlich, sich an diese Richtlinien zu halten, um die Einhaltung rechtlicher und ethischer Standards in Forschung und Experiment zu gewährleisten.