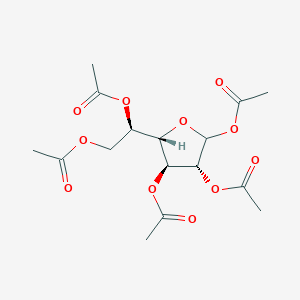
D-Galactofuranose Pentaacetate
Übersicht
Beschreibung
D-Galactofuranose Pentaacetate is a derivative of D-galactofuranose, a five-membered ring form of the sugar galactose. This compound is characterized by the presence of five acetyl groups attached to the hydroxyl groups of the galactofuranose molecule. It is widely used in biochemical research, particularly in the study of glycosylation processes and the synthesis of complex carbohydrates .
Wissenschaftliche Forschungsanwendungen
D-Galactofuranose Pentaacetate has several applications in scientific research:
Chemistry: Used as a building block in the synthesis of complex carbohydrates and glycoconjugates.
Medicine: Investigated for its potential in developing therapeutic agents targeting glycosylation pathways in pathogens.
Industry: Utilized in the production of specialized chemicals and as a reagent in various biochemical assays.
Safety and Hazards
Zukünftige Richtungen
Galactofuranose, the atypical and thermodynamically disfavored form of D-galactose, has been found in numerous pathogenic micro-organisms, making the enzymes responsible for its biosynthesis interesting targets . Since galactofuranose has never been found in mammals, galactofuranose-biosynthetic pathways have raised much interest as targets for drug development to combat microbial infections .
Wirkmechanismus
D-Galactofuranose Pentaacetate, also known as 1,2,3,5,6-PENTA-O-ACETYL-D-GALACTOFURANOSE, is a fascinating compound with a rich history in chemistry and biochemistry . This compound has been synthesized from D-galactose and has been used in various biomedical research applications .
Target of Action
It’s known that galactofuranose, the non-acetylated form of this compound, plays a crucial role in the biosynthesis of certain biological molecules .
Mode of Action
It’s known that the compound is a condensation product of a 4-methylumbelliferone with the anomeric form of d-galactose . This suggests that it may interact with its targets through a similar mechanism.
Biochemical Pathways
It’s known that galactofuranose, the non-acetylated form of this compound, is involved in the biosynthesis of certain biological molecules .
Pharmacokinetics
Given its molecular weight of 39034 , it’s likely that it has good bioavailability.
Result of Action
It’s known that the compound has immense potential in the field of biomedical research .
Biochemische Analyse
Biochemical Properties
D-Galactofuranose Pentaacetate plays a role in biochemical reactions, particularly in the context of enzymatic activity. A novel Galactofuranose-Specific β-D-Galactofuranosidase from Streptomyces species has been identified that exhibits activity for 4-nitrophenyl β-D-galactofuranoside . This enzyme was able to release galactose residue from galactomannan prepared from the filamentous fungus Aspergillus fumigatus , suggesting that this compound could be a substrate for this enzyme.
Cellular Effects
This compound may have effects on various types of cells and cellular processes. Galactofuranose, the parent compound, is a component of polysaccharides and glycoconjugates present on the surface of the cell wall in many pathogenic bacteria and eukaryotes . The absence of galactofuranose often results in morphological abnormalities and an impaired cell wall function in these organisms .
Molecular Mechanism
It is known that the enzyme β-D-galactofuranosidase can release galactose residue from substrates such as galactomannan . This suggests that this compound may interact with this enzyme and potentially others in a similar manner.
Temporal Effects in Laboratory Settings
The first endo-β-d-Galf-ase, which can interact with galactofuranose, was found to have specific stability properties and kinetic characteristics .
Metabolic Pathways
This compound may be involved in the metabolic pathways of galactofuranose. Galactofuranose is a component of polysaccharides and glycoconjugates, and its transferase has been well analyzed .
Vorbereitungsmethoden
Synthetic Routes and Reaction Conditions: The synthesis of D-Galactofuranose Pentaacetate typically involves the peracetylation of D-galactose. The process begins with the dissolution of D-galactose in boiling pyridine, followed by the addition of acetic anhydride. The reaction mixture is then heated to facilitate the acetylation process. After completion, the product is purified through fractional crystallization and recrystallized from ethanol to obtain pure this compound .
Industrial Production Methods: While specific industrial production methods for this compound are not well-documented, the general approach involves large-scale acetylation reactions under controlled conditions to ensure high yield and purity. The use of automated reactors and advanced purification techniques can enhance the efficiency of the production process.
Analyse Chemischer Reaktionen
Types of Reactions: D-Galactofuranose Pentaacetate undergoes various chemical reactions, including:
Oxidation: The acetyl groups can be oxidized under specific conditions to form corresponding carboxylic acids.
Reduction: Reduction reactions can remove the acetyl groups, reverting the compound back to D-galactofuranose.
Substitution: The acetyl groups can be substituted with other functional groups through nucleophilic substitution reactions.
Common Reagents and Conditions:
Oxidation: Common oxidizing agents include potassium permanganate and chromium trioxide.
Reduction: Reducing agents such as lithium aluminum hydride or sodium borohydride are used.
Substitution: Nucleophiles like hydroxylamine or hydrazine can be employed for substitution reactions.
Major Products:
Oxidation: Formation of galactofuranuronic acid derivatives.
Reduction: Regeneration of D-galactofuranose.
Substitution: Formation of various substituted galactofuranose derivatives.
Vergleich Mit ähnlichen Verbindungen
D-Glucose Pentaacetate: Similar in structure but derived from glucose instead of galactose.
