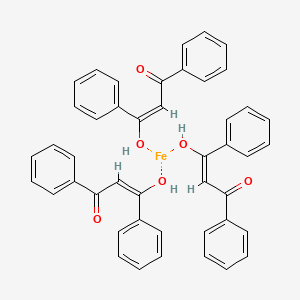
Tris(dibenzoylmethanato)iron
Übersicht
Beschreibung
Tris(dibenzoylmethanato)iron, also known as iron(III) tris(1,3-diphenyl-1,3-propanedionato), is an organometallic compound with the molecular formula C45H36FeO6. It is characterized by its deep yellowish-red to black crystalline appearance and is known for its stability and solubility in tetrahydrofuran (THF). This compound is widely used as a catalyst in various organic reactions due to its unique chemical properties .
Wirkmechanismus
Target of Action
Tris(dibenzoylmethanato)iron, with the empirical formula C45H36FeO6 , is primarily used as a catalyst in organic synthesis . Its primary targets are organic compounds, specifically alkenyl halides and Grignard reagents .
Mode of Action
The compound acts as a catalyst for the coupling of alkenyl halides with Grignard reagents . It also promotes the samarium (II) iodide intramolecular Barbier-type reaction for the synthesis of bicyclo [m.n.l]alkan-1-ols . The interaction with its targets results in the formation of new chemical bonds, facilitating the synthesis of complex organic compounds.
Biochemical Pathways
It is known to facilitate the synthesis of bicyclo [mnl]alkan-1-ols , which are involved in various biochemical reactions.
Result of Action
The primary result of this compound’s action is the facilitation of chemical reactions, leading to the synthesis of complex organic compounds . It enables the coupling of alkenyl halides with Grignard reagents and promotes the samarium (II) iodide intramolecular Barbier-type reaction .
Biochemische Analyse
Biochemical Properties
Tris(dibenzoylmethanato)iron plays a significant role in biochemical reactions, particularly as a catalyst. It interacts with various enzymes and proteins, facilitating reactions that are otherwise slow or inefficient. For instance, it has been used in the coupling of alkenyl halides with Grignard reagents and in the samarium(II) iodide promoted intramolecular Barbier-type reaction for the synthesis of bicyclo[m.n.l]alkan-1-ols . These interactions are primarily due to the compound’s ability to stabilize transition states and lower activation energies, thereby enhancing reaction rates.
Cellular Effects
This compound influences various cellular processes, including cell signaling pathways, gene expression, and cellular metabolism. It has been observed to affect the function of cells by modulating the activity of specific enzymes and proteins involved in these pathways. For example, it can alter the phosphorylation status of proteins, thereby impacting signal transduction and gene expression. Additionally, its interaction with metabolic enzymes can lead to changes in cellular metabolism, affecting the overall energy balance and function of the cell .
Molecular Mechanism
The molecular mechanism of action of this compound involves its binding interactions with biomolecules. It can act as an enzyme inhibitor or activator, depending on the specific enzyme and the context of the reaction. The compound’s ability to chelate metal ions plays a crucial role in its mechanism of action. By binding to metal ions, this compound can inhibit the activity of metalloenzymes or alter their substrate specificity. Additionally, it can influence gene expression by interacting with transcription factors or other regulatory proteins .
Temporal Effects in Laboratory Settings
In laboratory settings, the effects of this compound can change over time due to its stability and degradation properties. The compound is generally stable under standard laboratory conditions, but prolonged exposure to light or air can lead to its degradation. This degradation can affect its efficacy and the outcomes of biochemical reactions. Long-term studies have shown that the compound can have lasting effects on cellular function, particularly in in vitro and in vivo models, where it can influence cell growth and differentiation over extended periods .
Dosage Effects in Animal Models
The effects of this compound vary with different dosages in animal models. At low doses, the compound can enhance specific biochemical reactions without causing significant adverse effects. At higher doses, it can exhibit toxic effects, including oxidative stress and cellular damage. These threshold effects are crucial for determining the safe and effective use of the compound in research and potential therapeutic applications .
Metabolic Pathways
This compound is involved in various metabolic pathways, interacting with enzymes and cofactors essential for these processes. It can affect metabolic flux and metabolite levels by modulating the activity of key enzymes. For instance, its interaction with enzymes involved in the tricarboxylic acid cycle can influence the production of ATP and other critical metabolites, thereby impacting cellular energy metabolism .