D-Mannose Pentaacetate: Another similar compound derived from mannose.
D-Xylose Pentaacetate: Derived from xylose, differing in the sugar backbone.
Uniqueness: D-Galactofuranose Pentaacetate is unique due to its five-membered ring structure and its specific role in the glycosylation processes of certain pathogenic microorganisms. Unlike its pyranose counterparts, the furanose form is less common and has distinct biochemical properties that make it valuable in research and industrial applications .
Eigenschaften
IUPAC Name |
[(2R)-2-acetyloxy-2-[(2S,3S,4R)-3,4,5-triacetyloxyoxolan-2-yl]ethyl] acetate | |
---|---|---|
Source | PubChem | |
URL | https://pubchem.ncbi.nlm.nih.gov | |
Description | Data deposited in or computed by PubChem | |
InChI |
InChI=1S/C16H22O11/c1-7(17)22-6-12(23-8(2)18)13-14(24-9(3)19)15(25-10(4)20)16(27-13)26-11(5)21/h12-16H,6H2,1-5H3/t12-,13+,14+,15-,16?/m1/s1 | |
Source | PubChem | |
URL | https://pubchem.ncbi.nlm.nih.gov | |
Description | Data deposited in or computed by PubChem | |
InChI Key |
JRZQXPYZEBBLPJ-RRMRAIHUSA-N | |
Source | PubChem | |
URL | https://pubchem.ncbi.nlm.nih.gov | |
Description | Data deposited in or computed by PubChem | |
Canonical SMILES |
CC(=O)OCC(C1C(C(C(O1)OC(=O)C)OC(=O)C)OC(=O)C)OC(=O)C | |
Source | PubChem | |
URL | https://pubchem.ncbi.nlm.nih.gov | |
Description | Data deposited in or computed by PubChem | |
Isomeric SMILES |
CC(=O)OC[C@H]([C@H]1[C@@H]([C@H](C(O1)OC(=O)C)OC(=O)C)OC(=O)C)OC(=O)C | |
Source | PubChem | |
URL | https://pubchem.ncbi.nlm.nih.gov | |
Description | Data deposited in or computed by PubChem | |
Molecular Formula |
C16H22O11 | |
Source | PubChem | |
URL | https://pubchem.ncbi.nlm.nih.gov | |
Description | Data deposited in or computed by PubChem | |
Molecular Weight |
390.34 g/mol | |
Source | PubChem | |
URL | https://pubchem.ncbi.nlm.nih.gov | |
Description | Data deposited in or computed by PubChem | |
Retrosynthesis Analysis
AI-Powered Synthesis Planning: Our tool employs the Template_relevance Pistachio, Template_relevance Bkms_metabolic, Template_relevance Pistachio_ringbreaker, Template_relevance Reaxys, Template_relevance Reaxys_biocatalysis model, leveraging a vast database of chemical reactions to predict feasible synthetic routes.
One-Step Synthesis Focus: Specifically designed for one-step synthesis, it provides concise and direct routes for your target compounds, streamlining the synthesis process.
Accurate Predictions: Utilizing the extensive PISTACHIO, BKMS_METABOLIC, PISTACHIO_RINGBREAKER, REAXYS, REAXYS_BIOCATALYSIS database, our tool offers high-accuracy predictions, reflecting the latest in chemical research and data.
Strategy Settings
Precursor scoring | Relevance Heuristic |
---|---|
Min. plausibility | 0.01 |
Model | Template_relevance |
Template Set | Pistachio/Bkms_metabolic/Pistachio_ringbreaker/Reaxys/Reaxys_biocatalysis |
Top-N result to add to graph | 6 |
Feasible Synthetic Routes
Q1: What is the significance of D-Galactofuranose Pentaacetate in the synthesis of 4-Nitrocatechol 1-yl α-D-Galactofuranoside?
A1: this compound serves as a crucial precursor in the multi-step synthesis of 4-Nitrocatechol 1-yl α-D-Galactofuranoside []. This target compound is important because it acts as a substrate for exo-galactofuranosidases, enzymes involved in the breakdown of galactofuranose-containing polysaccharides. Specifically, this compound undergoes bromination to yield tetra-O-acetyl α-D-galactofuranosyl bromide, which then reacts with 4-nitrocatechol to ultimately produce the desired substrate [].
Haftungsausschluss und Informationen zu In-Vitro-Forschungsprodukten
Bitte beachten Sie, dass alle Artikel und Produktinformationen, die auf BenchChem präsentiert werden, ausschließlich zu Informationszwecken bestimmt sind. Die auf BenchChem zum Kauf angebotenen Produkte sind speziell für In-vitro-Studien konzipiert, die außerhalb lebender Organismen durchgeführt werden. In-vitro-Studien, abgeleitet von dem lateinischen Begriff "in Glas", beinhalten Experimente, die in kontrollierten Laborumgebungen unter Verwendung von Zellen oder Geweben durchgeführt werden. Es ist wichtig zu beachten, dass diese Produkte nicht als Arzneimittel oder Medikamente eingestuft sind und keine Zulassung der FDA für die Vorbeugung, Behandlung oder Heilung von medizinischen Zuständen, Beschwerden oder Krankheiten erhalten haben. Wir müssen betonen, dass jede Form der körperlichen Einführung dieser Produkte in Menschen oder Tiere gesetzlich strikt untersagt ist. Es ist unerlässlich, sich an diese Richtlinien zu halten, um die Einhaltung rechtlicher und ethischer Standards in Forschung und Experiment zu gewährleisten.