Transport and Distribution
Within cells and tissues, this compound is transported and distributed through interactions with specific transporters and binding proteins. These interactions determine its localization and accumulation within different cellular compartments. The compound’s ability to bind to metal ions also plays a role in its transport and distribution, as it can be sequestered in organelles such as mitochondria or lysosomes .
Subcellular Localization
The subcellular localization of this compound is influenced by targeting signals and post-translational modifications that direct it to specific compartments or organelles. For example, it may be directed to the mitochondria, where it can influence mitochondrial function and energy production. Additionally, its localization within the nucleus can affect gene expression by interacting with transcription factors and other regulatory proteins .
Vorbereitungsmethoden
Synthetic Routes and Reaction Conditions: Tris(dibenzoylmethanato)iron can be synthesized through the reaction of iron(III) chloride with dibenzoylmethane in the presence of a base such as sodium hydroxide. The reaction typically occurs in an organic solvent like ethanol or methanol under reflux conditions. The product is then purified through recrystallization .
Industrial Production Methods: While specific industrial production methods are not extensively documented, the synthesis generally follows the laboratory preparation route with optimizations for scale-up. This includes using larger reaction vessels, continuous stirring, and controlled temperature conditions to ensure consistent product quality .
Analyse Chemischer Reaktionen
Types of Reactions: Tris(dibenzoylmethanato)iron undergoes various types of chemical reactions, including:
Oxidation: It can catalyze the oxidation of olefins to ketones at room temperature using air as the oxidant.
Reduction: It forms a redox couple with its iron(II) counterpart, facilitating reduction reactions.
Substitution: It participates in cross-coupling reactions with Grignard reagents, leading to the formation of new carbon-carbon bonds.
Common Reagents and Conditions:
Oxidation: Phenylsilane as an additive, air as the oxidant, room temperature conditions.
Reduction: Iron(II) chloride and neocuproine as catalysts.
Substitution: Alkenyl halides and Grignard reagents in the presence of THF.
Major Products:
Oxidation: Ketones and corresponding alcohols as by-products.
Reduction: Various reduced organic compounds depending on the substrates used.
Substitution: Coupled products with new carbon-carbon bonds.
Wissenschaftliche Forschungsanwendungen
Tris(dibenzoylmethanato)iron has a wide range of applications in scientific research:
Vergleich Mit ähnlichen Verbindungen
Iron(III) acetylacetonate (C15H21FeO6): Similar in structure but with acetylacetonate ligands instead of dibenzoylmethane.
Iron(III) chloride (FeCl3): A simpler iron compound used in various catalytic processes.
Iron(III) oxalate (Fe2(C2O4)3): Another iron compound with different ligand properties.
Uniqueness: Tris(dibenzoylmethanato)iron is unique due to its stability, solubility in THF, and its ability to catalyze a wide range of organic reactions under mild conditions. Its specific ligand environment provides distinct reactivity compared to other iron compounds, making it a valuable catalyst in both academic and industrial settings .
Eigenschaften
CAS-Nummer |
14405-49-3 |
---|---|
Molekularformel |
C45H33FeO6 |
Molekulargewicht |
725.6 g/mol |
IUPAC-Name |
iron(3+);3-oxo-1,3-diphenylprop-1-en-1-olate |
InChI |
InChI=1S/3C15H12O2.Fe/c3*16-14(12-7-3-1-4-8-12)11-15(17)13-9-5-2-6-10-13;/h3*1-11,16H;/q;;;+3/p-3 |
InChI-Schlüssel |
JWPHPBLJVLHKOZ-UHFFFAOYSA-K |
SMILES |
C1=CC=C(C=C1)C(=CC(=O)C2=CC=CC=C2)O.C1=CC=C(C=C1)C(=CC(=O)C2=CC=CC=C2)O.C1=CC=C(C=C1)C(=CC(=O)C2=CC=CC=C2)O.[Fe] |
Isomerische SMILES |
C1=CC=C(C=C1)/C(=C\C(=O)C2=CC=CC=C2)/O.C1=CC=C(C=C1)/C(=C\C(=O)C2=CC=CC=C2)/O.C1=CC=C(C=C1)/C(=C\C(=O)C2=CC=CC=C2)/O.[Fe] |
Kanonische SMILES |
C1=CC=C(C=C1)C(=CC(=O)C2=CC=CC=C2)[O-].C1=CC=C(C=C1)C(=CC(=O)C2=CC=CC=C2)[O-].C1=CC=C(C=C1)C(=CC(=O)C2=CC=CC=C2)[O-].[Fe+3] |
Herkunft des Produkts |
United States |
Retrosynthesis Analysis
AI-Powered Synthesis Planning: Our tool employs the Template_relevance Pistachio, Template_relevance Bkms_metabolic, Template_relevance Pistachio_ringbreaker, Template_relevance Reaxys, Template_relevance Reaxys_biocatalysis model, leveraging a vast database of chemical reactions to predict feasible synthetic routes.
One-Step Synthesis Focus: Specifically designed for one-step synthesis, it provides concise and direct routes for your target compounds, streamlining the synthesis process.
Accurate Predictions: Utilizing the extensive PISTACHIO, BKMS_METABOLIC, PISTACHIO_RINGBREAKER, REAXYS, REAXYS_BIOCATALYSIS database, our tool offers high-accuracy predictions, reflecting the latest in chemical research and data.
Strategy Settings
Precursor scoring | Relevance Heuristic |
---|---|
Min. plausibility | 0.01 |
Model | Template_relevance |
Template Set | Pistachio/Bkms_metabolic/Pistachio_ringbreaker/Reaxys/Reaxys_biocatalysis |
Top-N result to add to graph | 6 |
Feasible Synthetic Routes
Q1: What are the products of Tris(dibenzoylmethanato)iron(III) photodegradation in the presence of oxygen?
A1: Research on the photolysis of this compound(III) in benzene solution, under oxygen-rich conditions, reveals the formation of several products. The primary products include a basic ferric salt of diphenyltartaric acid, benzoic acid, and phenyl benzoate. Notably, phenylglyoxylic acid appears as an intermediate during this photodegradation process [].
Q2: Can this compound(III) be used as a catalyst for olefin oxidation? What are the advantages of this approach?
A2: Yes, this compound(III) [Fe(dbm)3] demonstrates efficacy as a catalyst for oxidizing olefins into ketones []. This method, often conducted at room temperature, utilizes air as the sole oxidizing agent and incorporates phenylsilane (PhSiH3) as an additive. The reaction displays high functional group tolerance and achieves impressive regioselectivity, yielding ketones with up to 97% yield. Researchers have observed alcohols as byproducts in this process. Key advantages of this catalytic approach include mild reaction conditions, operational simplicity, and broad substrate compatibility.
Q3: What is the role of phenylsilane in the this compound(III)-catalyzed olefin oxidation?
A3: Labeling experiments have provided insights into the role of phenylsilane in this catalytic reaction. Findings indicate that a hydrogen atom from phenylsilane gets incorporated during the oxidation process. Furthermore, the oxygen atom found in both the resultant ketone and alcohol originates from the surrounding atmosphere []. This suggests that phenylsilane acts as a hydrogen source and potentially plays a role in activating the catalyst or facilitating oxygen transfer.
Haftungsausschluss und Informationen zu In-Vitro-Forschungsprodukten
Bitte beachten Sie, dass alle Artikel und Produktinformationen, die auf BenchChem präsentiert werden, ausschließlich zu Informationszwecken bestimmt sind. Die auf BenchChem zum Kauf angebotenen Produkte sind speziell für In-vitro-Studien konzipiert, die außerhalb lebender Organismen durchgeführt werden. In-vitro-Studien, abgeleitet von dem lateinischen Begriff "in Glas", beinhalten Experimente, die in kontrollierten Laborumgebungen unter Verwendung von Zellen oder Geweben durchgeführt werden. Es ist wichtig zu beachten, dass diese Produkte nicht als Arzneimittel oder Medikamente eingestuft sind und keine Zulassung der FDA für die Vorbeugung, Behandlung oder Heilung von medizinischen Zuständen, Beschwerden oder Krankheiten erhalten haben. Wir müssen betonen, dass jede Form der körperlichen Einführung dieser Produkte in Menschen oder Tiere gesetzlich strikt untersagt ist. Es ist unerlässlich, sich an diese Richtlinien zu halten, um die Einhaltung rechtlicher und ethischer Standards in Forschung und Experiment zu gewährleisten.